(1)
Cardiology, University of Wisconsin, Madison, WI, USA
(2)
Loyola University Chicago, Stritch School of Medicine, Loyola University, Maywood, IL, USA
(3)
Medicine, Cardiology, Loyola University Medical Center, Maywood, IL, USA
(4)
Internal Medicine, Cardiovascular Medicine UC Davis Vascular Center, Sacramento, CA, USA
(5)
Medicine, Cardiology, Vascular and Endovascular Medicine, Loyola University Medical Center, Maywood, IL, USA
Keywords
Percutaneous transluminal angioplastyBare-metal stentBare-nitinol stentDrug-eluting stentCryoplastyCutting balloonsScoring balloonsDrug-eluting balloonStent graftAtherectomyExcimer laserExcisional atherectomyOrbital atherectomyVibrational angioplastyFemoropoplitealInfrapoplitealAbove kneeBelow kneeChronic total occlusionCrossing deviceReentry deviceEmbolic protection devicePaclitaxelSirolimusEverolimusRapamycinPeripheral artery disease (PAD) rates have risen globally over the past decade. PAD currently affects one in ten people over 70 years of age [1]. The prevalence of PAD in patients with risk factors such as diabetes, hypertension, and obesity may be as high as 26 % [2]. With the increasing rate of associated risk factors and the overall aging population, the incidence of PAD will likely continue to climb. When suitable, percutaneous intervention is frequently used as a treatment of lower extremity occlusive artery disease and, in many cases, has shown more satisfactory results than more invasive techniques [3–5]. One of the main challenges of using endovascular interventions continues to be preventing restenosis of the altered segment of vessel.
Mechanism of Balloon Angioplasty and Stenting
Use of percutaneous transluminal angioplasty (PTA) also referred to as balloon angioplasty in the treatment of occlusive arterial disease relies on several mechanisms of increasing the arterial lumen diameter. First, pressure from the balloon causes a controlled dissection of the intima and media, while simultaneously compressing the atherosclerotic plaque. Contents of the plaque are pushed longitudinally and axially in the vessel wall as the balloon expands [6]. The acute benefit is limited by arterial response to this insult resulting in elastic recoil causing up to a 40 % reduction in the lumen diameter [6, 7]. Deploying a stent during this process assists in increasing lumen area by providing scaffolding for the artery wall that almost completely prevents the elastic recoil [6]. Additionally, dissection creates intimal flaps that increase the risk of acute or subacute vessel closure. Stenting helps reduce the risk of immediate closure by keeping the flaps pressed between the scaffolding and arterial wall [8].
Pathophysiology of Restenosis
Studies have shown that restenosis is dictated by elastic recoil, negative/constrictive remodeling, and neointimal hyperplasia [6, 9]. In stented segments, the structural support of struts effectively eliminates the effects of recoil and negative remodeling, making neointimal hyperplasia the limiting factor of long-term maintenance of arterial patency [10–12]. This process is incited by the increased inflammation from the chronic presence of the stent. Smooth muscle cells from the media are stimulated to migrate and proliferate, causing luminal narrowing.
Several mechanisms behind restenosis have been studied in animal models. There are three major aspects of the process: denudation of the endothelium, disruption of the intima, and penetration of the smooth muscles in the vascular media [7, 9, 12]. Each injury to the vessel contributes to late restenosis through the attempted repair mechanism. Denudation of the epithelial surface results in the release of pro-inflammatory and procoagulant factors, adhesion molecules, and growth stimulators. Rupturing the plaque and disruption of the endothelium expose subepithelial collagen to the lumen contents. Circulating and locally released vonWillebrand factor binds the collagen surface, allowing platelet adherence and activation. Injury results in the activation of platelets, plaque rupture, release of growth factors, inflammatory cytokines, reactive oxygen species combined, and macrophage chemotactic factors [7, 9, 13]. This environment along with fibrin deposition and thrombosis aids in cellular migration by providing a matrix [7]. Platelet degranulation releases mitogens that promote further cell activation and proliferation [12, 14, 15]. Smooth muscle cells and fibroblasts then migrate to the site of injury. Eventually, this process of repair results in smooth muscle migration from the media to the intima above the stent, followed by cellular proliferation. The end result is a neointimal scar consisting mainly of collagen, proteoglycans, and vascular smooth muscle cells [7, 9, 12, 13].
Animal models with verification through human studies have experimentally determined the time and order of the vascular proliferative response after stent implantation. Deployment of the stent initiates the process as described above. Acutely, there is an invasion of inflammatory cells around the struts with associated platelet adherence and deposition of fibrin. In the following few weeks, the struts are covered in immature neointima, consisting of macrophages and alpha-actin-negative spindle cells. Smooth muscle cells then differentiate and begin proliferating, while additional extracellular matrix is deposited by fibroblasts. Several weeks to months later, there is a re-endothelialization process that concludes the proliferative response .
