Background
Epicardial adipose tissue (EAT) measured by echocardiography has been proposed to be associated with metabolic syndrome and increased cardiovascular risks. However, its independent association with fatty liver disease and systemic inflammation beyond clinical variables and body fat remains less well known.
Methods
The relationships between EAT and various factors of metabolic derangement were retrospectively examined in consecutive 359 asymptomatic subjects (mean age, 51.6 years; 31% women) who participated in a cardiovascular health survey. Echocardiography-derived regional EAT thickness from parasternal long-axis and short-axis views was quantified. A subset of data from 178 randomly chosen participants were validated using 16-slice multidetector computed tomography. Body fat composition was evaluated using bioelectrical impedance from foot-to-foot measurements.
Results
Increased EAT was associated with increased waist circumference, body weight, and body mass index (all P values for trend = .005). Graded increases in serum fasting glucose, insulin resistance, and alanine transaminase levels were observed across higher EAT tertiles as well as a graded decrease of high-density lipoprotein (all P values for trend <.05). The areas under the receiver operating characteristic curves for identifying metabolic syndrome and fatty liver disease were 0.8 and 0.77, with odds ratio estimated at 3.65 and 2.63, respectively. In a multivariate model, EAT remained independently associated with higher high-sensitivity C-reactive protein and fatty liver disease.
Conclusions
These data suggested that echocardiography-based epicardial fat measurement can be clinically feasible and was related to several metabolic abnormalities and independently associated fatty liver disease. In addition, EAT amount may contribute to systemic inflammation beyond traditional cardiovascular risks and body fat composition.
Cardiovascular diseases have long been the leading cause of mortality and morbidity in developed countries. Visceral fat linked to central obesity has been shown to be strongly associated with metabolic derangements and higher cardiovascular risk. Recently, epicardial adipose tissue (EAT) has been proposed as a reliable predictor of visceral adiposity and metabolic abnormalities. By behaving as a source of several bioactive molecules affecting cardiac morphology and function, EAT may also exert its toxic effect on the myocardium and coronary arteries through the local secretion of proatherogenic adipokines.
Besides traditional metabolic parameters, the relationship between EAT, nonalcoholic fatty liver disease, and systemic inflammation in terms of high-sensitivity C-reactive protein (hsCRP) is less thoroughly explored. In recent years, nonalcoholic fatty liver disease linked to several metabolic disturbances and unfavorable lipid profiles has been recognized as the hepatic manifestation of metabolic syndrome (MS). This is also reflected by increases in hepatic enzymes in high-risk groups. Furthermore, the issue of using hsCRP for cardiovascular risk identification in patients with normal lipid profile has also surfaced.
The goal of this study was to examine the correlation between EAT and associated metabolic derangements, insulin resistance, fatty liver disease, systemic inflammation, and coronary atherosclerosis.
Methods
Subjects
In this retrospective analysis, echocardiography, liver ultrasound, and coronary artery calcium scoring with computed tomographic (CT) imaging were performed in 361 consecutive subjects who participated in a primary cardiovascular health survey at a tertiary medical center in Taipei, Taiwan, from 2006 to 2008. Patients with previous myocardial infarction, stroke, coronary arterial disease, peripheral arterial disease or prior hospitalization for congestive heart failure were excluded. The presence of a history of hypertension was defined as systolic blood pressure >140 mm Hg, diastolic blood pressure >90 mm Hg, or previously diagnosed hypertension under pharmaceutical control. Diabetes mellitus was defined as fasting glucose level >126 mg/dL or previously diagnosed diabetes mellitus under pharmaceutical control. Hyperlipidemia was defined a known history and/or the use of lipid-lowering drugs, such as statins or fibrates, on a daily basis. MS was defined as fulfilling at least three of five criteria from the National Cholesterol Education Program Adult Treatment Panel III. Nonalcoholic fatty liver disease was defined on abdominal echocardiography after the exclusion of excessive alcohol use, hepatitis B or C confirmed by serologic tests, and other known etiologies of liver disease.
