Epidemiology
Introduction
The clinical features of infective endocarditis were first described in the 16th century by Jean François Fernel, a French physician who first introduced the term ‘physiology’ to describe the study of the function of living things [ ]. Over the next few centuries, through astute observations of human physiology and pathophysiology, several preeminent figures laid the foundations of our fundamental knowledge of infective endocarditis, including the pathogenesis of microorganisms, the formation of valvular lesions, and the clinical sequelae of embolization [ ]. In 1885, Sir William Osler, of the ‘Osler’s node’ eponymous fame for this very disease, gave a series of landmark lectures that not only synthesized the large preceding body of knowledge into a coherent conceptual framework, but also brought endocarditis to the attention of the medical community [ ].
In the early 20th century, even as physician’s understanding of endocarditis continued to evolve, morbidity and mortality remained high in the absence of effective treatments. Early regimens saw the use of sulfonamides, poisons such as mercury and arsenic, and hyperthermia, and cure rates were expectedly nonexistent [ , ]. Fortunately, the 20th century also saw parallel advances in the field of microbiology and biochemistry. The discovery of penicillin by Sir Alexander Fleming in 1928, and later the first successful clinical trials and refinement of mass production techniques by Howard Florey, Ernst Chain, and Norman Heatley in the 1940’s finally provided physicians with hopes of a cure.
Penicillin drastically changed the natural course of the disease as it was highly effective against the wild-type Staphylococcus and Streptococcus bacterial strains that had not yet experienced artificial selective pressure. As this medical miracle saw use even beyond endocarditis, it was befitting that Sir Alexander Fleming, after having received the 1945 Nobel Prize in Physiology or Medicine for its discovery, forewarned the emergence of resistance [ ]. Indeed, within mere years after widespread use physicians began to see resistant Staphylococcus aureus among other resistant organisms, and thus began an evolutionary arms race that continues to this day [ ].
Penicillin also marked the beginning of an ever-changing landscape of infectious endocarditis. Medical advances through the rest of the 20th century and into the early 21st century include the development of novel antibiotics, use of cardiovascular implantable electronic devices (CIEDs) and prosthetic valves, the ubiquitous use of long-dwelling venous catheters, and a growing hemodialysis population—all of which have impacted clinical practice and management [ ]. In addition, the growing opioid epidemic in America has affected both the prevalence and incidence of right-sided endocarditis and introduced rare organisms into the microbiology [ , ]. Together, all these changes have influenced the epidemiology of endocarditis considerably.
General epidemiology
Infectious endocarditis remains a rare disease, with an incidence of 2–10 cases per 100,000 people, and varies according to world regions, country, and even different areas within a nation’s borders [ ]. In countries with consistent reporting, the overall incidence appears to be at least stable, if not slightly increasing [ , ]. Despite these statistics, the epidemiology has changed significantly over the years. Infectious endocarditis in the preantibiotic era predominantly affected those in their 30 to 40s, often in the setting of rheumatic valvulopathy, and with Streptococcus spp. as the predominant pathogen [ , ]. However, beginning in the 20th century, as the nations of the world began to diverge in terms of industrialization and wealth, so too have the features of infective endocarditis between low- and high-income countries. Low-income countries continue to retain their preantibiotic era features, with rheumatic disease remaining a key risk factor, affecting up to two-thirds of cases [ ]. High-income countries, on the other hand, have seen rheumatic disease fall to the wayside in the setting of improved living conditions and readily available treatment for Streptococcus pharyngitis [ , ]. In its stead, it has been replaced by an older population, with a different set of risk factors, and a changing microorganism profile [ ].
The average age of infective endocarditis in high-income countries in the early 21st century has now risen to the 60 and 70s, with a relatively even split between genders [ , , , ]. This shift in age is a direct reflection of an increased life expectancy, with age-related comorbidities, and the procedures necessary for their management [ , , ]. General age-related risk factors include hypertension, diabetes, coronary artery disease, and kidney and liver disease [ , ]. Because of the increasing end-stage renal disease (ESRD) population, hemodialysis is now one of the biggest risk factors for infectious endocarditis, accounting upward of 25% of infective endocarditis cases surveyed [ , ]. Valvulopathies, both congenital such as bicuspid aortic valves, and age-related such as regurgitation and prolapse, also convey risk [ ]. The affected valves are predominantly left-sided, most commonly the aortic valve, with right-sided infective endocarditis being much rarer at around 5%–10% [ , ]. Unfortunately, repair of these various valvular diseases with prosthetic devices only serve to further increase the risk, accounting upward of 20% of cases [ , , , ]. Similarly, the rising prevalence of CIEDs for the treatment and management of various cardiovascular diseases has also been accompanied by a concomitant rise in device-related infective endocarditis [ , ].
