Critical heart disease in the newborn includes all congenital heart lesions that would result in neonatal demise unless immediate intervention is undertaken. It is estimated that congenital heart disease occurs in about 8 per 1000 live births.1 Not all congenital heart disease (CHD) presents in the newborn period. However, there are a handful of conditions that need immediate management in a newborn infant, the failure of which precludes survival. Roughly 3.5 in 1000 live births have critical CHD.1 Neonatal critical heart diseases can be broadly described as those that present as severe cyanosis (right heart obstruction) and those that present as shock (left heart obstruction). Table 5-1 lists common conditions in both categories. The common denominator to all critical heart diseases presenting in the neonatal period is that they need a patent ductus arteriosus for survival until further palliative procedures can be undertaken. To understand the physiology of these lesions, it is important to have knowledge of the circulatory changes that happen right after birth.
CHD Presenting with Cyanosis | CHD Presenting with Shock |
---|---|
Tetralogy of Fallot (TOF) | Critical aortic stenosis |
Dextro-transposition of great arteries (d-TGA) | Coarctation of aorta |
Tricuspid atresia | Interrupted aortic arch |
Total anomalous pulmonary venous connection (TAPVC) | Hypoplastic left heart syndrome (HLHS) |
Pulmonary atresia with intact ventricular septum (PAIVS) | |
Critical pulmonary stenosis |
Blood oxygenated in the placenta is returned by way of the umbilical veins. Most of the umbilical venous blood shunts through the ductus venosus to the inferior vena cava (IVC), which provides a low-resistance bypass.2 The IVC blood is composed of streams of hepatic venous blood, umbilical venous blood, and blood from lower extremities. The stream of umbilical venous blood selectively enters the left atrium, though the foramen ovale guided by the eustachian valve. The left ventricle (LV) pumps out this blood into the ascending aorta for distribution to the coronaries, head, and upper extremities.2 The superior vena caval stream and rest of the IVC blood pass into the right ventricle (RV). The RV pumps out this blood into the pulmonary trunk. A small amount of this blood enters the pulmonary circulation, and the rest passes through the ductus arteriosus into the descending aorta and finally reaches the placenta via the umbilical arteries. Figure 5-1 shows the key elements of fetal circulation. The main differences between fetal circulation and postnatal circulation consist of the following:
- Placental circulation provides gas exchange for the fetus
- Absence of gas exchange in the collapsed lungs; this results in very little flow of blood to the lungs
- Presence of a ductus venosus providing a low-resistance bypass for umbilical venous blood to reach the IVC
- Widely open foramen ovale to provide a route to the oxygenated blood to reach the left atrium
- Widely open ductus arteriosus to allow RV blood to reach descending aorta
Figure 5-1

Diagram of fetal circulation showing 4 sites of shunt: placenta, ductus venosus, foramen ovale, and ductus arteriosus. aa, artery; LA, left atrium; LV, left ventricle; RA, right atrium; RV, right ventricle; v, vein. (Reproduced, with permission, from Cunningham FG, Leveno KJ, Bloom SL, Hauth JC, Rouse DJ, Spong CY. Williams Obstetrics. 23rd ed. New York, NY: McGraw-Hill; 2010.)
After birth, the lungs expand and provide oxygenation of blood. The increased flow of blood to the pulmonary artery passing though the lungs and reaching the left atrium increases the left atrial pressure. The effect of this is that the septum primum approximates with the septum secundum and the foramen ovale closes functionally. Clamping of the cord increases the systemic vascular resistance (SVR). Thus, the systemic pressure increases, while the pulmonary artery pressure falls due to lowering of the pulmonary vascular resistance.2 As a result, flow of blood though the ductus arteriosus reverses. Instead of blood flowing from the pulmonary artery to the aorta, as in the fetus, the blood flows from aorta to pulmonary artery. The ductus arteriosus constricts and closes off. The pulmonary and systemic circulations thus separate from each other soon after birth.
