Fig. 7.1
Specification of cardiac asymmetry. (a) Cilia generated leftward flow at the embryonic node initiate an asymmetric signaling cascade in which nodal, lefty2, and pitx2 are expressed exclusively in the left lateral plate mesoderm. This left-sided expression has important consequences for heart looping, a process in which the initially symmetric heart tube undergoes dextral looping to form the four-chambered heart positioned on the left side of the thoracic cavity. (b) Normal intracardiac anatomy in the newborn mouse heart visualized by histopathology examination using episcopic confocal microscopy reveals the arrangement of four cardiac chambers (RV/LV, LA/RA) with two great arteries (Ao/PA), with the aorta (Ao) seen arising from the left ventricle (LV) [45]. (c) Histopathology examination of a newborn heart at a different episcopic confocal microscopy imaging plane showed the normal intracardiac anatomy of the interatrial septum separating the right and left atria and the interventricular septum partitioning the left and right ventricles. Abbreviations: V ventricle, A atria, LA left atrium, RA right atrium, LV left ventricle, RV right ventricle, Ao aorta, PA pulmonary artery
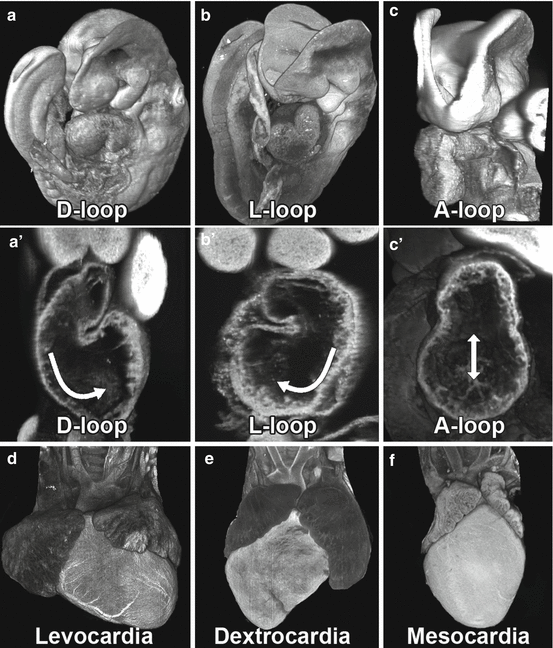
Fig. 7.2
Heart looping and heart situs. The linear heart tube undergoes dextral looping (a, a’) yielding levocardia with heart apex pointing to the left and positioned on the left side of the chest cavity (d). Defects in left-right patterning can perturb heart looping, resulting in reversed looping (L-loop); b, b’ with heart apex pointing to the right, or dextrocardia (e), or abnormal looping (A-loop, c, c’), with midline cardiac situs, or mesocardia (f). 2D images and 3D reconstructions were obtained by episcopic confocal microscopy. Arrows specify direction of heart rotation [45]
7.3 Complex Congenital Heart Disease and Heterotaxy
The finding of complex congenital heart disease in patients or mice with heterotaxy is well described. Mice are readily suited for modeling human congenital heart disease; as being air-breathing animals, they have the identical four-chambered cardiac anatomy as humans [3]. Some examples of congenital heart defects found in mutant mouse models with heterotaxy are shown in Fig. 7.3. Compared to normal levocardia (Fig. 7.3a), mice with laterality defects can exhibit mirror-image dextrocardia, which is the complete reversal of normal heart structure (Fig. 7.3b). Aberrant left-right patterning also can lead to more complex phenotypes, such as ventricular dextroversion in which the heart exhibits dextrocardia, but the ventricular chamber placement and outflow tract alignment are not altered (Fig. 7.3c). Other examples of phenotypes associated with abnormal left-right patterning include transposition of the great arteries (TGA), a condition in which the connections between the ventricles and the outflow tracts are reversed (Fig. 7.3d), double-outlet right ventricle (DORV) in which both outflow tracts originate from the right ventricle (Fig. 7.3e), and atrioventricular septal defect (AVSD) with complete failure of the atrioventricular septum, resulting in a hole that allows communication between all four cardiac chambers.
