Background
The aim of this study was to evaluate the capacity and reproducibility of three-dimensional echocardiographic (3DE) strain parameters in the assessment of global left ventricular (LV) systolic function.
Methods
A total of 128 subjects with differing LV ejection fractions were investigated using two-dimensional echocardiographic (2DE) and 3DE strains. Three-dimensional echocardiographic strain allows obtaining longitudinal, circumferential, radial, and area strains. First, values of global longitudinal strain (GLS) by 2DE and 3DE speckle-tracking analyses were compared. Thereafter, 3DE strain parameters were correlated with LV ejection fraction and indexed output. Last, the variability of 3DE versus 2DE strain measurements as well as recorded time of analysis were assessed.
Results
After excluding 21 patients for insufficient image quality, four for arrhythmia, two for severe valvular disease, and one for severe dyspnea, the final population consisted of 100 patients. Comparison between 2DE and 3DE GLS revealed high correspondence ( r = 0.91, y = 1.04 x − 0.71) and mean error measurement of −1.3% (95% confidence interval, −5.7 to 3.2). Among strain parameters, global area strain exhibited the highest correlation with LV ejection fraction ( y = −1.65 + 10.4, r = −0.92, P < .001). Intraobserver measurement variability proved acceptable: 8% for GLS (vs 6% on 2DE analysis), 7% for circumferential strain (vs 15% on 2DE analysis), 7% for radial strain (vs 33% on 2DE analysis), and 5% for global area strain. The mean error between two measurements was lower with 3DE than 2DE analysis for circumferential and radial strains but similar for GLS. The mean time of analysis was of 117 ± 16 sec for 3DE analysis, which was 25% less than for 2DE analysis ( P < .001).
Conclusions
Of all strain parameters, new 3DE area strain correlated best with common LV systolic function parameters and is thus the most promising approach, while all 3DE strain markers exhibited good reproducibility.
Thanks to Doppler tissue imaging and speckle-tracking two-dimensional echocardiographic (2DE) imaging, the quantification of left ventricular (LV) function has moved beyond the simple ejection fraction (EF) measurements and qualitative analysis of regional wall motion. The normal values of the three components of LV deformation (longitudinal, circumferential, and radial strains) were previously determined using speckle-tracking 2DE imaging in various populations, such as patients with familial hypertrophic cardiomyopathy, aortic stenosis, type 2 diabetes, paroxysmal atrial fibrillation, and heart failure, with promising results in terms of prognostic evaluation and patient management. Although 2DE global longitudinal strain (GLS) was shown to be reproducible and accurate, circumferential and radial strains were less reliable, with measurement variability of >10% and 15%, respectively, which limits their use for LV systolic function evaluation in clinical practice.
Recent technology progress has led to the development of a high-resolution three-dimensional (3D) echocardiographic (3DE) transducer, facilitating the acquisition of volumetric data sets with high image quality, in addition to a computerized algorithm for rapid online or offline contour tracking. With only minimal operator interaction, this development allows for semiautomated and simultaneous data analysis of LV volumes, EF, and strains (longitudinal, circumferential, radial, and area deformations).
Although 2DE strain evaluation is subject to the out-of-plane motion of the speckles, the volumetric 3DE myocardial deformation approach appears more promising. We hypothesized that 3DE strain parameters, especially area strain, would be more reproducible, accurate, and promising markers of global LV systolic function in clinical practice in comparison with 2DE strain data.
This study was designed to evaluate GLS obtained by 3DE and 2DE imaging, to correlate 3DE strain parameters with 3D LV EF and indexed cardiac output by 2DE and Doppler imaging, and finally to evaluate variability of 3D strain parameters in comparison with 2DE strain data.
Methods
Study Protocol
Patients scheduled for routine echocardiography in our cardiology department in the course of hospitalization or during cardiology visits were prospectively included and evaluated using both 2DE and 3DE imaging.
The 2DE and 3DE acquisitions were obtained successively for all subjects by the same physician, with 2DE and 3DE analyses being performed retrospectively and offline.
Excluded from the study were patients with insufficient ultrasound image quality (defined as more than three LV segments that were not optimally visualized using conventional 2DE), moderate or greater valvular disease, unstable clinical condition, severe dyspnea precluding breath-holding for ≥6 sec, or arrhythmia.