Endovascular Interventions for Critical Limb Ischemia in the Lower Extremity
The management of lower extremity PAD can be quite complex and presents a number of very unique clinical and therapeutic challenges especially in the setting of critical limb ischemia (CLI) . The underlying complex nature of PAD has limited the success of a number of endovascular therapeutic modalities in the treatment of CLI; these clinical challenges have been the driving force behind the development and advancement in endovascular therapies. This review will aim to highlight the historical challenges of endovascular intervention in the treatment of CLI as well as focus on the advancements which have been developed. While the primary focus of this chapter is treatment/management of CLI, included are several studies which evaluated CLI, symptomatic ischemia, and intermittent claudication as inclusion criteria. While some of the presented trials included both above- and below-the-knee lesions in the reported data (and are therefore presented as such), every effort was made to separate and present the available data by anatomic location, as the treatment and results for each of the reviewed therapeutic modalities may differ between above- and below-the-knee lesions. A summary of the important individual studies appears in Table 35.1.
Table 35.1
Summary of important studies evaluating endovascular technologies in lower extremity peripheral artery disease (including critical limb ischemia)
Trial name | Study population | Outcomes measured (major) | Results |
---|---|---|---|
Percutaneous transluminal angioplasty | |||
Dorros et al. [NR] [18] | 235 patients (284 ischemic limbs) | Technical success [E] | 95 % |
5-year limb salvage [E] | 91 % | ||
Söder et al. [NR] [19] | 121 de novo lesions and 67 chronic total occlusions | Primary angiographic success [E] | 84 % vs 61 %* |
Trocciola et al. [NR] [21] | 58 patients with claudication and 63 patients with CLI | Primary patency at 3, 6, and 9 months [E] | 100, 98, and 85 % vs 89, 80, and 72 % |
Limb salvage at 12 months (CLI only) [E] | 91 % | ||
PTA vs bypass | |||
BASIL [R] [5] | 224 in PTA arm and 228 in bypass arm | 6-month amputation-free survival [E] | 48 vs 60 (HR 1.07, CI 0.72; 1.6) |
Bare-metal stents | |||
Vogel et al. [NR] [24] | 41 patients (68 % for limb salvage) | Primary patency at 6, 12, and 24 months [E] | 95, 84, and 84 % |
Limb salvage at 6, 12, and 24 months [E] | 92, 89, and 89 % | ||
RESILIENT [R] [25] | 134 patients in the BMS arm and 72 patients in PTA arm | Primary patency at 12 months [E] | 81.3 % vs 36.7 %* |
Freedom from TLR at 12 months [E] | 87.3 % vs 45.1 %* | ||
STROLL [NR] [26] | 250 patients | Primary patency at 1 year [E] | 81.70 % |
Freedom from 30 days’ MAEs [S] | 100 % | ||
SUPERB [NR] [32] | 264 patients (265 lesions) | Primary patency at 1 year [E] | 78.90 % |
Stent grafts | |||
Saxon et al. [R] [41] | 97 in stent-graft arm and 100 patients in PTA arm | Technical success [E] | 95 % vs 66 %* |
Primary patency at 1 year [E] | 65 % vs 40 %* | ||
40 patients (50 limbs) in stent-graft arm and | Primary patency at 1 year [E] | 73.5 % vs 74.2 % | |
40 patients (50 limbs) in bypass arm | Primary patency at 4 years [E] | 59 % vs 58 % | |
VIBRANT [R] [45] | 72 patients in stent-graft arm | Primary patency at 3 years [E] | 24.2 % vs 25.9 % |
And 76 patients in BMS arm | Freedom from 30 days of MAEs [S] | 98.6 % vs 100 % | |
VIPER [NR] [46] | 113patients (119 limbs) | Primary patency at 1 year [E] | 73 % |
30 days of procedure-related MAEs [S] | 0.80 % | ||
VIASTAR [R] [47] | 72 patients in stent-graft arm | Primary patency at 1 year [E] | 70.9 % vs 55.1 % |