Outcome of a newborn with critical CHD ultimately depends on the timely assessment and accurate diagnosis of the underlying defect, as well as the prompt evaluation of potential secondary end-organ damage. Fetal ultrasound has become a vital tool in prenatal diagnosis of critical CHD. Prenatal diagnosis avoids hemodynamic compromise that is frequently seen following postnatal diagnosis.3,4
In general, lesions that mandate delivery near a pediatric tertiary cardiac care center include (1) those that require immediate surgery or cardiac catheterization, (2) those that require ductal patency for cardiac output, and (3) those that require ductal patency for pulmonary blood flow.
Spontaneous delivery close to full term is generally recommended. Delivery room resuscitation should follow general guidelines for neonatal resuscitation. Airway should be stabilized. Vascular access should be obtained by placement of umbilical venous and arterial catheterization. Metabolic abnormalities and hypovolemia should be corrected. Inotropic agents and prostaglandin should be available and administered when indicated. Oxygen should be used with caution, particularly in those with single-ventricle physiology. Oxygen is a potent pulmonary vasodilator and will cause pulmonary overcirculation at the expense of systemic circulation. Conversely, oxygen should be administered when a cyanotic right-sided obstructive lesion is suspected or when there is concurrent lung disease. In general, arterial oxygen saturations should be maintained between 80% and 85%, which in a neonate with single-ventricle physiology and good cardiac output translates to a balanced circulation.
Once the neonate is resuscitated and stabilized, a thorough evaluation is warranted. Although there are numerous cardiac lesions, there are many similarities in their clinical presentation. Signs and symptoms include cyanosis, discrepant pulses, signs of congestive heart failure, and cardiogenic shock. The initial evaluation of a newborn with suspected critical CHD includes a thorough physical examination, 4-extremity blood pressure, preductal and postductal saturation, a chest radiograph (Figure 5-2), electrocardiogram, hyperoxia test, and an echocardiogram. Features particular to individual lesions will be discussed in detail later in this chapter.
Once the diagnosis is made, attention should continue to focus on the basic principles of neonatal life support and maintenance of a patent ductus arteriosus. Airway must be stabilized, vascular access must be secured, volume status and inotropic support must be maintained, and systemic and pulmonary circulations must be balanced. Prostaglandin E1 (PGE1) is administered in nearly all cases of critical CHD. When administration of PGE1 does not improve the clinical condition, total anomalous pulmonary venous return with obstruction, hypoplastic left heart syndrome with an intact atrial septum, or transposition of great arteries with restrictive atrial communication should be suspected, and urgent catheterization or surgical intervention is indicated.
For most neonates with critical CHD whose ductal patency is maintained with PGE1, attention to balancing pulmonary (Qp) and systemic circulation (Qs) is essential.5 This is particularly important for patients with single-ventricle physiology and complete intracardiac mixing of systemic and pulmonary venous return. In this situation, the relative resistances to flow govern the ratio of distribution of flow between the systemic and pulmonary circuits. Resistance to Qp is encountered by subvalvar or valvar pulmonary stenosis, pulmonary vascular resistance (PVR), and elevated pulmonary venous and left atrial pressures. Resistance to Qs likewise occurs by subvalvar or valvar aortic stenosis, aortic arch hypoplasia or coarctation, and SVR. The management goal of patients with single-ventricle physiology is to provide adequate pulmonary blood flow without compromising systemic oxygen delivery and tissue perfusion.
Ideally in patients with good cardiac output, with a mixed venous oxygen saturation (Svo2) of 60% and no lung pathology and thus a pulmonary vein oxygen saturation (Spvo2) of 100%, a systemic arterial oxygen saturation (Sao2) of 80% would represent a Qp:Qs ratio of 1:1, because pulmonary artery saturation (Spao2) is the same as Sao2 because of complete mixing. An Sao2 of 90% may lead to an Svo2 of 70% and a Qp:Qs of 2:1. An Sao2 of 70% and Svo2 of 50% will result in a Qp:QS of 0.66:1.