Systolic and diastolic blood pressures were systematically measured during the echocardiographic studies. Written informed consent was obtained from all subjects, and the study was approved by the local ethics committee of the Bordeaux University Hospital.
Transthoracic 2DE and 3DE Data Acquisitions
The patients were examined in the left lateral decubitus position using a Vivid E9 commercial ultrasound scanner (version BT11; GE Vingmed Ultrasound AS, Horten, Norway) with phased-array transducers (M5S-D and 4V-D).
Two-dimensional data acquisitions were obtained, including parasternal long-axis and short-axis views and three standard apical views. For each view, three consecutive cardiac cycles were recorded during quiet respiration. Grayscale recordings were optimized for LV evaluation at a mean frame rate of ≥50 frames/sec. To evaluate cardiac output, conventional acquisitions were obtained while zooming on the LV outflow tract area and with the pulsed Doppler cursor placed at the same level in the apical five-chamber view. Color Doppler recordings were made to exclude valvular dysfunction. Doppler flow recordings were performed with a horizontal sweep velocity of 100 mm/sec.
For 3DE, a fully sampled matrix-array transducer with almost 3,000 active elements was used (4V-D; GE Vingmed Ultrasound AS). The 3DE data acquisition was obtained in an adjustable volume divided into six subvolumes. The acquisition of subvolumes was steered electronically using the ultrasound system, with the transducer kept in a stable position. The acquisition was triggered to the R wave of the electrocardiogram on consecutive heartbeats. To ensure the correct spatial registration of each subvolume, the acquisitions were obtained in an end-expiratory breath-hold lasting 6 to 8 sec (depending on the heart rate) and recorded at the LV apex with a mean volume rate of ≥30 volume/sec and a six-beat acquisition to ensure optimal temporal and spatial resolution. Care was taken to include the entire LV cavity and myocardium, including the epicardium, within the pyramidal scan volume.
To allow for 3DE repeatability analysis (interexamination evaluation), a second 3DE volumetric acquisition was obtained by the same operator ≥5 min after the first. The resultant 3DE data sets acquired from the machine were transferred to a separate workstation for offline data analysis via the hospital picture archiving and communication system, under blinded conditions.
Analysis of 2DE Imaging
All standard measurements, including LV outflow tract diameter and LV outflow tract time-velocity integral, were taken to calculate LV output indexed to body surface area. On the basis of apical four-chamber and two-chamber views, LV volumes and EF were measured according to Simpson’s rule. All measurements were obtained using the average of three cardiac cycles.
For 2DE strain analysis, the software automatically tracked the contour on subsequent frames after three endocardial markers were placed in an end-diastolic frame. Adequate tracking could be verified in real time and corrected by adjusting the region of interest or by manually correcting the contour to ensure optimal tracking. Two-dimensional longitudinal strain was assessed in apical views. Average longitudinal strains were calculated for the 17 segments in relation to the strain magnitude at aortic valve closure. Longitudinal systolic deformation was characterized as shortening, and using the strain definition, systolic indices yielded a negative value. Circumferential and radial strains were obtained from a mid parasternal short-axis view data set (six-segment analysis).
Three-Dimensional Echocardiographic Strain Quantification
Data analysis was performed offline using the original raw data from all 3DE data sets on an EchoPAC software workstation (version BT11, 4D Auto LVQ; GE Vingmed Ultrasound AS) for semiautomated endocardial surface detection.
Data acquisition was conducted in several stages. First, alignment with presentation of four-chamber, two-chamber, and three-chamber apical views, as well as the transversal plane, was performed. Orientation was performed automatically.
Second, for end-diastolic volume, the operator placed, in end-diastole, one point in the middle of the mitral annulus plane and a second point at the LV apex. The software generated an end-diastolic endocardial border tracing. Papillary muscles were included within the LV cavity. Adjustments of the automatic tracing were performed manually by the operator to reposition it closer to the endocardial border.
Third, for end-systolic volume, the same process was repeated in end-systole. In the case of significant deviations, the operator was allowed to adjust the trace closer to the endocardial border.
The analysis enabled the acquisition of LV volumes and LV EF in <30 sec. A minimum of four initial points had to be selected by the user. Additional clicks, if necessary, were quantified. The duration of the time taken to obtain volumes and to measure LV EF using 3DE and 2DE imaging was compared.