The microbiology of infective endocarditis in high-income countries has also changed as a direct consequence of medical advancement. Prior to 1960 and 1970s, viridans group streptococci accounted for the majority of cases [ ]. However in the last few decades, staphylococcal species have now supplanted viridans streptococci, now accounting for up to 40% of cases, with S. aureus as the predominant organism [ , , , ]. This change in pattern is attributed to a growing immunocompromised population such as those on long-term steroids and other immunosuppressive medications, and those with solid organ or bone marrow transplants [ ]. This change also parallels a rise in the use of long-term dwelling catheters, increase in invasive procedures such as transcatheter aortic valve replacement (TAVR), and the ubiquity of prosthetic devices including prosthetic valves and CIEDs [ , , , ]. For similar reasons, there has also been an increase in Enterococcus spp., β-hemolytic Streptococcus spp., and nutritionally variant Streptococci [ , ]. The percentage makeup of rare organisms such as gram-negatives, fungus, and atypical bacteria remain low [ , , , ].
Infective endocarditis in injection drug users
Infective endocarditis in intravenous drug users comprises a unique epidemiologic group. The population is younger with a median age of 40, and affects more males (55%–58%) than females [ , , ]. The annual rate varies from 1.5 to 20 per 1000 users, representing a 20x higher rate of risk than the general population [ , ]. Cases have risen alongside the growing opioid epidemic, reaching upward of one-third of all infective endocarditis cases in certain tertiary care centers [ ]. In contrast to nonusers, infective endocarditis in injection drug users is predominantly right-sided (76%–79%), with a smaller proportion affecting left-sided valves (16%–30%) or involving both sides (5%–10%) [ ]. Of the affected right-sided valves, there is a preference for the tricuspid valve, upward of 69% [ , ]. This predilection for right-sided valves is believed to be due to direct injection of chemical substances and microorganisms into the venous system, causing both caustic and physical damage to the valvular tissue leading to subsequent infectious seeding [ , ]. There is also data to suggest that certain vasoactive drugs, particularly cocaine, may cause vascular vasospasm of the valvular intima, triggering local injury and thrombus formation to act as a nidus for infection [ ]. This mechanism may also explain why noninjection substance users have a higher risk of infective endocarditis [ ].
Similar to nonusers, S. aureus is the most common organism, but at a significantly much higher percentage, with one comparison study showing 68% in injection drug users compared to 28% in nonusers [ , ]. This stark difference is felt to be due to increased rates of S. aureus colonization [ , ]. Following Staphylococcus , Streptococci spp. and Enterococcu s spp. are the next most common pathogens [ ]. What was statistically ‘rare’ in the nonuser population were more common in the setting of injection drug use, with higher rates of gram-negatives such as Pseudomonas , fungal pathogens (especially Candida spp.), and polymicrobial infections [ , , ]. In addition, because of habits associated with injection drug use and associated paraphernalia such as oral preparation of the needle and of the injection site, HACEK organisms and other oral flora are also not uncommon [ ].
Morbidity and mortality
Infective endocarditis has substantial morbidity and mortality. Several factors influence the overall prognosis including the specific pathogen(s) involved, presence of embolic phenomenon and infectious complications, preexisting comorbidities, degree of valvular involvement, and necessity of surgical intervention [ ]. Depending on the clinical situation, initial in-hospital mortality can range anywhere from single-digits to as high as 45% [ , , ]. Poor prognostic factors include left-sided or paravalvular involvement, prosthetic valve endocarditis (PVE), presence of multiple comorbidities, CNS involvement, advanced age, and Staphylococcus or fungal infections [ , ]. Even with modern medicine, prognosis continues to remain poor, with 1-year mortality as high as 40% [ , , , ]. In one study using mathematical modeling, those with infective endocarditis continue to have lower survival rates compared to that expected of the general population at 1 year (90% infective endocarditis (IE) vs. 92% non-IE), 3 years (81% IE vs. 86% non-IE), and 5 years (70% IE vs. 82% non-IE), demonstrating that IE continues to convey excess morbidity and mortality for years after the initial diagnosis [ ].