Neonates with unbalanced physiology can be broadly categorized as (1) those with inadequate Qp resulting in hypoxemia and (2) those with excessive Qp resulting in systemic hypoperfusion. Inadequate Qp results from increased PVR. Hence, to increase Qp, the underlying cause of diminished Qp should be identified. For example, patients with hypoplastic left heart spectrum hypoxemia because of restrictive interatrial communication should undergo transcatheter or surgical septostomy. Excessive Qp leads to hypotension, acidosis, and decreased tissue perfusion. It can result from either increased SVR or diminished PVR. Elevated SVR can be a result of increased exogenous catecholamines or secondary to exogenous vasoactive agents. Table 5-2 summarizes strategies to balance Qp:Qs.
Qp:Qs | |||
---|---|---|---|
Increased Qp:Qs | Decreased Qp:Qs | ||
Increased SVR | Decreased SVR | Increased PVR | Decreased PVR |
Epinephrine, high-dose dopamine | Oxygen, hyperventilation, alkalosis, nitric oxide | Hypoxia, hypercarbia, hypoventilation, acidosis | Milrinone, dobutamine |
Unrestrictive interatrial communication | Restrictive interatrial communication |
Cyanosis results from right-to-left shunting of blood. It is invariably due to some degree of right heart obstruction. Consequently, the venous blood has no outlet and has to mix with the arterial blood across either an interatrial communication (atrial septal defect [ASD]) or interventricular communication (ventricular septal defect [VSD]). In the fetal life, the venous blood shunts across an ASD or a VSD to get to the aorta. Depending on the degree of right heart obstruction, there may be little flow from the pulmonary artery to the aorta across a patent ductus arteriosus (PDA) to even reversal of flow from the aorta to the pulmonary artery. This may be the first clue on a fetal ultrasound for suspicion of a cyanotic heart disease. Soon after birth, the lung is the organ where gas exchange takes place. Hence, the entire cardiac output needs to go to the lungs. However, with right heart obstruction, this is not feasible unless there is a PDA. If not diagnosed prenatally or early after birth, these infants could develop severe cyanosis due to decreased, effective pulmonary blood flow. Hence, they require prostaglandin infusion to keep ductal patency. The common cyanotic heart diseases are listed in Table 5-1. We will now discuss each heart disease in detail.
The classic example of cyanotic patients with pulmonic stenosis is tetralogy of Fallot (TOF). It is the most common cyanotic CHD. TOF occurs in 7% of all CHDs.6 Anatomically, TOF consists of:
- VSD
- Pulmonic stenosis or pulmonary atresia
- Overriding aorta
- Right ventricular hypertrophy
Physiologically, pulmonic stenosis causes concentric right ventricular hypertrophy without cardiac enlargement. Once the right and left ventricular pressures have become identical, increasing severity of pulmonic stenosis reduces the flow of blood into the pulmonary artery and increases the right-to-left shunt. The VSD is silent, but the pulmonic stenosis produces a systolic ejection murmur. The severity of cyanosis is directly proportional to the severity of pulmonic stenosis, but the intensity of the systolic murmur is inversely related to the severity of pulmonic stenosis. The P2 component of S2 is soft and generally inaudible.
Neonates and infants with TOF may develop hypoxic spells. Hypoxic or hypercyanotic spells are also commonly referred to as “Tet spells.” Tet spells occur predominantly after waking up or following exertion.7 They are characterized by a period of uncontrollable crying, rapid and deep breathing (hyperpnea), deepening of cyanosis, limpness or convulsions, and occasionally death. Events such as crying lower the SVR, increase the right-to-left shunt, and initiate the spell by establishing a vicious cycle of hypoxic spells. The fall in arterial Po2, in addition to an increase in Pco2 and a fall in pH, stimulates the respiratory center and produces hyperpnea, which increases the negative intrathoracic pressure and increases the systemic venous return to the RV.
