Fig. 6.1
Graphic summary of coronary blood vessel development in relation to epicardial-derived cell lineages. The upper left cartoon reproduces the characteristic concentric arrangement of coronary blood vessel cell types (lower left pannel; red, CoE; green, CoSMC)
6.3.2 Hierarchical Differentiation of Coronary Blood Vessel Cell Progenitors
An intense debate about the arterio-venous patterning of the coronary vascular system remains open. Coronary arteries and coronary veins are morphologically different and join the systemic circulation at opposed anatomical positions, since coronary veins drain to the coronary sinus, derived from the posterior (venous) pole of the embryonic heart, and coronary arteries are connected to the aortic root, derived from the anterior (arterial) pole of the developing heart. Not only are coronary arteries and veins anatomically distinct entities, but anomalies in these two vessel types are linked to different cardiac conditions, arteries to sudden death [27] and veins to arrhythmias [28].
A possible strategy to understand the mechanics of coronary artery versus vein development is to dissect the diversity of cell lineages contributing to coronary blood vessel morphogenesis. Recent data suggests that the coronary endothelium (CoE) develops from multiple embryonic cell sources, including different endocardial subpopulations (sinus venosus and ventricular endocardium), (pro)epicardial cells, and blood-borne cells. Indeed, it is difficult to exclude contributions from other tissues to CoE since vascular progenitors are known to be extremely plastic in their phenotype. In this context, it is logical to think that building the complex arteriovenous coronary vascular tree, which develops independently from the aortic root (the point of eventual connection of the coronary circulation to the systemic one), may require a variety of cell progenitors. This is in accordance with the differentiation mechanics of the primitive coronary arterial vascular plexus, which forms isolated from the coronary venosus component, with a large contribution of ventricular endocardial cells as well as endothelial cells of different origins as mentioned above. However, some authors argue against a multilineage origin of CoE, favoring the reprogramming of arterial CoE cells from a single endocardial source, namely, the sinus venosus endocardium [29]. These authors used a clonal analysis approach to report that 30 % of CoE clones were shared with endocardial endothelium. Unfortunately, the lack of specific Cre lines for genetic tracing of defined cardiac chamber-specific endocardial populations prevents us from making definitive conclusions on this point.
Definitive patterning of coronary blood vessels requires the stable formation of the blood vessel medial wall (Fig. 6.1). Coronary smooth muscle cells (CoSMCs) are, for the major part, epicardial derivatives, although other alternative sources (e.g., neural crest) have been suggested [30]. CoSMC follow CoE differentiation being the maturation of the vessel smooth muscle wall, a late event that is triggered by the initiation of effective coronary circulation. This physiological delay in the differentiation of CoSMC is regulated by epicardial retinoids, working synergistically with VEGF to promote early vascular morphogenesis at the expenses of blood vessel muscularization [31]. It has been interpreted that late formation of the vascular wall permits CoE remodeling and proper patterning from an intricate, immature capillary network, to give rise to the characteristic “inverted crown”-shaped adult coronary vascular system. In this regard, anomalous muscularization of CoE during embryonic development could explain part of the known coronary congenital malformation phenotypes, especially those involving anomalous coronary artery course and termination [1].
A much neglected coronary cell type is that of coronary fibroblasts (Fig. 6.1). Differentiation of coronary adventitial fibroblasts (CoF) takes place once CoSMC have initiated their maturation to form the fibrous adventitia layer that appears in coronary arteries of large caliber. This adventitial layer includes a high number of epicardial-derived fibroblasts, a cell type characterized by the early expression of the transcription factor TCF21 [32], although it cannot be ruled out that heart fibroblasts of a non-epicardial origin, such as the endocardium, neural crest, or bone marrow (see [33]), can also locally contribute to the formation of the coronary adventitia.
Epicardial-derived CoF are ontogenetically related to ventricular interstitial fibroblasts [34], but their distinct location and different response to cardiovascular disease (e.g. perivascular fibrosis followed by diffuse interstitial fibrosis in pressure-overload models versus the massive, acute inflammation-induced fibrosis of the ischemic heart [35, 36]), suggests these two cardiac fibroblasts populations have diverged during their differentiation and maturation from common progenitors. Deciphering the signals that elicit specific fibrotic responses from different cardiac fibroblast populations is an important future challenge in the cardiovascular field, and some recent reports have undertaken the important task of identifying the origin of distinct populations of CFs as related to specific cardiac disease [37]. An important step will be overcoming the limitations raised by the poor specificity of current so-called fibroblast markers such as vimentin, fibroblast-specific protein 1 (Fsp1), discoidin domain receptor 2 (Ddr-2), or cluster of differentiation 90(CD90/Thy1) [33].
Conclusion
We have shown that multilineage contribution to coronary blood vessel development during the normal patterning of the embryonic and prenatal coronary blood vessel system may be a substrate for the initiation of adverse responses to pathologic stimuli. Furthermore, we have highlighted the importance of cell and tissue interactions during coronary blood vessel formation and identified the characteristic transcriptional and signaling networks whose disruption could drive diverse forms of coronary disease, to finally conclude that extensive research in the field is required to increase our knowledge on coronary blood vessel disease.
References
1.