Clinical outcomes for those with Intravenous drug use (IVDU) also have high morbidity and mortality. One comparison study between users and nonusers show comparable initial in-hospital mortality (6% IVDU vs. 9% non-IVDU, P > .05) and 1-year all-cause mortality (16% IVDU vs. 13% non-IVDU, P > .05) [ ]. Another comparison study showed that while IVDU had better survival in initial in-hospital mortality (6.8% IVDU vs. 9.6% non-IVDU, P < .001) and 30-day readmission mortality (3.4% IVDU vs. 7.9% non-IVDU, P < .001), mortality at later readmissions between 30 days and 180 days was similar (4% IVDU vs. 3.8% non-IVDU, P > .05) [ ]. Long-term prognosis for IVDU is similarly poor, with longitudinal studies showing overall mortality reaching as high as 30%–40% [ , ]. These comparable survival rates for IVDU, despite higher risk behavior, can be attributed to a younger population with fewer preexisting comorbidities [ ]. Another potential explanation is the higher rate of right-sided valvular involvement in those with IVDU, as right-sided infective endocarditis has more favorable outcomes [ ]. On the contrary, left-sided infective endocarditis in IVDU is worse than nonusers [ ].
Because of the poor prognosis, there is interest in identifying root causes to improve clinical outcomes. It is known that prompt diagnosis, appropriate antibiotic administration, and early evaluation for surgical candidacy are crucial to improve survival [ , ]. Unfortunately, this does not always occur, with reports of delayed diagnoses, inappropriate antibiotic use, delays in surgical interventions, or simply not following or deviating from expert guidelines [ , ]. As such, multiple expert groups now recommend establishing a multidisciplinary team for the management of infectious endocarditis given the complexity of the disease [ , , ]. These ‘endocarditis teams’ have been shown to reduce both in-hospital and long-term mortality, decrease complications from infectious embolic phenomenon, a reduction in time to appropriate surgical intervention, and lower surgical mortality [ , ]. In fact, endocarditis teams have shown to be independent positive predictors of 1-year survival [ ].
Pathophysiology of infection
Introduction
The vascular endothelial lining has evolved to perform several vital functions including the regulation of vascular tone, exchange of molecules between blood and tissue compartments, immune system regulation, and homeostasis [ ]. Promotion of bacterial and fungal adhesion is absent from these functions. This is not an evolutionary omission but rather a protective mechanism. The bloodstream is considered a sterile body site [ ] but when microbes gain entry into this space, the endothelial lining functions as a protective barrier ( Fig. 1.1 ) .

In general, there are four critical factors that must be accomplished in successive order for the development and propagation of infective endocarditis. Those factors include the development of endothelial damage, pathogen access into the intravascular space, pathogen adherence to the endothelium, and pathogen proliferation [ ].
A variety of factors can lead to endothelial damage, including mechanical forces, autoantibodies, inflammatory injury, as well as direct insults from the pathogens themselves [ ]. The most important of these factors, pathogenic virulence will be discussed in further detail later in this book. Once the endothelial lining of the valve has suffered an insult, the next step of the process is merely a waiting game for a pathogen to gain entry into the bloodstream. Whether through mucosal breakage, hematogenous spread from another infected tissue, or direct inoculation of the bloodstream as in the case of intravenous drug use or an indwelling catheter, once an organism has entered the bloodstream, it has the potential to adhere to the damaged valve and initiate pathogenesis.
Pathogens can directly adhere to the damaged lining or they can adhere to a sterile platelet-fibrin thrombus, acting as an infectious nidus [ ]. Pathogens have varying affinity for adherence to the endothelial lining. S. aureus , for instance, has a very high affinity for adhesion, whereas most Enterobacterales (formerly known as Enterobacteriaceae) have a much lower affinity for adhesion. S. aureus is also unique in that it has the ability to directly infect intact endothelium, which contributes to its overall high rate of infectivity [ ].
