The right ventricle plays a crucial role in determining patient functional status and prognosis in various conditions. Accordingly, the quantification of right ventricular (RV) function should be part of any routine echocardiographic workup. However, the assessment of RV size and function remains one of the most challenging and technically difficult tasks in echocardiography. There are many anatomic and functional peculiarities that distinguish the right ventricle from the left ventricle, which pose significant challenges to the conventional echocardiographic assessment of RV function and preclude the extrapolation of the knowledge and evidence accumulated for the left side to the right ventricle.
In recent years, RV strain imaging emerged as a superior metric of RV systolic performance, overcoming some of the limitations of conventional echocardiographic parameters ( Fig. 9.1 ). RV longitudinal strain (RVLS) can be measured by both Doppler tissue imaging and speckle-tracking echocardiography techniques and has been validated in experimental animal studies with sonomicrometry and with cardiac magnetic resonance in several cardiac conditions. The advantages of using the speckle tracking over the Doppler tissue imaging technique rely on the independence of the measurements from the angle of insonation and better reproducibility. In general, RV pathology can be divided into pressure loaded and volume loaded and myopathic patterns ( Fig. 9.2 ), which have different strain curves.



The purposes of this chapter are to familiarize readers with the anatomy of the right ventricle and the advantages of RV strain analysis with respect to conventional echocardiographic parameters and to provide an overview of the current knowledge and clinical applications of RV strain imaging in various pathologic conditions.
Functional anatomy and myocardial fiber arrangement of the right ventricle
The right ventricle resembles a triangle when viewed in frontal section and a crescent when viewed in cross section because of the concave position of the free wall and convex shape of the interventricular septum toward the right ventricle in both systole and diastole under normal loading conditions. Compared with the left ventricle, the right ventricle has a 10% to 15% larger end-diastolic volume and one-third to one-sixth smaller mass. Because the right ventricle normally operates at a lower pressure than the left ventricle, the normal RV wall is thinner (3–5 mm in adults) and more compliant than the left ventricular (LV) wall. Thus, for a comparable pressure increase, the right ventricle dilates more than the left ventricle because of higher wall stress (larger radius and smaller wall thickness). Unlike LV coronary blood flow primarily occurring in diastole, the RV coronary blood flow occurs during both systole and diastole. The right ventricle has a smaller oxygen demand than the left ventricle and is less vulnerable to ischemia.
Anatomically, the right ventricle is composed of three portions: the inlet, the trabeculated apical myocardium, and the outflow tract (or infundibulum). The major mechanisms contributing to RV pump function are different from those of the left ventricle and include (1) inward motion of the RV free wall (“bellows effect”); (2) contraction of the longitudinal fibers, which shortens the RV long axis by drawing the tricuspid annulus toward the apex; (3) interventricular septal motion; and (4) circumferential shortening of the RV outflow tract. RV contraction occurs in a peristaltic fashion starting at the inflow tract and proceeding to the outflow tract, with a 25- to 50-ms time delay between inflow and outflow. RV contraction is generated by a deep subendocardial layer of longitudinal fibers that cause a base-to-apex shortening and a superficial subepicardial layer of circumferential fibers running parallel to the atrioventricular groove that cause the inward transversal contraction. The myocytes in the subepicardial layer are arranged more circumferentially than in the left ventricle and the third middle layer of spiral fibers that is present in the left ventricle is lacking on the right side. Thus RV contraction relies more heavily on longitudinal shortening than does the LV contraction. The right ventricle also has helical fibers and undergoes torsion. Both ventricles have mutually encircling epicardial circumferential fibers and share the same interventricular septum and intrapericardial space, explaining the phenomenon of ventricular systolic and diastolic interdependence, in which alterations in the shape, size, and compliance of one ventricle can affect the other.
RV adaptation to disease is determined by the degree of pressure overload, volume overload, and alterations in intrinsic contractility. The right ventricle, as opposed to the left ventricle, ejects blood into a low-pressure, high-compliance pulmonary circulation. Thus, the right ventricle tolerates volume overload quite well and may remain well adapted to right-sided valvular regurgitant lesions or interatrial left-to-right shunt for extended periods of time. Conversely, the right ventricle is very sensitive to changes in afterload. A brisk increase in pulmonary vascular resistance (PVR; e.g., massive pulmonary embolism) induces acute dilation and rapid RV pump failure. However, a gradual increase in PVR allows for RV adaptive remodeling, first by increasing its contractility and mass (concentric hypertrophy mainly due to the circumferential layer) ( Fig. 9.3 ). This explains the observed shift in contraction from a longitudinal to a circumferential pattern in the RV free wall compared with the normal right ventricle. When this adaptation fails, maladaptive RV remodeling leads to RV dilation, eccentric hypertrophy, worse systolic and diastolic function, and decreasing LV preload because of competition for space within the pericardium. It seems that even minor increases in PVR, such as in patients with chronic obstructive pulmonary disease (COPD) and no significant pulmonary hypertension (PH), are enough to generate important structural and functional changes, such as RV hypertrophy, dilation, and subsequently dysfunction. Similarly, modest increases in mean airway pressure during positive pressure ventilation can reduce RV contractile performance and lower cardiac output even when RV preload is maintained.