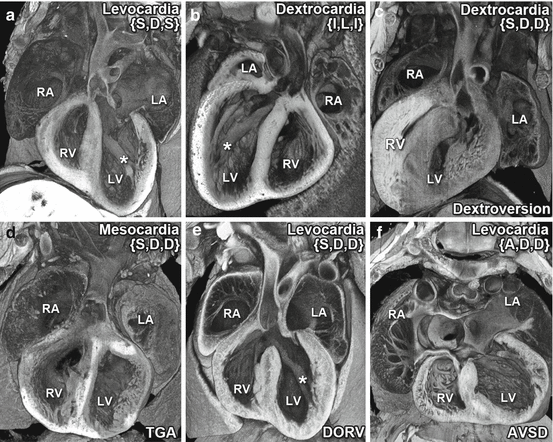
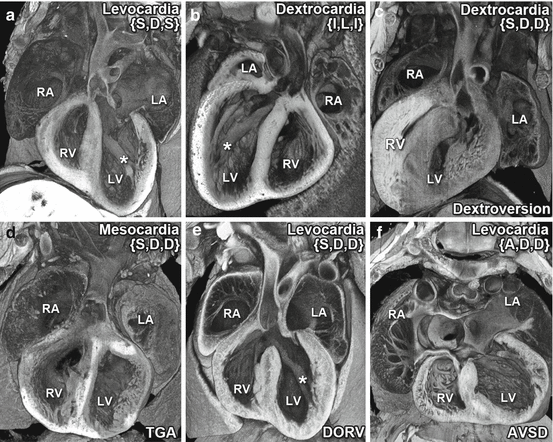
Fig. 7.3
Congenital heart defects associated with left-right patterning defects. Complex congenital heart defects are often found in conjunction with heterotaxy. Shown are five (b–f) examples of structural heart defects observed in mouse mutants with heterotaxy as compared to the normal cardiac anatomy of a wild-type mouse (a). In the wild-type heart (a), the heart apex points to the left, indicating levocardia, and the two atria and two ventricles are in their normal left-right position. Also seen are the papillary muscles attached to the free wall in the LV and the aorta emerging from the LV. The mutant heart shown in (b) exhibits mirror-image dextrocardia with the apex pointing to the right. Thus the four chambers and the intracardiac anatomy are entirely normal but are mirror symmetric in orientation. The mutant heart shown in (c) also exhibits dextrocardia but is said to have dextroversion, as the morphologic right and left ventricles/atria remain in the anatomic right and left, respectively, with correct outflow tract orientation. Other complex congenital heart defects commonly observed with heterotaxy are transposition of the great arteries (TGA), in which the connections between the ventricles and the outflow tracts are reversed (d), double-outlet right ventricle (DORV), in which both outflow tracts originate from the RV, or atrioventricular septal defect (AVSD), in which the atrial and ventricular septum fail to form, giving rise to abnormal common atrioventricular valves (f). Note that the heart exhibiting TGA also is characterized by abnormal midline positioning or mesocardia (d). For each heart, the Van Praagh segmental classification is indicated in brackets {} to denote the situs of the atria, direction of heart looping, and relations of the outflow tract (S solitus, I inverted, D dextral looping, A ambiguous). Normal cardiac situs is denoted by {S,D,S}, while mirror symmetric situs is indicated by {I,L,I}. The * denotes papillary muscle attached to the free wall, a characteristic of the left ventricle. 3D reconstructions were generated from serial 2D image stacks obtained by episcopic confocal microscopy [45]
7.4 Modular Assembly of Cardiac Asymmetry
While surgical palliation now allows many patients with congenital heart disease to survive their initial acute cardiovascular deficits, even those with complex structural heart defects, there remains high morbidity and mortality in these patients. To track and study the emergence of cardiac asymmetry and its disturbance, Van Praagh developed the “segmental” approach for the classification of cardiac asymmetry [4]. This approach utilizes a three-part lettering system to describe: (1) the atrial situs, (2) the direction of heart looping, and (3) the relationship of the great arteries [5]. In this manner, atrioventricular and inflow/outflow tract identities can be summarized and tabulated across a patient cohort. Thus normal cardiac situs would be described as (S,D,S) – solitus (S) or normal atria situs, normal dextral (D) looping of the heart, and solitus (S) or normal arrangement of the great arteries. What is striking with regard to patterning of cardiac asymmetry captured by the Van Praagh classification scheme is that left-right patterning of the different segments of the heart, i.e., the atria, ventricle, and outflow tract, can be specified in a modular fashion independent of each other. Thus it is possible to have a heart with two left or two right atria (referred to as left or right atrial isomerism), or a left atrium connected to a right ventricle, but on the body’s right or left side. It also is possible to have the pulmonary artery connected to the left ventricle and the aorta