With the pathogen attached to the valve, ongoing replication then allows for the development of vegetation, or a proliferative infectious mass. Individual pathogen virulence factors and the host immune system play vital roles in this process by promoting ongoing replication and survival [ ].
Overview of pathogens
A thorough understanding of the patient in front of you will often permit the clinician to predict the causative organism with relative degree of accuracy. There is a direct correlation between a patient’s exposure history and the patient’s microbiologic etiology of an infection. This association is quite strong with infective endocarditis. Gram-positive cocci including Staphylococcus spp. (skin), Streptococcus spp. (skin, mouth, gastrointestinal (GI) tract), and enterococci (GI and genitourinary (GU) tracts) each compromise a unique niche of normal human flora. Breaks in the skin and/or mucosal surfaces promote hematogenous inoculation. Once access to the bloodstream has been obtained, these organisms, to varying degrees, exhibit enhanced binding to damaged vasculature which increases their propensity to infect heart tissues. Conversely, gram-negative rods primarily colonize the respiratory, GI, and GU tracts and demonstrate decreased abilities to stick to cardiac tissue. However, disruptions of the GI and GU tracts can permit hematogenous dissemination of gram-negative rods (GNRs) with varying degrees of risk for infective endocarditis, depending on underlying patient- and pathogen-specific factors. The third important group of pathogens to be considered is yeast, specifically Candida spp. These fungi often colonize the GI tract and plan an important role in both nosocomial and PVE. More detail on the various pathogens associated with infective endocarditis will be explained in a subsequent chapter.
The microbe’s role in vegetation
As vegetation matures, it becomes a meshwork of fibrin, platelets, white and red blood cells, and interspersed conglomerates of bacteria. The vegetation develops into a complex ecosystem that functions to protect the bacterial organisms from the innate immune system as well as antimicrobials. Outer layers of fibrin function as a defense against phagocytic cell penetration. Bacteria deep within the vegetation experience reduced targeting from the immune system and exist in reduced metabolic states, which can provide added protection against antimicrobials which target rapidly dividing and metabolically active bacteria (i.e., beta-lactams) [ ]. The fibrin/cellular matrix can persist long after the infectious component of the vegetation has been eradicated, and in the absence of surgical extraction of the vegetation, it can take months or even years for the immune system to clear out the dead bacteria and microorganism debris from the vegetation [ ].
The role of valvular integrity in the development of infective endocarditis
In general, patients must progress through the above four steps in sequence in order for infective endocarditis to develop. If the host’s immune defenses are able to intervene before the completion of this process, infection cannot develop. However, it should be noted that the underlying integrity of the cardiac valves also plays a critical role in the development of pathogenesis, and their impairment can significantly increase the risk for subsequent infection. Transient bacteremia is a common phenomenon that can occur following the manipulation of a nonsterile body site [ ]. A representative example of this phenomenon is the brushing of one’s teeth. In a study of 290 subjects comparing brushing teeth with single tooth extraction with or without amoxicillin prophylaxis, all three scenarios were associated with transient bacteremia. The authors concluded that while the overall incidence was lowest for the tooth-brushing group, the frequency at which patients perform this activity on a daily basis likely makes this scenario the highest overall risk for the development of endocarditis [ ]. Why then, do we all not develop endocarditis on a far more frequent basis given the relatively high frequency of brushing, assuming one performs regular oral hygiene? The answer appears to lie in the preservation of valvular integrity, and so long as this remains, the risk for overall infection remains low. However, a number of structural conditions can increase the risk for the development of infective endocarditis by impairing the integrity of the cardiac valves, which in turn allow for pathogenesis to initiate. The most common conditions associated with impairment of valvular integrity include mitral valve prolapse (MVP), rheumatic heart disease (RHD), congenital cardiac malformations, and the presence of prosthetic heart valves.