Deformation metrics of right ventricular myocardial function
Strain and strain rate are parameters reflecting RV global and regional systolic function. Longitudinal strain is calculated as the percentage of systolic shortening of the RV wall from base to apex, and longitudinal strain rate is the rate of this shortening. As a measure of myocardial deformation, RVLS is less confounded by overall heart motion, translation, and loading conditions ( Fig. 9.4 ) than other conventional parameters of RV longitudinal function, such as tricuspid annular plane systolic excursion (TAPSE) and tissue Doppler imaging (TDI) S-wave velocity.

RVLS can be derived by TDI or by speckle-tracking methods. Two-dimensional (2D) speckle-tracking echocardiography (STE) is the current standard for clinical practice, being less angle dependent, feasible, and reproducible than TDI strain. However, the feasibility of 2D STE-derived strain relies upon good image quality, optimal temporal resolution (50–90 fps), and lack of foreshortening, dropouts, and reverberations.
Accordingly, the latest American Society of Echocardiography (ASE)/European Association of Cardiovascular Imaging (EACVI) guidelines on cardiac chamber quantification by echocardiography have recommended RVLS for clinical use as a sensitive and reproducible index of RV performance. However, the lack of uniformity on the software, method, and definition used for measuring and reporting RV strain in various studies and the limited reference values have precluded its full implementation in clinical practice.
In 2018, the EACVI/ASE/Industry Task Force published recommendations for the standardization of RV strain image acquisition and analysis for both clinical and scientific purposes. Also, more robust normative values have become available.
As for the left ventricle, most available evidence pertains for RV strain in the longitudinal direction, which is the currently recommended parameter. Because of the thin RV free wall, the computation of transversal (radial) strain is not accurate and, even though such a measure might be clinically relevant in some conditions, this parameter is not recommended for routine use. Because the obliquely oriented layer of myocardial fibers is not represented in the right ventricle, there is no powerful influence of twist mechanics to overall RV contraction.
As is the case on the left side, strain rate is less load dependent than strain and conceptually a better index of RV “contractility.” However, it has lower clinical feasibility than strain and is currently limited to research purposes.
Image acquisition and postprocessing of RV longitudinal strain
The recommended acquisition for RVLS quantification by 2D STE is the RV-focused apical four-chamber view ( Fig. 9.5 ), as this section allows a better definition of RV free wall and provides more reproducible measurements of RVLS than the standard four-chamber view centered on the left ventricle. This image is obtained from a more lateral position of the transducer than the required for standard apical four chamber, while taking care to position the LV apex at the center of the scanning sector and display the largest RV dimensions (longitudinal and transversal), the apex, and the entire RV free wall throughout the cardiac cycle ( Fig. 9.6 ). To achieve a correct orientation, the RV-focused apical four-chamber view also should not display the aortic valve (which would imply a cut plane position that is too anterior) nor the coronary sinus (a cut plane position that is too posterior) but only the interatrial septum. Of note, the strain values obtained from the RV-focused view are larger in magnitude than those obtained from the standard apical four-chamber view. This documented lack of interchangeability of strain analysis between the two approaches has important practical implications for patient follow-up and for the reference values used for reporting RV function abnormality (see Reference Values, later), which should follow a consistent approach with the recommendations from EACVI/ASE/Industry Task Force.