Patients with TOF may become symptomatic anytime after birth. The degree of obstruction determines the severity of cyanosis. Electrocardiogram (ECG) in TOF shows a right axis deviation with RV hypertrophy. The chest radiograph shows a normal-sized heart with upturned apex suggestive of RV hypertrophy (Figure 5-3). The absence of main pulmonary artery segment gives it the shape described as “coeur-en-sabot” or boot-shaped heart. The pulmonary fields are oligemic.
Figure 5-3

Chest radiograph from a newborn with tetralogy of Fallot. It shows a normal-sized heart with upturned apex suggestive of right ventricular hypertrophy. The absence of main pulmonary artery segment gives it the shape described as ”coeur-en-sabot” or boot-shaped heart. The pulmonary fields are oligemic.
Neonates with TOF should be monitored in the neonatal intensive care unit. The degree of cyanosis is determined by the degree of pulmonary blood flow. PGE1 infusion is generally not required. However, as the duct starts occluding, the extent of RV outflow obstruction becomes apparent. Severely cyanotic infants need to be started on PGE1 infusion to maintain ductal patency for adequate pulmonary blood flow.
Hypoxic spells of TOF require immediate recognition and appropriate treatment, because they can cause serious central nervous system complications. Treatment of hypoxic spell strives to break the vicious cycle. The infant should be picked up and held in a knee–chest position. Morphine sulfate 0.2 mg/kg subcutaneously or intramuscularly suppresses the respiratory center and abolishes hyperpnea. Alternatively, ketamine 2 mg/kg intravenously (IV) works by increasing the SVR and sedates the infant. Oxygen can be administered, but it has little demonstrable effect on arterial oxygen saturation. Acidosis stimulates the respiratory center causing hyperpnea. Hence, it should be aggressively corrected. Vasoconstrictors, such as phenylephrine 0.02 mg/kg IV, increase SVR and may break the spell. β-Blockers such as propranolol (0.1 mg/kg) or esmolol administered IV reduce the heart rate and may reverse the spell.
Neonates with TOF who are duct dependent or having hypercyanotic spells require some form of intervention before full repair. Balloon dilatation/stent implantation of the RV outflow tract and pulmonary valve, although not widely practiced, has been attempted to delay repair for several months.8,9 Stent implantation of the ductus arteriosus has also been attempted to allow adequate pulmonary blood flow. Stenting of the ductus arteriosus allows for discontinuation of PGE1 and discharge from the intensive care unit (ICU). This will buy time for nutrition and growth and ultimately a complete repair.
Surgical palliative shunt procedures may be warranted in the neonate for similar indications (eg, TOF with pulmonary atresia, TOF with severely hypoplastic pulmonary artery, severely cyanotic neonate, or medically unmanageable hypoxic spells). Typically a Gore-Tex interposition shunt is placed between the subclavian artery and the ipsilateral pulmonary artery via a lateral thoracotomy (Figure 5-4A). The operative mortality rate is 1% or less.
Figure 5-4

A. Picture illustrating a modified right Blalock-Taussig shunt. It involves placement of an extended polytetrafluoroethylene shunt between the innominate artery and the right pulmonary artery. B. Picture illustrating a complete repair of tetralogy of Fallot with ventricular septal defect patch closure, resection of right ventricle muscle bundle, and a transannular patch. (Reproduced, with permission, from McDonald RW. Ped US Today. 2008;13:161-228. Copyright © Chrestomathis Press Inc., All rights reserved.)