Infective endocarditis in the setting of mitral valve prolapse
MVP affects 2%–3% of the US population. A descriptive analysis from the 1980s initially revealed an association between MVP and viridans group streptococci endocarditis [ ]. Prior to 2007, guidelines from the American Heart Association considered the presence of MVP to be an indication for preprocedural antibiotic prophylaxis. However, MVP has been removed as a risk factor for infective endocarditis due to the lack of evidence to suggest that the risk of postprocedural bacteremia in the setting of preexisting MVP is high enough to outweigh the risk of adverse events associated with antibiotic therapy [ ]. To further quantify the risk of infection, one study compared the risk of endocarditis in patients with MVP based on the presence or absence of a heart murmur. Patients with a murmur were found to have a probability of 1 in 1400 per year risk. While this risk seems low, it was 35 times higher than MVP patients without an audible murmur [ ].
Rheumatic heart disease
RHD is a sequela of acute rheumatic fever (ARF) which results from an autoimmune response to group A streptococcal ( Streptococcus pyogenes ) pharyngitis. The disease occurs due to the fact that these streptococci contain cardiac myosin-like sequences in their M protein, which result in immunogenic cross-reactivity and initiate valvular damage in susceptible individuals. The mitral and aortic valves are the most likely valves to be affected. Moreover, the associated chronic inflammation from this immune response can result in narrowing of the valves, resulting in subsequent reduced forward flow or leaking valves with increased regurgitation [ ]. RHD can be the result of a severe case of ARF or several recurrent episodes [ ]. In addition to damaging cardiac valves, the widespread inflammatory response can also damage a variety of other tissues, including the skin, joints, and nervous system. Prior to 1940, RHD was present in 80%–90% of patients diagnosed with endocarditis [ ].
The incidence of RHD has significantly decreased in the latter half of the 20th century, particularly in the wealthiest countries. By the 1980s, this percentage had fallen to 23%–35% in two sample populations from the United Kingdom [ ]. Improved hygiene, decreased population density, and access to medical care have all been proposed as contributing factors. However, the wide availability of penicillin for the treatment of acute streptococcal pharyngitis has been the only intervention proven to have reduced the incidence of ARF [ ].
Congenital heart disease and infective endocarditis
Congenital heart disease (CHD) is characterized by a diverse group of inborn anatomic variations including structural and functional abnormalities of the valves or great vessels as well as septal wall defects [ ]. CHD serves as an important risk factor for the development of endocarditis, particularly in the pediatric population with an incidence of two to seven times that of the general population [ , ].
Cyanotic CHD lesions, left-sided lesions, and endocardial cushion defects have been associated with an increased risk of endocarditis during childhood. Owing to medical advances, many children with CHD undergo corrective surgery or valvular replacement. However, patients are at a further elevated risk of developing infectious endocarditis during the first 6 months postoperatively [ ]. In patients who have undergone valve replacement for a congenital heart defect, they are significantly more likely to develop nosocomial endocarditis (61% vs. 14%) compared to those with native valve CHD [ ].
Prosthetic valve endocarditis
Prosthetic material, including prosthetic heart valves, serves as a nidus for the development of infections. PVE accounts for approximately 20% of all endocarditis cases [ ] with an incidence of 0.3%–1.2% per patient year [ , , ]. All prosthetic valves are at risk, but that risk varies based on the time since valve surgery and type of valve. PVE is traditionally classified as early (≤12 months postoperatively), or late (>12 months postoperatively) [ ]. It is important to recognize this differentiation because the epidemiology and microbiology of early and late had several differences.
Early
The early time frame following prosthetic valve placement is associated with a higher risk of infection. In early PVE, pathogens either gain access directly during the operative procedure or via hematogenous spread during the postoperative period. During this time, endothelialization is ongoing and pathogens have direct access to the prosthesis-annulus boundary and to the valvular/paravalvular tissue along suture tracts. Sutures can serve as an additional nidus for pathogen adherence. Direct access during this crucial time of healing to these exposed tissues can increase the risk for paravalvular abscess development [ ].
The early postoperative period is further subdivided into <2 months and 2–12 months postoperatively with regard to likely microbiologic etiologies. In the immediate (<2 month) period, the most likely pathogens are S. aureus and coagulase negative staphylococcus (CoNS) followed by GNRs and Candida species. This pathogen profile is not unexpected, given the high rate of nosocomial acquisition. Antimicrobial resistance would also be expected to be more common in this population as well. During the 2–12 months postoperative period, CoNS is the most common pathogen recovered, followed by S. aureus , streptococci, and enterococci [ , , ]. PVE during this time frame is a mix of nosocomial and community acquired pathogens, and thus variable amounts of antimicrobial resistance are usually encountered.