Fourth, for the volume waveform, the correct alignment of the contours with the endocardium during the cardiac cycle was controlled.
Fifth, a second semiautomated delineation was made in relation to the epicardium to delineate the region of interest for strain analysis.
Last, after treatment by the software (1 min), the final 3DE analysis allowed the measurement of 3DE GLS, global circumferential strain (GCS), global area strain (GAS), and global radial strain (GRS), which were computed at end-systole ( Figure 1 ). GAS was calculated as the percentage variation in the surface area defined by the longitudinal and circumferential strain vectors. The duration of analysis time to obtain all the data including strains was calculated and compared with that for 2DE analysis. The mean time taken to obtain LV volumes, EF, and strains was 2 min.

Statistical Analysis
All statistical analyses were performed using SPSS for Windows version 15.0 (SPSS, Inc., Chicago, IL). All data are expressed as mean ± SD.
To compare 3DE with 2DE GLS and to correlate 3DE strains with LV EF and indexed cardiac output, linear regression analyses were performed, and Pearson’s correlation coefficients were calculated. Agreements were assessed using the method proposed by Bland and Altman for the comparison of 3DE and 2DE GLS.
To assess intraobserver variability, 2DE and 3DE data from 60 patients were analyzed twice offline by the same operator, with an interval of ≥1 week between the two analyses. A second observer blinded to the results of the preceding investigations similarly performed the 60 analyses to assess interobserver variability. Variability was expressed as percentage, intraclass correlation coefficient, and mean difference ± SD between two observations. Percentage intraobserver variability was calculated as the absolute difference divided by the average of the two measurements realized by the first operator. Percentage interobserver variability was calculated as the absolute difference divided by the average of the measurements performed by the two operators. Agreement was assessed using the method proposed by Bland and Altman. In addition, the repeatability (interexamination variability) of 3D strain measurements was determined by the same operator repeating the two assessments, separated by an interval of ≥1 week.
Results
Study Population
Among the 128 screened subjects, 21 patients were excluded on account of having at least three LV segments not visualized on 2DE (considered as insufficient image quality), four patients for arrhythmia, two patients for moderate to severe valvular disease, and a further patient for severe dyspnea precluding breath-holding. A total of 100 subjects were included in the final analysis: 21 subjects without echocardiographic abnormalities and diagnosed cardiac disease, 28 with dilated cardiomyopathy, 22 with ischemic cardiomyopathy, 10 with paroxysmal arrhythmia, six with mild valvular disease, two with anthracycline chemotherapy, two with myocarditis, two with cardiac amyloidosis, two with cardiac hemochromatosis, one with familial hypertrophic cardiomyopathy, one with heart transplantation, and three for cardiac checkup. The population comprised 76% men, with a mean age of 53.3 ± 19.4 years and a mean LV EF of 50.4 ± 14.7% by 3DE imaging. Other clinical and echocardiographic characteristics of the study population are summarized in Table 1 .
Variable | Value |
---|---|
Men | 76% |
Age (y) | 53.3 ± 19.4 |
Body surface area (m 2 ) | 1.8 ± 0.2 |
Systolic blood pressure (mm Hg) | 120.3 ± 18.9 |
Diastolic blood pressure (mm Hg) | 70.4 ± 12.5 |
Heart rate (beats/min) | 66.0 ± 11.4 |
2DE LV end-diastolic diameter (mm) | 56.3 ± 11.2 |
Indexed LV output by Doppler (L/min/1.73 m 2 ) | 3.9 ± 0.9 |
3DE LV end-diastolic volume (mL) | 129.0 ± 60.3 |
3DE LV end-systolic volume (mL) | 69.7 ± 52.6 |
3DE LV EF (%) | 50.4 ± 14.7 |
2DE GLS (%) | −14.5 ± 5.9 |
3DE GLS (%) | −13.4 ± 4.8 |
3DE GAS (%) | −24.3 ± 8.2 |
3DE GCS (%) | −14.5 ± 5.6 |
3DE GRS (%) | 36.7 ± 15.4 |
Time of Analysis and 3DE Algorithm Adjustments
The total duration of offline analysis of the 3DE data set (LV volumes, EF, and strains) averaged 117 ± 16 sec, including manual initialization ( Table 2 ), which was 25% less than the time used for 2DE analyses ( P < .001). The adjustments made by the operator required 2.6 ± 0.9 clicks for the correction of the endocardial contour and 1.2 ± 1.2 clicks for the epicardial contour, which slightly increased the analysis time by up to 30 sec. Because of inadequate tracking, the software automatically excluded an average of 0.8 ± 1.0 myocardial segments from the 17 taken for global analysis.