Total repair of TOF is carried out under cardiopulmonary bypass and circulatory arrest. The procedure includes patch closure of the VSD, resection of infundibular tissue, and placement of a transannular patch (Figure 5-4B). The mortality rate is 2% to 3%.10-12
Most patients can be extubated in the operating room or soon after surgery. Bleeding could be a problem during the postoperative period especially in polycythemic patients. Right bundle-branch block caused by the right ventriculotomy occurs in over 90% of patients and is well tolerated. Complete heart block occurs in less than 1% of patients.10-12 Junctional ectopic tachycardia and ventricular arrhythmia occur in 2% to 5% of postoperative patients.10-12
Pulmonary atresia with intact ventricular septum (PAIVS) is a rare CHD, accounting for 1% of all CHDs. The pulmonary valve is atretic and is replaced by a membrane. However, the pulmonary artery trunk and branch pulmonary arteries are usually well developed. The size of the tricuspid valve and the RV varies and relates to survival.13,14 When all 3 portions of the RV are present, the tricuspid valve and the RV are almost of normal size. This is called the tripartite type of pulmonary atresia, because all portions of the RV (the inlet, tubercular, and infundibular portions) are present. In this chapter, we discuss only this variant of PAIVS. Because there is no outlet to the right heart, an interatrial communication (ASD or patent foramen ovale [PFO]) is obligatory. The mixed blood from the left heart is pumped out to the aorta, and a PDA is necessary for survival after birth.
The neonate is severely cyanotic and may have mild respiratory distress. The physical examination reveals a single second heart sound with a faint pansystolic murmur caused by tricuspid valve regurgitation. The chest radiograph demonstrates mild cardiomegaly with decreased pulmonary vascular markings. The ECG demonstrates a normal axis with decreased right-sided forces. Echocardiography is diagnostic. It will reveal the atretic, nonmobile pulmonary valve, with no flow of blood across it. The RV cavity is usually smaller than normal and hypertrophied. There is right-to-left shunt across the interatrial communication and left-to-right shunt across the PDA.
Neonates with PAIVS are dependent on a PDA for pulmonary circulation. Hence, PGE1 infusion should be started right after birth if there was a prenatal diagnosis or immediately after the diagnosis is suspected or confirmed. Neonates with PAIVS usually require a catheter or surgical intervention soon after birth. Hence, they are typically not fed until then.
Routine cardiac catheterization and angiocardiography are undertaken in almost all neonates born with PAIVS. Angiocardiography will demonstrate the presence or absence of coronary sinusoids15 from the RV and whether the coronary flow is dependent on the RV (Figure 5-5).
The determination of either the presence or absence of RV-dependent coronary sinusoids15 is critical in the management of these patients. If the RV, pulmonary artery, and tricuspid valve are determined to be of adequate size that will allow a future biventricular repair and if there are no RV-dependent coronary sinusoids, then radiofrequency-assisted valvotomy and balloon dilatation can be performed (Figure 5-6).
Figure 5-6

A. Injection of contrast in the right ventricular outflow tract shows no communication to the pulmonary artery. There is a plate-like atresia of the pulmonary valve. B. Radiofrequency (RF) perforation of the pulmonary valve. The tip of the RF wire can be seen to cross through the atretic pulmonary valve into the main pulmonary artery. C. Balloon valvuloplasty of the pulmonary valve over a wire that has now been passed across the perforation made in the pulmonary valve. D. Right ventriculogram after intervention shows contrast to fill the main pulmonary artery through the pulmonary valve.
There is usually a plate-like membrane that separates the RV from the pulmonary artery.16 A radiofrequency tipped wire is used to perforate through this membrane followed by balloon dilatation of this valvotomy site to establish patency between the RV outflow tract to the pulmonary artery. After this procedure, the baby is taken back to the ICU and PGE1 is discontinued. In approximately 50% of those who undergo this procedure, there is no need for further palliation at this point.17,18 The other 50% of patients still do not have adequate pulmonary blood flow and need further palliation to establish pulmonary blood flow. One such palliation could be stenting of the PDA in the catheterization lab.