to the right ventricle, i.e., transposition of the great arteries, and this can occur with the ventricles positioned in their normal left-right position (d-TGA) or in an inverted orientation (l-TGA). Such complex shuffling of cardiac situs can cause not only mixing of oxygenated with deoxygenated blood, but in some instances, blood flow is routed in two parallel circuits such that the systemic blood flow bypasses the lung altogether. These findings suggest that understanding how left-right asymmetry is established during cardiac morphogenesis is of great importance for elucidating the etiology of congenital heart disease.
7.5 Left-Right Patterning
Patterning of left-right asymmetry is a developmental process highly conserved in evolution. In the vertebrate embryo, the initial break in symmetry occurs via signaling mediated by a transient, ciliated organ, known as the embryonic node in mouse embryos. At the embryonic node, monociliated pit cells have rotating motile cilia and are surrounded by perinodal crown cells harboring nonmotile primary cilia [6, 7]. Motile cilia of the pit cells rotate clockwise, generating a leftward flow, resulting in propagation of a signal that establishes the left-right axis through asymmetric expression of nodal, a transforming growth factor (Tgf) ligand, on the left side, and its inhibitor DAN domain family member 5, BMP antagonist (Dand5) on the right side of the embryonic node [8]. Further studies investigating how the leftward nodal flow is perceived and propagated have led to three distinct models: (1) an accumulation of morphogens on the left side of the embryo, (2) an asymmetric distribution of node vesicular parcels containing hedgehog proteins and retinoic acid, or (3) detection of fluid flow by the nonmotile cilia of the crown cells leading to asymmetric calcium signaling via polycystic kidney disease (Pkd) 2 and the Pkd1-related locus Pkd1l1 [9–13]. Most intriguing is recent evidence from our laboratory resulting from analysis of a novel dynein, axonemal, heavy chain 5 (Dnah5) mutant, indicating that nodal flow may be necessary but not sufficient for establishing the left-right axis.
7.6 Heart Looping and Development of Cardiac Asymmetry
Cardiac morphogenesis begins with migration of cells comprising the primary heart field in the cardiac crescent to the axial midline, forming a hollow linear heart tube. This is followed by a distinct rightward or dextral (D) looping of the heart tube, the first evidence of cardiac asymmetry. Defects in the specification of left-right patterning can perturb heart looping, causing reversed looping (L-loop) or no looping (A-loop) which can result in dextrocardia (L-loop) or mesocardia (A-loop), respectively (Fig. 7.2) [14]. The process of heart looping also serves to initiate cardiac chamber formation such that the initially posteriorly positioned atrial segment is brought to a cranial position relative to the primitive ventricles, establishing the anterior or superior positioning of the atrial segment of the heart tube relative to the ventricle as seen in the mature heart (Fig. 7.1) [15]. While the primitive heart tube is formed by cells from the primary heart field, these cells will only give rise to the future left ventricle, while most of the remainder of the heart, the right ventricle, outflow tract, and atria, are derived from another cardiac progenitor cell population, the second heart field cells [16, 17]. These cells migrate into the looped heart tube via the dorsal mesenchyme protrusion and are added onto the arterial and venous pole of the heart over a period of several days during embryogenesis [18]. Second heart field cells play an important role in elaboration of the four-chambered heart and contribute cells that form the atrial septum [19].