Variable | 2DE Analysis Time (sec) | 3DE Analysis Time (sec) | P Value, 2DE Versus 3DE Analysis |
---|---|---|---|
Volumes and EF | 57.9 ± 6.9 | 26.1 ± 4.1 | 2.6 × 10 −20 |
Two-dimensional longitudinal strain | 59.3 ± 8.1 | — | — |
All strains | 99.7 ± 10.2 | 78.7 ± 18.4 | 5.5 × 10 −6 |
Volumes and strains | 157.6 ± 14.0 | 116.9 ± 16.1 | 6.3 × 10 −12 |
Comparison of GLS between 2DE and 3DE Analyses
The correlation and mean error of measurement between 2DE and 3DE analysis for GLS were high, at r = 0.91 ( y = 1.04 x − 0.71) and −1.3% (95% confidence interval, −5.7 to 3.2), respectively. Figure 2 displays linear regression, with Bland-Altman analysis showing a narrow dispersion.

Comparison between 3DE Strain Parameters and LV EF
Figure 3 illustrates the linear regression analysis of the different 3D strain parameters, with LV EF and LV output indexed to body surface area. The highest correlation with 3D LV EF was obtained for GAS ( y = −1.65 + 10.4, r = −0.92, P < .001). As expected, GLS was correlated less with 3D LV EF ( r = −0.84) than were GCS ( r = −0.91), GRS ( r = 0.91), and GAS ( r = −0.92).
Comparison between 3DE Strain Parameters and Indexed LV Output