There are several surgical palliative procedures that are available for neonates with an adequately sized tricuspid valve and RV. One approach would be to perform a closed surgical valvotomy without cardiopulmonary bypass plus a modified Blalock-Taussig shunt (Figure 5-4A). Another approach involves placement of a transannular RV outflow patch (Figure 5-4B) and a Blalock-Taussig shunt under cardiopulmonary bypass. These procedures are palliative, allowing the neonate to grow after being discharged from the hospital. They will require a definitive biventricular repair in the future.17,18
When the RV or tricuspid valve is small and will not allow for a future biventricular repair, then a Blalock-Taussig shunt without the RV outflow patch is performed. A Fontan operation is performed at a later time. Postoperative critical care management of neonates after a palliative surgical procedure is similar to that described for TOF.
Dextro-transposition of the great arteries (d-TGA) is defined as the aorta arising from the RV and the pulmonary artery arising from the LV. It account for about 5% of all CHD. It is more prevalent in males than females (3:1).19
In complete d-TGA, the aorta lays anterior and to the right of the pulmonary artery and carries desaturated blood from the RV to the body, and the pulmonary artery arises posteriorly from the LV and carries oxygenated blood to the lungs (Figure 5-7).
Figure 5-7

Diagram representing dextro-transposition of the great arteries showing the aorta arising from the right ventricle and the pulmonary artery arising from the left ventricle. (Reproduced, with permission, from Fyler DC, ed. Nadas’ Pediatric Cardiology. Philadelphia, PA: Hanley & Belfus; 1992.)
Because the systemic and pulmonary circulations are separate, survival depends on the presence of atrial, ventricular, or aortopulmonary (PDA) communications. Complete d-TGA is classified as (1) with intact ventricular septum or (2) with VSD. The latter group is further subdivided into cases with or without pulmonic stenosis. The physiology of d-TGA with VSD and pulmonic stenosis is similar to TOF.20 In this section, we will only discuss patients with TGA with intact ventricular septum.
In patients with d-TGA, the oxygenated pulmonary venous blood recirculates in the lungs, whereas the systemic venous blood recirculates in the systemic circulation.19 Survival depends on the mixing available between the 2 circulations. In patients with intact ventricular septum, the mixing site is the atrial communication. Generally, the atrial communication is the PFO, and being small, the mixing is poor. The neonates will become symptomatic due to severe hypoxemia and systemic acidosis. As the PVR falls, the PDA shunts from aorta to pulmonary artery and does not serve as a site for mixing. The extra pulmonary blood flow across the PDA returns to the left atrium and shunts across the PFO to mix at the atrial level provided the atrial communication is unrestrictive.19 Hence, it is generally a good idea to keep the PDA open with infusion of PGE1.
Neonates with d-TGA are cyanotic at birth. If undiagnosed, the neonate presents with rapid breathing and congestive heart failure within the first week of life. If the interatrial communication results in poor mixing, the neonate continues to remain severely cyanotic. Physical examination shows severe cyanosis with or without signs of congestive heart failure, normal first heart sound, single loud second heart sound, and insignificant grade 1 to 2 ejection systolic murmur. The ECG shows right axis deviation and RV hypertrophy. The chest radiograph shows cardiomegaly with a narrow base and plethoric lung fields. The cardiac silhouette has an “egg on side” appearance (Figure 5-8).