The region of interest (ROI) should be manually traced and should include both the RV free wall and septum, and its width should be adjusted to cover the thickness of RV free wall (≈ 5 mm in normal subjects and larger in the presence of RV hypertrophy) (see Fig. 9.6 ). Care should be taken to avoid placing the ROI on the pericardium (leading to underestimation of strain magnitude) or too low, below the tricuspid annulus (leading to paradoxic systolic lengthening in this region caused by atrial filling) (see Fig. 9.6 ). If the ROI width is too narrow, encompassing mainly the endocardial layer, strain magnitude will be overestimated. Other software measuring the strain at the endocardium might request only an endocardial delineation of RV cavity by a contour line and provide different values than software measuring the full wall or midmyocardium.
Before validating the measurements, tracking quality should be verified visually from the cine loops displaying the dynamic deformation of the ROI during the cardiac cycle and from the strain curve patterns. Doppler tracings of the tricuspid and pulmonary valves should be used to determine the timing of end diastole and end systole, and the strain peak value during systole (i.e., peak systolic strain) should be recorded for clinical purposes.
The segmentation of the RV free wall between apex and base includes three segments (basal, mid, and apical segments), which have equal length at end diastole. The septum is segmented in a similar manner. For reporting RVLS, the average of the three segments of the free wall is recommended and should be labeled as RVFWLS. If the septum was included in the strain average, this should be clearly acknowledged because the measurements of RV strain that include both free wall and interventricular septum (six segments, labeled RV4CLS) are smaller in magnitude than the three segments of RVFWLS. Although RVFWLS is most commonly used, there are circumstances when RV4CLS might be of interest, especially when there is potential septal involvement.
Finally, in addition to amplitude parameters, temporal parameters such as the time-to-peak strain (time from the onset of R wave on electrocardiogram [ECG] to maximum RV segment longitudinal shortening) can also be assessed. RV mechanical dispersion can be calculated as the standard deviation of time-to-peak strain in a six-RV-segment model ( Fig. 9.7 ). Despite the fact that RV mechanical dispersion can be obtained also from a three-segment model of the RV (including the RV free-wall segments only), the six-RV-segment model is more robust for calculation of standard deviation.

The learning curve for novice readers to achieve independent competency in consistency and reproducibility of RVFWLS has been studied by Chamberlain et al, who described the number of studies required to achieve expert level of competency (intraclass correlation coefficient >0.90) among all novice observers. No significant differences in agreement and reproducibility were observed between novices and experts in RVFWLS analyses after they had reached a plateau (100 studies) in their learning curve, and substantial improvement was seen after 25 cases ( Fig. 9.8 ).

Software packages used to measure RV myocardial deformation
There are several available software packages, including RV Strain (EchoPAC, GE Vingmed, Horten, Norway), Syngo Velocity Vector Imaging (VVI) (Siemens Healthineers, Mountain View, CA, USA), EchoInsight Right Ventricle (RV) (Epsilon Imaging, Ann Arbor, MI, USA), AutoStrain RV (Image Arena 4.6, TomTec Imaging Systems, Unterschleissheim, Germany), and QLab 13 (Philips Medical Systems, Andover, MA, USA).
EchoPAC PC
Q-analysis and AFI (Automatic Function Imaging, EchoPAC Software Only) are the most commonly used software packages to measure RV strain. Both algorithms are not dedicated to the right ventricle—they were developed for the left ventricle and should be adapted to the shape of the right ventricle in the RV focused four-chamber apical view. Recently, a dedicated RV strain analysis software package adapted for the shape of the right ventricle has become commercially available (RV Strain, EchoPAC, GE Vingmed, Horten, Norway) ( Fig. 9.9 ). After having identified the RV endocardial border on an end-systolic frame of the RV focused four-chamber apical view by clicking three separate points (apex, and lateral and septal points of the tricuspid annulus), these software packages automatically identify the ROI and track the motion of the speckles on subsequent frames. The ROI can be adjusted to fit the thickness of the RV free wall and the septum. Adequate tracking has to be verified in real time and can be corrected by adjusting the ROI width or manually adjusting the contour to ensure optimal tracking (see Fig. 9.9 ). The algorithm will then calculate peak strain values for the six segments (three in free wall and three in septum). RV strain automatically calculates the RV4CLS for the entire RV myocardium, the RVFWLS, and the TAPSE parameter (see Fig. 9.9 ). The trace window will show individual strain curves for the six segments, as well as the RV4CLS (dotted line) and the RVFWLS (dashed line) curves of Fig. 9.9 . EchoPAC PC is a vendor-specific software and can only analyze raw data obtained from GE ultrasound systems.