Angelini P (2007) Coronary artery anomalies: an entity in search of an identity. Circulation 115:1296–1305PubMed
2.
3.
4.
Rentschler S, Yen AH, Lu J et al (2012) Myocardial Notch signaling reprograms cardiomyocytes to a conduction-like phenotype. Circulation 126:1058–1066PubMedCentralPubMedCrossRef
5.
Pérez-Pomares JM, de la Pompa JL (2011) Signaling during epicardium and coronary vessel development. Circ Res 109:1429–1442PubMedCrossRef
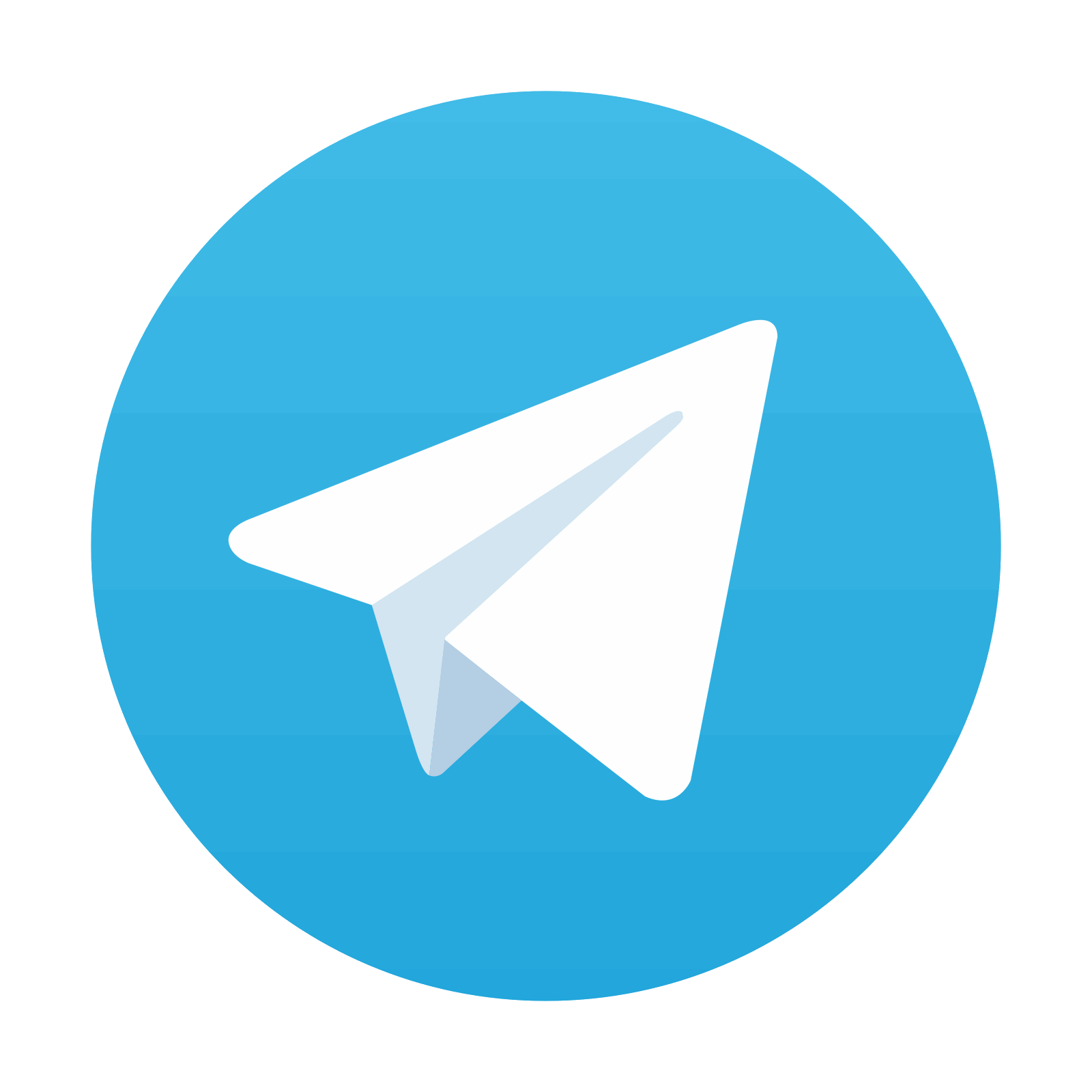
Stay updated, free articles. Join our Telegram channel

Full access? Get Clinical Tree
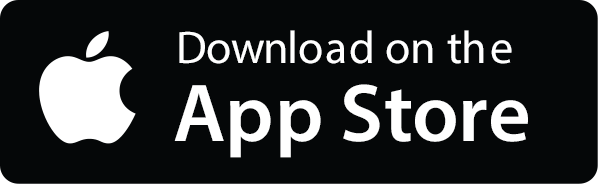
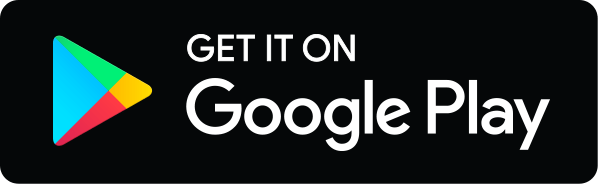