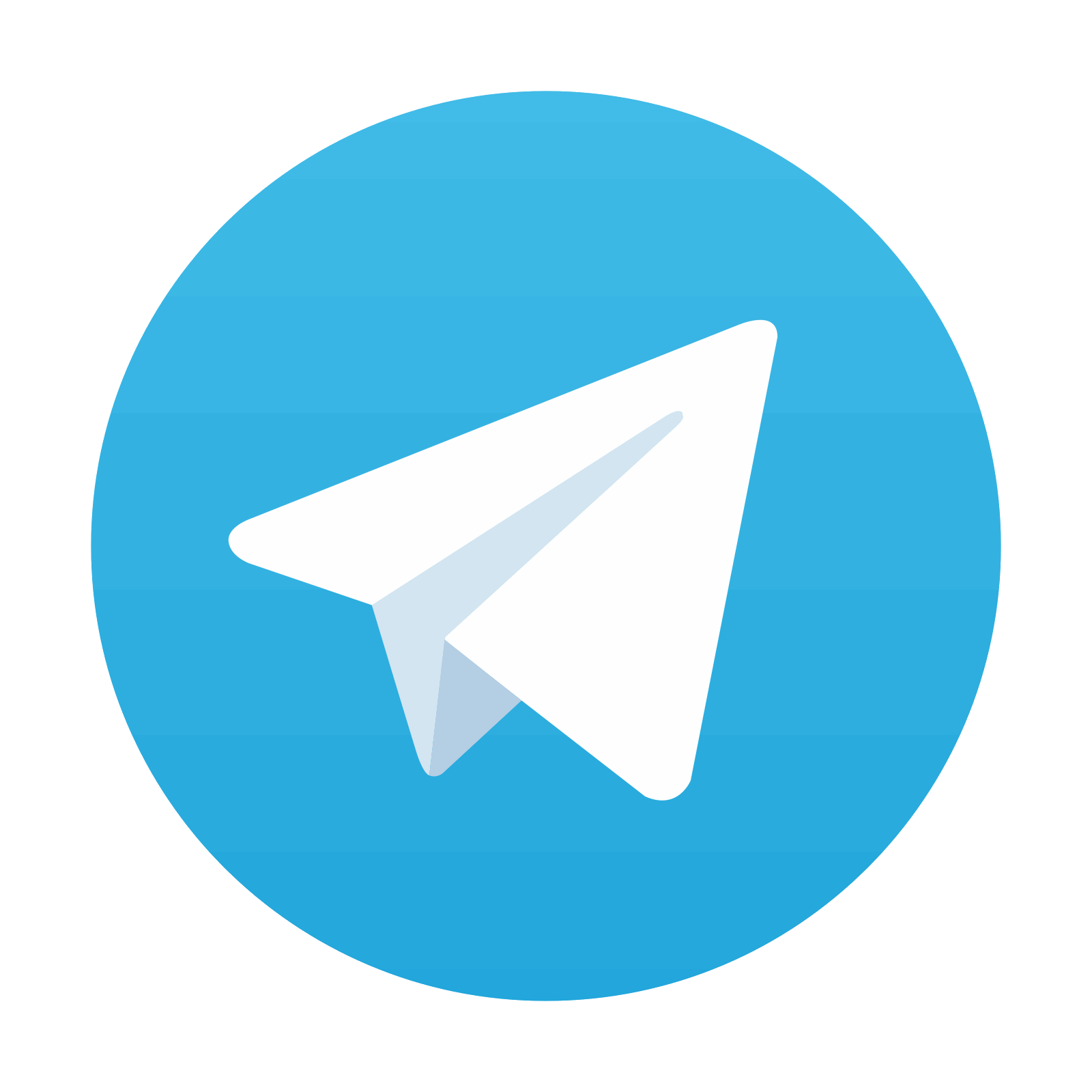
Stay updated, free articles. Join our Telegram channel

Full access? Get Clinical Tree
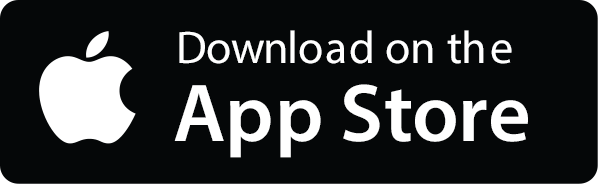
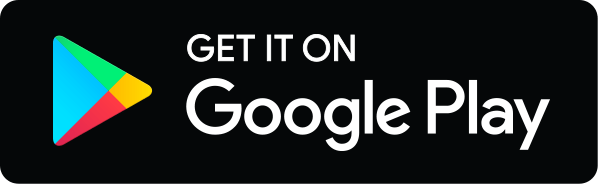