Anthropometric Measurements
All baseline characteristics and information regarding anthropometric measures were collected. Standardized cuff sphygmomanometer–defined resting blood pressures were measured by medical staff members blinded to the other test results. Body fat analysis was assessed using bioelectrical impedance from foot-to-foot measurements with a Tanita-305 body fat analyzer (Tanita Corporation, Tokyo, Japan), which provides an estimate of impedance and calculated body fat composition. Data output included body weight, impedance, fat mass, fat-free mass, and fat percentage as calculated by the manufacturer’s software.
Lab Data Acquisition and Analysis
A Hitachi 7170 Automatic Analyzer (Hitachi Corporation, Hitachinaka Ibaraki, Japan) was used to measure fasting and postprandial glucose levels (hexokinase method), glycosylated hemoglobin, creatinine (kinetic colorimetric assay), total cholesterol and triglyceride, and alanine aminotransferase (enzymatic method). Lipid profiles including low-density lipoprotein and high-density lipoprotein cholesterol were obtained using homogenous enzymatic colorimetric assay. HsCRP levels were determined using a highly sensitive, latex particle–enhanced immunoassay (Elecsys 2010; Roche Diagnostics GmbH, Mannheim, Germany).
Echocardiographic Measurements
Each subject underwent two-dimensional and M-mode transthoracic echocardiography using a Hewlett-Packard Sonos 5500 (Philips Medical Systems, Andover, MA) equipped with a 2.5-MHz to 4.5-MHz transducer, with images stored on videotapes. Standard parasternal and apical views were obtained in the left lateral decubitus position. Epicardial fat is generally identified as the echo-free space between the myocardium and pericardium. For parasternal long-axis assessment, EAT thickness was measured at the right ventricular free wall on M-mode cuts generated by placing the ultrasound beam perpendicular to the aortic annulus as proposed by Iacobellis et al. For parasternal short-axis assessment, the view at the aortic valve level was chosen to validate with CT measurements at the same level ( Figures 1 A and 1 B). Previous studies suggested that the papillary muscle level is best visualized on echocardiography, but because we were comparing with CT images with or without contrast enhancement, the aortic root was thought to serve as a better anatomic landmark because the interventricular septum and papillary muscles could not be differentiated or well visualized on nonenhanced CT imaging. Three repeated measures of maximum depth at end-systole from both views were performed, with the average data to be representative in our study. For reproducibility assessment, intraobserver and interobserver coefficients of variation were determined by repeated measurement on 35 randomized cases by two experienced readers blinded to the subjects’ clinical data.

Liver Ultrasound
We used ultrasound, a widely used surrogate for liver biopsy, for the assessment of fatty liver. Abdominal sonography was performed using a Toshiba Nemio SSA-550A instrument (Toshiba, Tochigi-ken, Japan) by hepatology specialists completely blinded to other laboratory results. In brief, liver pathology was further graded semiquantitatively as absent, mild, or moderate to severe according to the level of echoes arising from the hepatic parenchyma, as suggested in previous studies. On the basis of the acknowledgment that the interpretation of fatty liver by abdominal ultrasound may tend to be subjective and for statistical ease, we further defined subjects with at least moderate fatty liver disease as having significant fatty liver disease in this study.
Multidetector CT Measurements of Epicardial Fat
One hundred seventy-eight randomly chosen participants were selected for comparison and validation with CT imaging (58 subjects compared with contrast CT imaging and 120 subjects compared with noncontrast CT imaging). Scanning was performed using a 16-slice multidetector CT scanner (Sensation 16; Siemens Medical Solutions, Forchheim, Germany) with 16 × 0.75 mm collimation, rotation time of 420 msec, and tube voltage of 120 kV. In one breath hold, images were acquired from above the level of tracheal bifurcation to below the base of heart using prospective electrocardiographic triggering, with the center of the acquisition at 70% of the R-R interval. Multiplanar reconstructions of the multidetector CT data were obtained in the short-axis planes at the aortic valve level, as well as the standardized three-chamber view. To ensure that all measurements across the patients were consistent, the plane included three landmarks on CT imaging: the center of aortic valve for short-axis, the center of mitral annulus, and the apex of the left ventricle for long-axis orientation corresponding to echocardiography-derived images. EAT was measured at the aortic root level along the retrosternal area on the right ventricular free wall in both views. ( Figures 1 C– 1 F).