Late
PVE occurring >12 months postoperatively behaves similarly to native valve endocarditis, with underlying loss of valvular integrity seen similar to rheumatic and congenital heart diseases. By this point, paravalvular tissues have become endothelialized; however, the native heart structure has been altered, which changes the surface and flow characteristics of the valves. The altered valvular tissue, whether bioprosthetic or mechanical in nature, is more susceptible to the deposition of platelet/fibrin microthrombi. However, the endothelialization ultimately functions as a protective mechanism which reduces the ability of less virulent organisms, such as CoNS, from gaining a foothold and developing into vegetation. Endothelialization also functions to limit the development of late-onset paravalvular abscesses [ ].
The microbiology of late PVE also mirrors traditional NVE. Streptococci, S. aureus , followed by CoNS and enterococci are the most common pathogens in patients who do not inject drugs. Gram-negative rods and Candida spp. are also encountered in patients with a history of injection drug use or with indwelling central venous catheters [ , ].
Type of prosthetic valve
Historically, the risk of PVE is considered to be higher in patients who receive a bioprosthetic valve as compared to a mechanical valve. However, based on current literature, the difference in risk between these valve types is small. Data from the Swedish National Patient Registry (patients 50–69 years old) who underwent aortic valve replacement between 1997 and 2012 demonstrated that patients who received bioprosthetic valves had an 8.6% incidence of PVE versus 7.3% for patients with mechanical valves with a mean follow-up of 5.0 and 8.8 years, respectively [ ]. Three additional randomized trials included over 1400 patients with 8–20 years of follow-up also demonstrated trends, although not statistically significant, toward increased incidence in bioprosthetic PVE compared to mechanical PVE [ ]. A fourth study including >38,000 patients ≥ 65 years old found a 2.2% incidence of bioprosthetic PVE versus 1.4% mechanical valve PVE over a 12-year follow-up period with an adjusted hazard ratio of 1.65 (CI 1.31–1.95) [ ].
Also of interest is the risk of PVE following TAVR. TAVR was first performed in the United States in 2002 [ ] and similar to surgical aortic valve replacement (SAVR) the foreign material placed during a TAVR procedure provides a nidus for pathogen growth. Data from national Scandinavian registries and clinical trials have demonstrated a similar incidence of SAVR-associated PVE and TAVR-associated PVE as well as a higher risk during the first postoperative year [ , ]. The microbiologic causes of TAVR-associated PVE are similar to SAVR with the exception of a higher rate of enterococcal infections. Some have postulated the closer proximity of femoral access to the GU and GI tracts; however, the exact mechanism is unclear.
Clinical manifestations of infective endocarditis
Introduction
The consequences of endocarditis are seen locally within the heart as well as throughout nearly every organ system in the body. Local destruction of the heart valve and invasion of infection into the cardiac tissue can result in valvular incompetence and dehiscence, perforation of the papillary muscles, ventricular septum and wall, pericarditis, myocardial abscess, and complete heart block. Both sterile and microbe-containing emboli can travel to the lungs, spleen, blood vessels, skin, kidneys, eyes, and brain, resulting in catastrophic disease. Immunologic phenomena are not uncommon and can affect the eyes, kidneys, and the brain. The potential for endocarditis to cause such widespread damage to the body is a significant challenge to management as well as portends significant morbidity and mortality ( Figs. 1.2–1.7 ) .