Syngo velocity vector imaging
Syngo VVI is an offline software package for measuring myocardial deformation, which has been developed for the left ventricle. However, the algorithm can be adapted to measure RV strain. After manually drawing the RV endocardial border over one frame, endocardial borders are automatically tracked throughout the cardiac cycle. Myocardial velocity is calculated as the ratio between the time interval and frame-to-frame displacement ( Fig. 9.10 ). These velocity vectors (see Fig. 9.10 B) are displayed throughout the cardiac cycle, and the 2D strain and strain rate are calculated by comparing the speckle displacement in relation to other speckles along the endocardial border throughout the cardiac cycle. This software can measure strains in echocardiographic images from all models of commercially available ultrasound systems stored in DICOM format and automatically provides the time to peak segmental strain in addition to the segmental peak strain values (see Fig. 9.10 A).

Echoinsight right ventricle
The EchoInsight RV application enables users to perform RVFWLS imaging analysis as its default and allows flexibility by also providing four-chamber and regional RV strain analysis ( Fig. 9.11 ). In addition, based on the user’s indicated strain imaging region of interest, the EchoInsight RV application will automatically provide end systole and diastole RV areas, fractional area shortening, TAPSE, S′, and linear measurements. The EchoInsight RV application is a vendor neutral platform and can measure RV strain on all echocardiographic images stored in DICOM format.

Autostrain RV
The AutoStrain RV is a fully automated, offline software package dedicated to the measurement of RVLS. It does not require any other operator intervention than launching the algorithm on the proper RV-focused apical view, even if editing of the automated tracking is also possible. The algorithm tracks the endocardial border throughout the cardiac cycle ( Fig. 9.12 ). This software package is vendor independent and can measure RV strain on any echocardiographic image stored in DICOM format. The algorithm provides RV4CLS and RVFWLS but no RV segmental strain values.