The highest correlations were obtained for GAS ( y = −0.1 x + 1.5, r = −0.86, P < .001). GLS and GCS were correlated less with indexed cardiac output ( r = −0.83 and r = −0.83, respectively) than was GRS ( r = 0.85) or GAS ( r = −0.86).
Variability of 3DE Measurements
Mean intraobserver and interobserver variability for 2DE and 3DE strains is presented in Table 3 (mean percentage variability, intraclass correlation coefficient, and mean error measurement).
Strain Analyses | 2DE Analysis ( n = 60) | 3DE Analysis ( n = 60) | ||||
---|---|---|---|---|---|---|
% Variability | r | Mean Difference | % Variability | r | Mean Difference | |
Intraobserver | ||||||
Longitudinal | 6 ± 10 | 0.98 | −0.1 ± 1.0% | 8 ± 10 | 0.95 | 0.04 ± 1.5% |
Circumferential | 15 ± 15 | 0.88 | −0.6 ± 2.6% | 7 ± 10 | 0.97 | −0.5 ± 1.4% |
Radial | 33 ± 32 | 0.83 | 2.2 ± 11.0% | 7 ± 7 | 0.98 | 1.0 ± 3.3% |
Area | 5 ± 6 | 0.98 | −0.4 ± 1.7% | |||
Interobserver | ||||||
Longitudinal | 7 ± 7 | 0.97 | 0.1 ± 1.3% | 10 ± 8 | 0.90 | 0.2 ± 2.0% |
Circumferential | 13 ± 13 | 0.93 | 0.2 ± 2.3% | 10 ± 9 | 0.89 | 0.5 ± 2.1% |
Radial | 34 ± 37 | 0.81 | −2.3 ± 11.7% | 8 ± 7 | 0.93 | −0.5 ± 5.2% |
Area | 6 ± 6 | 0.95 | 0.3 ± 2.3% |
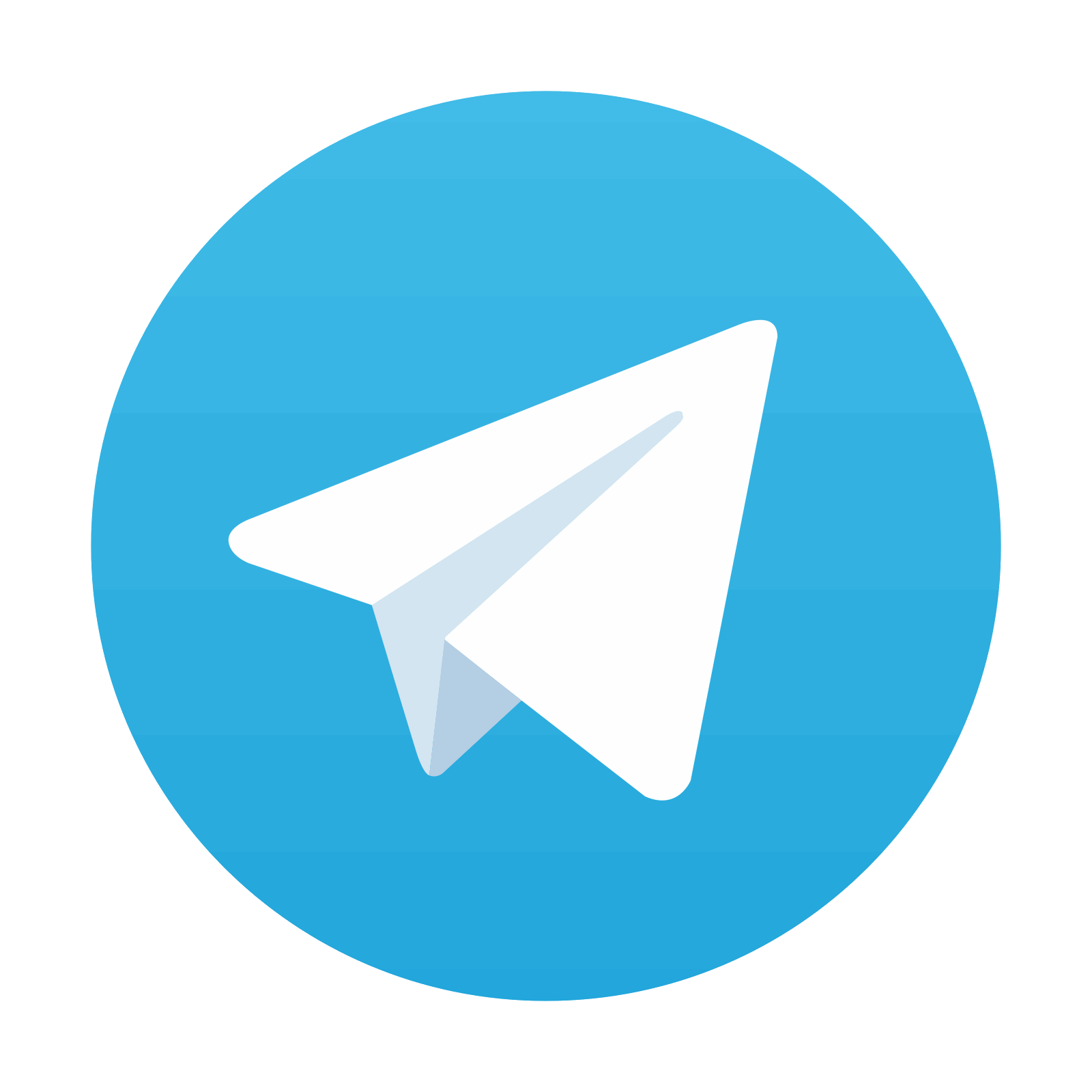
Stay updated, free articles. Join our Telegram channel

Full access? Get Clinical Tree
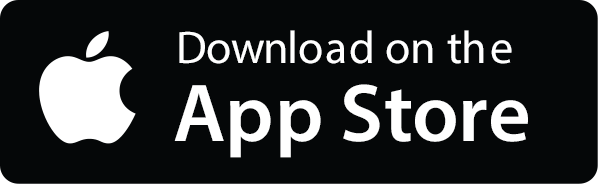
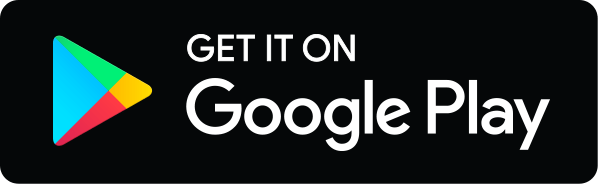