Studies using zebrafish, a model organism which breaks symmetry via a ciliated organ functionally homologous to the mouse embryonic node, the Kupffer’s vesicle (KV), have helped to elucidate the connection between early asymmetries in gene expression and heart looping [20]. While the zebrafish has only a two-chambered heart, it still undergoes cardiac looping and exhibits cardiac and visceral organ asymmetry. The transparency of the zebrafish embryo and its development externally has made it possible to study cardiac looping at the single-cell level. Such studies have indicated that activation of nodal signaling in the left lateral plate mesoderm (LPM) may direct heart looping by modulating directional migration of cardiac progenitor cells [21]. This is mediated through left-sided inhibition of bone morphogenetic protein (BMP) signaling by a nodal-regulated extracellular matrix protein, Has-2. As a result, a left-right difference in cell motility emerges in the zebrafish embryo, with the left side of the embryo exhibiting increased cell motility driving asymmetric looping of the heart tube [22]. Whether the same mechanism is conserved in the regulation of heart looping in the mouse embryo is yet to be determined.
7.7 Developmental Signaling in the Specification of Cardiac Asymmetry
The specification of cardiac asymmetry occurs during early embryogenesis, mediated by the same developmental processes that specify the entire left-right body axis. While the initial breaking of symmetry occurs at the embryonic node, signaling initiated at the node must be propagated to the LPM, resulting in left-sided LPM expression of Nodal, followed by left-sided expression of left-right determination factors Lefty1/Lefty2 (nodal inhibitors), and then left-sided expression of Pitx2, a paired-like homeodomain transcription factor [7]. This asymmetric gene expression cascade is essential for left-right organization of the entire embryo, with left-sided Pitx2 expression persisting in all of the visceral organ primordia throughout development. This is thought to regulate a gene expression program specifying left-sided differentiation (Fig. 7.1) [7, 23]. Since cells of the first and second heart field are found in both the left and right lateral plate mesoderm, it is likely that the early LPM asymmetric gene expression pattern may drive cardiac asymmetry and the coding of left-right patterning in the first and second heart field cells [24]. Consistent with this, Pitx2 expression persists exclusively on the left side of the developing heart, impacting patterning of the second heart field, which originates closer to the embryonic midline than the first heart field [25]. Expression of Pitx2 is also required later in development for formation of the aorta and pulmonary artery from the pharyngeal arches [26]. In addition, Pitx2 has been shown to regulate cell proliferation and cell migration, which may also impact heart looping and contribute to specification of cardiac left-right asymmetries (Fig. 7.1) [27].