Coronary Artery Calcium Measurement
We measured the calcification of the coronary arteries by using a dedicated offline workstation (Aquarius 3D Workstation; TeraRecon, San Mateo, CA). A coronary calcified lesion was defined as an area with a density >130 Hounsfield units and covering ≥6 pixels. The Agatston scoring method was applied by multiplying each lesion (area) by a weighted CT attenuation score in the lesion.
Statistical Analysis
Continuous data are expressed as mean ± SD and categorical data as frequencies and proportions of occurrence in all subjects. Differences of baseline demographics and anthropometric and metabolic parameters between groups were tested using analysis of variance, with categorical data analyzed using χ 2 or Fisher’s exact tests as appropriate. Post hoc comparisons between each group were further performed using Bonferroni’s correction. Wilcoxon’s rank-sum tests were used to estimate the trend of all continuous data and ordinal variables across all ordered groups. Receiver operating characteristic curves was used to calculate the areas under the curves in estimating MS and significant fatty liver disease from both echocardiography-derived EAT measures. A multivariate logistic regression model was used to determine the significance of covariate-adjusted relations between epicardial fat, hsCRP, and fatty liver disease with individual odds ratios, P values, and 95% confidence intervals. The comparison and validation of epicardial fat depth with CT imaging was performed by linear regression analysis. All data were analyzed using Stata version 8.0 (StataCorp LP, College Station, TX). The significance of P level (α value) for all analysis was two-sided, with values <.05 considered statistically significant.
Results
Baseline Demographic Data and Anthropometric and Metabolic Correlates
A total of 359 subjects (mean age, 51.6 years; 31% women) had sufficient image quality allowing both long-axis and short-axis measurements. The remaining two patients were excluded because the epicardial fat layer could not be identified very well on their short-axis views. Mean epicardial fat thickness was 7.6 ± 1.4 mm. The intraobserver and interobserver coefficients of variation for long-axis and short-axis EAT were 5.4% and 6.2% and 5.8% and 6.9%, respectively.
All subjects were divided into tertiles on the basis of average fat thickness. In our cohort, 81 subjects (22.4%) were diagnosed with MS according to our defined criteria. Other baseline characteristics, including blood pressure, anthropometrics, biochemical markers, and medical histories, are listed in Tables 1 and 2 . We observed that both systolic and diastolic blood pressures were significantly related to increasing EAT thickness ( P values for trend = .01 and .02, respectively). For anthropometric parameters, increasing EAT tertile groups were significantly associated with graded increase in body mass index, body weight, and waist circumference (all P values for trend = .005; P < .01 for highest vs lowest tertile by analysis of variance).
Variable | EAT tertile | P for trend | ||
---|---|---|---|---|
First (≥4.2 and <7.0 mm) ( n = 121) | Second (≥7.0 and <8.2 mm) ( n = 119) | Third (≥8.2 and <11.5 mm) ( n = 119) | ||
Age (y) | 50.5 ± 7.5 | 52.4 ± 9.5 | 52.3 ± 9.3 | .24 |
Women | 42 (34.7%) | 38 (30.9%) | 32 (27.1%) | .45 |
SBP (mm Hg) | 117.9 ± 17.6 | 124.2 ± 16.2 ∗ | 123.2 ± 15.8 ∗ | .01 |
DBP (mm Hg) | 74.2 ± 10.6 | 77.1 ± 10.4 | 77.2 ± 10.6 | .02 |
Weight (kg) | 64.4 ± 10.9 | 67.2 ± 10.8 | 70.2 ± 10.7 ∗ | .005 |
BMI (kg/m 2 ) | 23.8 ± 2.8 | 24.6 ± 2.9 | 25.3 ± 3.0 ∗ | .005 |
Waist (cm) | 81.1 ± 9.5 | 85.3 ± 8.1 ∗ | 86.4 ± 8.6 ∗ | .005 |
Body fat composition (%) | 25.3 ± 6.5 | 26.8 ± 5.6 | 26.5 ± 7.9 | .27 |
∗ P < .01 versus first tertile by analysis of variance with Bonferroni’s correction.