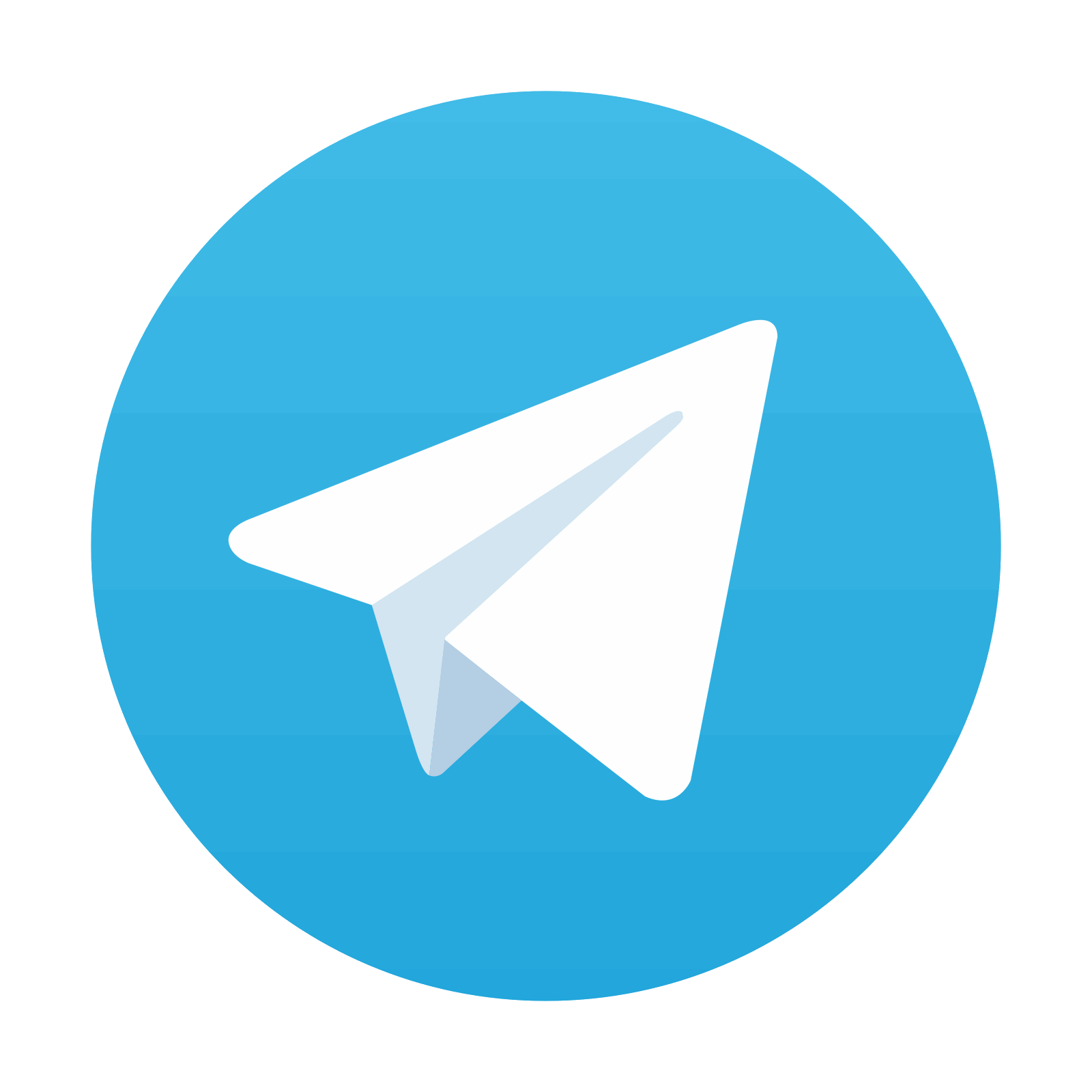
Stay updated, free articles. Join our Telegram channel

Full access? Get Clinical Tree
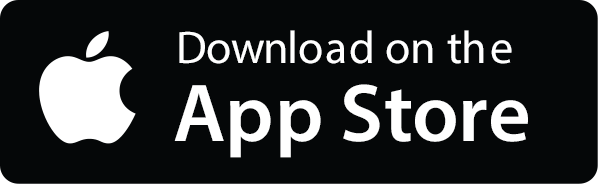
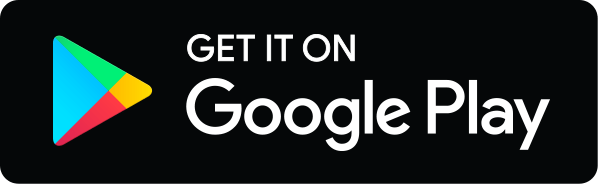