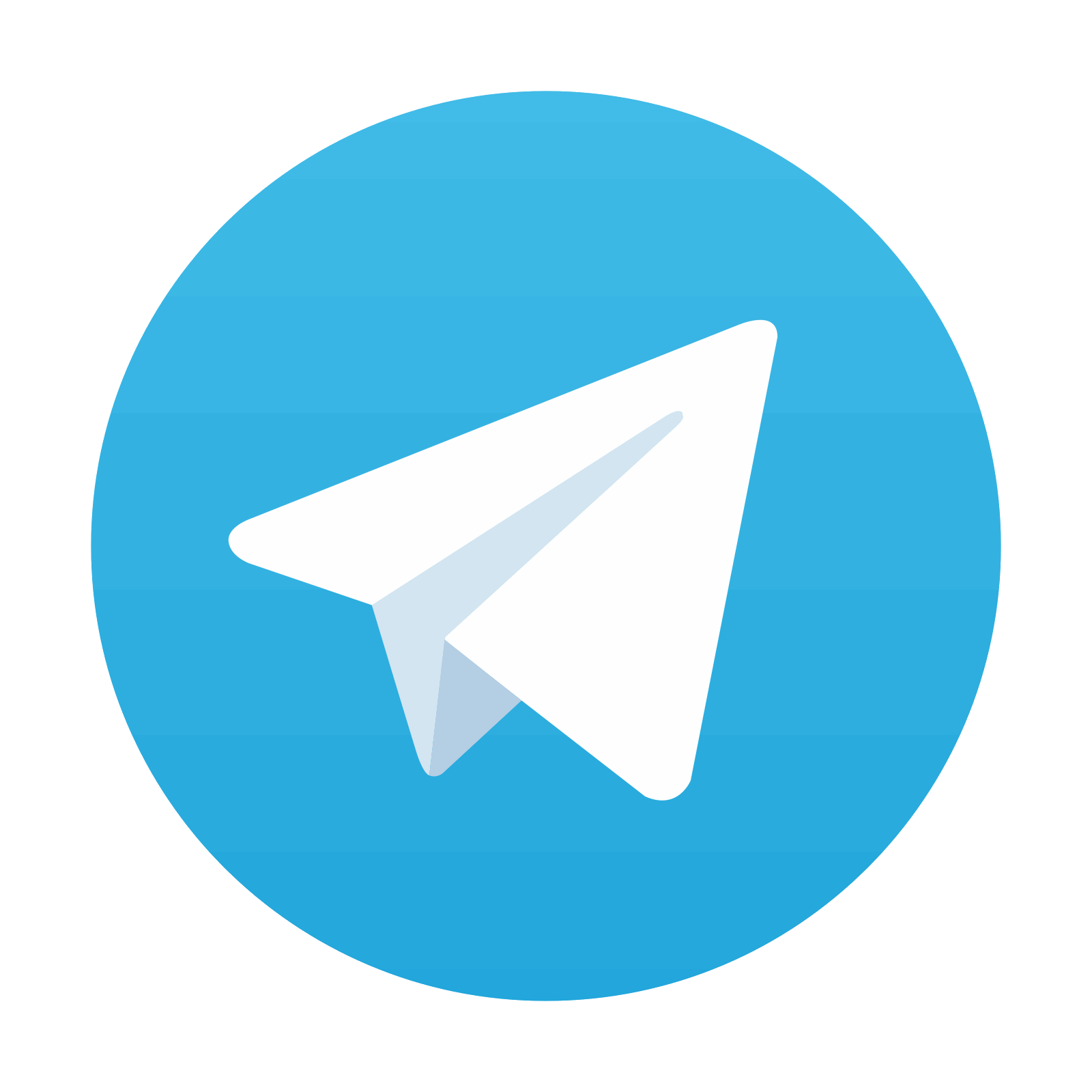
Stay updated, free articles. Join our Telegram channel

Full access? Get Clinical Tree
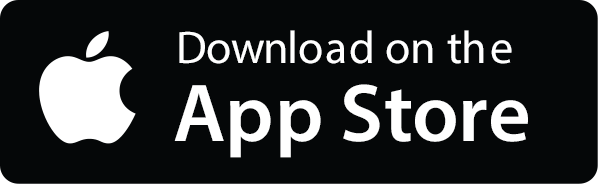
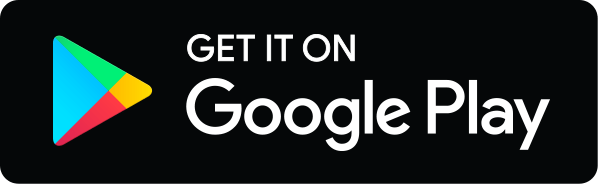