Variable | EAT tertile | P for trend | ||
---|---|---|---|---|
First (≥4.2 and <7.0 mm) ( n = 121) | Second (≥7.0 and <8.2 mm) ( n = 119) | Third (≥8.2 and <11.5 mm) ( n = 119) | ||
Fasting glucose (mg/dL) | 98.2 ± 24.1 | 99.8 ± 15.6 | 101.7 ± 18.1 | .01 |
Postprandial glucose (mg/dL) | 112.8 ± 44.7 | 118.3 ± 42.5 | 117.8 ± 35.7 | .02 |
HbA 1c (%) | 5.93 ± 0.82 | 5.97 ± 0.72 | 5.99 ± 0.7 | .15 |
Insulin (μIU/mL) | 5.41 ± 2.55 | 6.16 ± 3.31 | 6.72 ± 3.85 | .04 |
HOMA-IR (IU/mL) | 1.37 ± 1.27 | 1.46 ± 0.91 | 1.7 ± 1.06 ∗ | .01 |
ALT (IU/L) | 28.2 ± 15.8 | 30.8 ± 17.5 | 32.6 ± 15.9 | .005 |
Cholesterol (mg/dL) | 201.3 ± 37.3 | 201.2 ± 36.9 | 194.3 ± 33.6 | .27 |
TG (mg/dL) | 127.7 ± 74.2 | 148.3 ± 82.8 | 144.2 ± 87 | .1 |