Intervendor reproducibility of RV strain metrics has been tested in 35 patients by comparing measurements obtained from two vendor-specific (QLab 10.4, Philips Medical Systems, Andover, MA, USA and EchoPAC BT13, GE Vingmed, Horten, Norway) and one vendor-independent (ImageArea, 2D CPA version 1.2, TomTec Imaging Systems, Unterschleissheim, Germany) software package (all of them designed for the left ventricle and adapted to the RV shape). The study showed that, despite widespread variability in the data, there were no major systematic differences with vendor-specific imaging or approaches for calculating RVLS, despite system differences. There was acceptable variability for global RVLS but large variability (sometimes 30%–40%) for regional strains, which make regional RVLS less appealing.
Reference values
The definition of “abnormal” in echocardiography is often based on the availability of reference values, which point to a range that have been defined as normal when looking at biological metrics. Such a range of values is commonly calculated by taking (twice) the standard deviation from the mean or considering certain percentiles. The suspicion or even confirmation of an abnormality in the considered metrics is then established by demonstrating that the value measured exceeds the upper or lower limit of the reference value. As is often the case, the accuracy of the measurements from which the reference values are obtained may depend on the conditions and specific method employed to collect and analyze data.
Until 2018, the measurement of RV strain components was not standardized, and both the conventional apical four-chamber and the RV focused four-chamber view , , were used by the investigators scanning healthy subjects to obtain reference values for RV strain. We know that the use of the RV-focused four-chamber view provides higher absolute values of RV four-chamber and free-wall longitudinal strain measurements than those measured on conventional apical four-chamber view. Moreover, all available studies reporting reference values for the RV strain components were performed adapting software packages developed to measure the strain components of the left ventricle. Finally, in contrast to the large number of studies reporting the reference values for the different components of LV and left atrial (LA) myocardial deformation using STE, the number of studies that included healthy subjects and reported the normal values of RV global and free wall longitudinal systolic strain is quite limited. Indeed, few of these comply with the recommendations of the American Guidelines for Reference Intervals in the Clinical Laboratory (Clinical and Laboratory Standards Institute), which requires at least 120 healthy subjects as the minimal sample size to be enrolled in such studies, to avoid bias in estimation of threshold parameters.