LDL (mg/dL) | 131.5 ± 32.1 | 132.6 ± 32.9 | 127.8 ± 29.7 | .66 |
HDL (mg/dL) | 53.5 ± 13.3 | 48.4 ± 13 ∗ | 47.7 ± 12.2 ∗ | .005 |
eGFR (mL/min) | 86.7 ± 14.1 | 85.4 ± 16 | 84.1 ± 19.9 | .25 |
CCS | 37 ± 126.9 | 32.3 ± 141.1 | 50.9 ± 206.5 | .88 |
Hypertension | 24 (19.8%) | 34 (27.6%) | 39 (33.1%) | .068 |
Diabetes | 10 (8.3%) | 20 (16.3%) | 22 (18.6%) | .056 |
Hyperlipidemia | 1 (1%) | 17 (13.8%) | 27 (22.9%) | <.001 |
MS | 4 (3.31%) | 21 (17.1%) | 56 (47.5%) | <.001 |
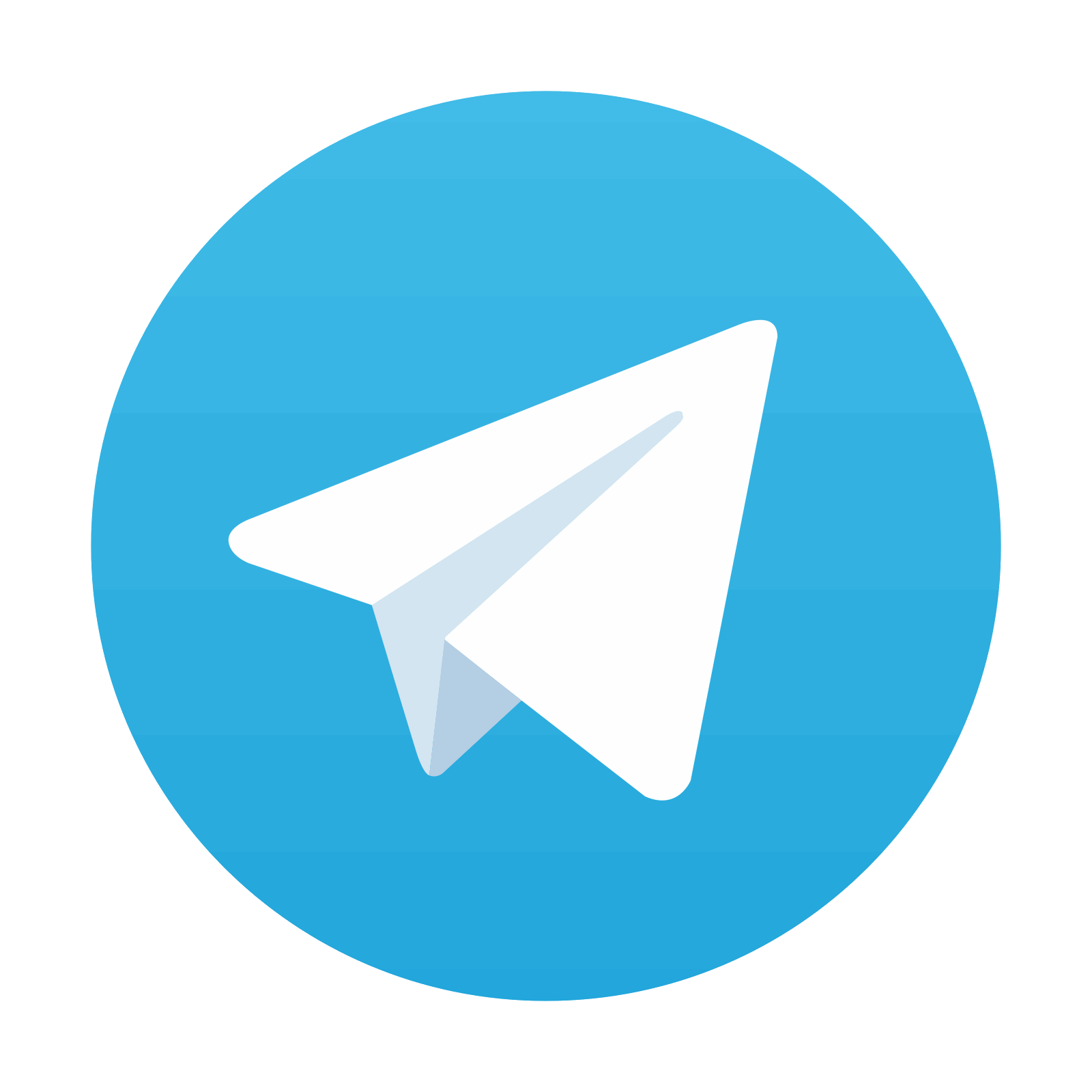
Stay updated, free articles. Join our Telegram channel

Full access? Get Clinical Tree
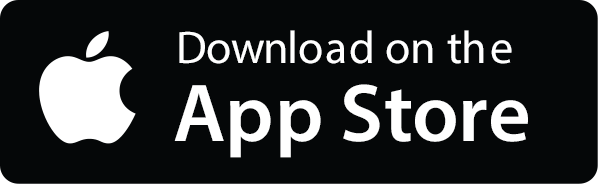
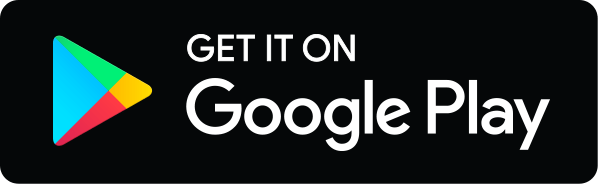