Table 9.1 summarizes the data obtained from such studies in 1457 healthy volunteers. , Most of the measurements were obtained with EchoPAC (GE Vingmed, Horten, Norway; n =1143), and the remaining with Syngo VVI (Siemens Healthineer, Mountain View, CA; n =186) and TomTec 2.0 (TomTec Imaging Systems, Unterschlessheim, Germany; n =128). On average, absolute RV strain values obtained with EchoPac (using the RV-focused four-chamber view) were larger than those obtained either with Syngo VVI or TomTec 2.0 (see Table 9.1 ). A meta-analysis of the available data showed that the lower limits of normality (LLN) of RV four-chamber longitudinal systolic strain (in absolute values) were 17.4% for EchoPac and 14.1% for Syngo VVI, whereas the LLN of RV free-wall longitudinal systolic strains (in absolute values) were 20.2% for EchoPAC, 15.5% for TomTec 2.0, and 13.4% for Syngo VVI. No data were available for Q-Lab (Philips Medical Systems, Andover, MA) or 2D Wall Motion Tracking (WMT, Canon Medical Systems, USA). On average, the LLN in women showed higher absolute values of both RV four-chamber and free-wall longitudinal strain than men (18.2% vs 17.3% and 20.7% vs 20.3%, respectively). There does not seem to be a significant change of RVLS values with age—at least until the age of 60 years ( Fig. 9.13 )—but it is also true that no studies were able to include ≥120 healthy older adult subjects. Although it has not been possible to perform subgroup analyses in healthy subjects ≥60 years old, there are suggestions that RV strain may fall in old age (see Fig. 9.13 ). Finally, it should be noticed that the normal values (and thereby, the LLN) for RV four-chamber longitudinal systolic strain (including both interventricular septum and RV free wall) are significantly lower than the values of RV free-wall longitudinal systolic strain.
Author | Year | Sample Size, n | Women, n (%) | Age, years | Ethnicity | Software Package | RV Four-Chamber Strain, % | LLN, % | RV Free-Wall Strain, % | LLN, % |
---|---|---|---|---|---|---|---|---|---|---|
Fine et al | 2013 | 186 | 114 (61) | 44±16 | Whites | Syngo (VVI) | 20.4±3.2 | 14.1 | 21.7±4.2 | 13.4 |
Chia et al | 2014 | 136 | 67 (49) | 45±15 | Whites and Asians | Echo-Pac (-) | 22.3±2.4 | 17.6 | 27.3±3.3 | 20.8 |
Muraru et al | 2016 | 276 | 153 (55) | 42±15 | Whites | Echo-Pac (BT113) | 25.8±3.0 | 19.9 | 30.5±3.9 | 22.8 |
Morris et al | 2017 | 238 | 119 (50) | 36±12 | Whites and Asians | Echo-Pac (BT113) | 24.5±3.8 | 17.0 | 28.5±4.8 | 19.0 |
Park et al | 2017 | 493 | 261 (53) | 47±15 | Asians | Echo-Pac (BT201) | 21.5±3.2 | 15.2 | 26.4±4.2 | 18.1 |
McGhie et al | 2017 | 128 | 64 (50) | 44±13 | Whites | TomTec 2.0 | – | . | 25.4±5.0 | 15.6 |
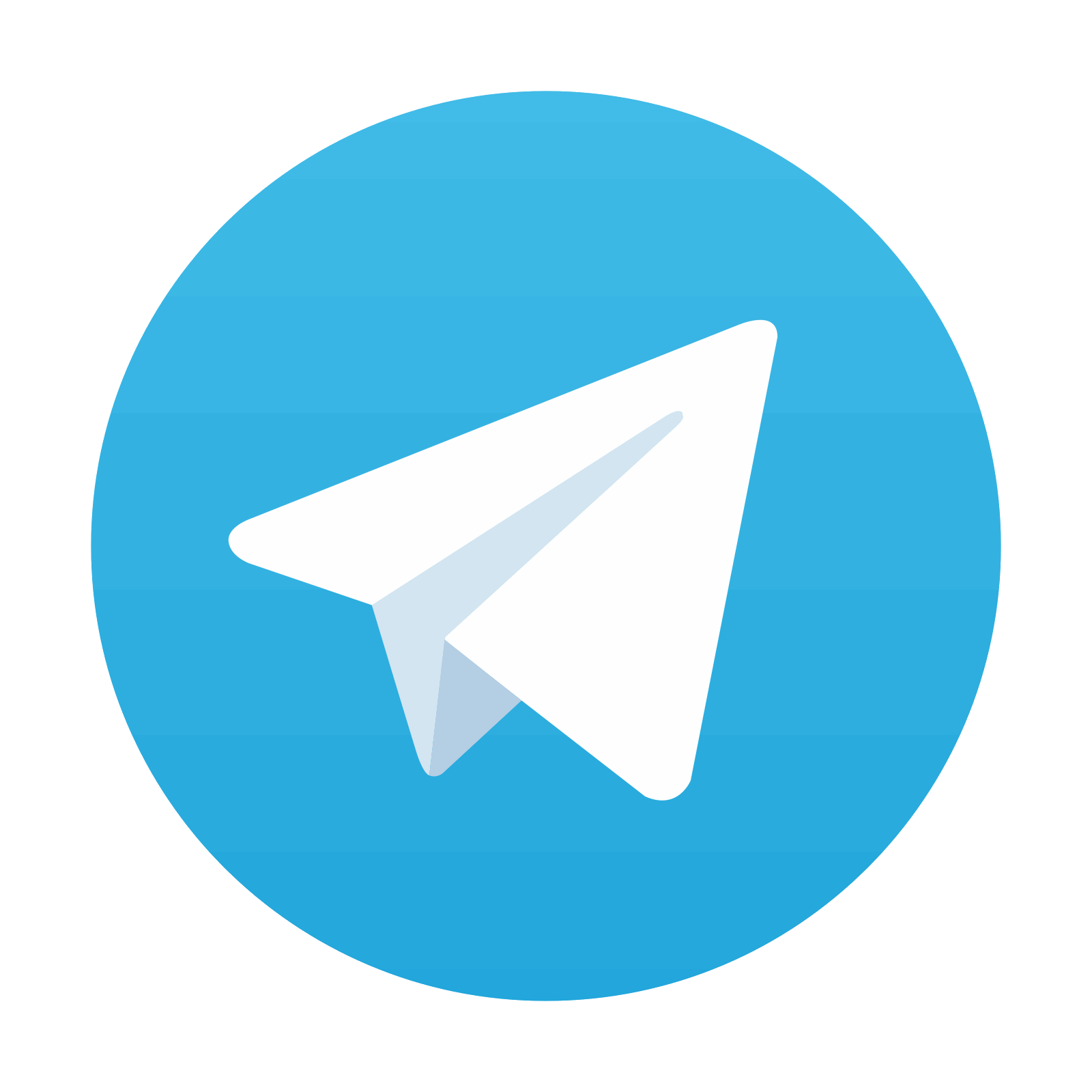
Stay updated, free articles. Join our Telegram channel

Full access? Get Clinical Tree
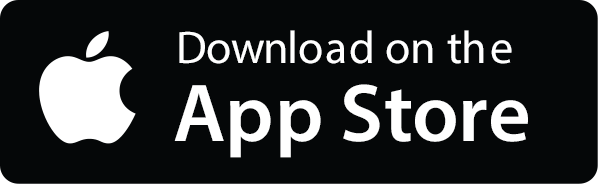
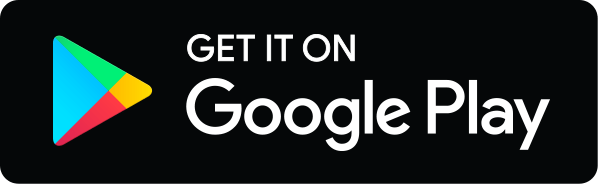