And 69 patients in BMS arm | 30 days of procedure-related MAEs [S] | 15 % vs 13 % | |
Drug-eluting stents | |||
Femoropopliteal disease | |||
SIROCCO I [R] [55] | 18 patients in DES arm and 18 patients in BMS arm | 6-month in-stent mean percent diameter stenosis by | 22.6 % vs 30.9 % |
quantitative angiography [E] | |||
29 patients in DES arm and 28 patients in BMS arm | 6-month in-stent mean luminal diameter [E] | 4.94 mm ± 0.69 vs 4.76 mm ± 0.54 | |
STRIDES [NR] [59] | 104 patients | In-stent binary restenosis at 6 months [E] | 14 % (CI 7.8;22.2) |
236 patients in DES arm vs 238 in primary PTA arm | Primary patency at 1 year in primary randomization [E] | 83.1 % vs 32.8 %* | |
120 patients with acute PTA failure randomized to | Event-free survival at 1 year in primary randomization [S] | 90.4 % vs 82.6 %* | |
provisional DES (61) or BMS arms (59) | |||
Infrapopliteal disease | |||
Falkowski et al. [R] [69] | 25 patients in DES arm and 25 patients in BMS arm | Restenosis rate at 6 month [E] | 16 % vs 76 %* |
ACHILLES [R] [70] | 99 patients in DES arm and 101 patients in PTA arm | In-stent binary restenosis at 12 months [E] | 22.4 % vs 41.9 %* |
YUKON-BTK [R] [71] | 82 patients in DES arm and 79 patients in BMS arm | Primary patency at 1 year [E] | 80.6 % vs 55.6 %* |
DESTINY [R] [73] | 74 patients in DES arm and 66 patients in BMS arm | Primary patency at 1 year [E] | 85 % vs 54 %* |
Cryoplasty | |||
Femoropopliteal disease | |||
Cold [R] [78] | 46 patients in PTA arm and 40 patients in cryoplasty arm | Technical success [E] | 54 % vs 35 %* |
Infrapopliteal disease | |||
BTK Chill [NR] [80] | 108 patients | Technical success [E] | 97.30 % |
Absence of amputation at 6 and 12 months [E] | 93.4 % and 85.2 % | ||
Cryoplasty-related complications [S] | One dissection, two residual stenoses, three required additional stenting | ||
CLIMB [NR] [81] | 100 patients | Primary patency at 12 months [E] | 55.9 ± 7.4 % |
Cutting balloon angioplasty | |||
Canuad et al. [NR] [84] | 16 patients in the claudication arm | Primary patency at 12 and 24 months [E] | 82.1 % and 82.1 % |
116 patients in the CLI arm | Primary patency at 12 and 24 months [E] | 64.4 % and 51.9 % | |
Amighi et al. [R] [86] | 21 patients in the CBA arm and 22 patients in the PTA arm | Restenosis rates at 6 months [E] | 62 % vs 32 %* |
Drug-eluting balloons | |||
Femoropopliteal disease | |||
48 patients in DEB arm, 54 patients in PTA arm, | Late lumen loss at 6 months [E] | 0.4 mm ± 1.2*, 1.7 mm ± 1.8, 2.2 mm ± 1.6 | |
52 patients in paclitaxel in contrast medium arm | |||
FEMPAC [R] [96] | 45 patients in DEB Arm vs 42 patients in PTA arm | Late lumen loss at 6 months [E] | 0.5 mm ± 1.1 vs 1.0 mm ± 1.1* |
LEVANT I [R] [97] | 49 patients in DEB arm vs 52 patients in PTA arm | Late lumen loss at 6 months [E] | 0.46 mm ± 1.13 vs 1.09 mm ± 1.07* |
LEVANT II [R] [98] | 316 patients in DEB arm vs 160 patients in PTA arm | Primary patency at 1 year [E] | 65.2 % vs 52.6 %* |
Freedom from MAEs [S] | 83.9 % vs 79 % | ||
PACIFIER [R] [99] | 44 cases in DEB vs 47 cases in PTA arm | Late lumen loss at 6 months [E] | −0.01 mm [CI −0.29; 0.26] vs 0.65 mm [CI 0.37; 0.93] |
44 lesions in DEB vs 48 in PTA arm | Late lumen loss at 6 months [E] | ||
IN.PACT SFA [R] [102] | 220 patients in DEB vs 111 in PTA arm | Primary patency at 1 year [E] | 82.2 % vs 52.4 %* |
Infrapopliteal disease | |||
DEBATE-BTK [R] [110] | 65 patients in DEB arm vs 67 patients in PTA arm | Binary restenosis at 12 months [E] | 27 % vs 74 %* |
13 lesions in DEB vs 17 in PTA arm | Late lumen loss at 6 months [E] | ||
IN.PACT DEEP [R] [111] | 239 patients in DEB arm vs 119 patients in PTA arm | Clinically driven TLR (CD-TLR) at 12 months [E] | 9.2 % vs 13.1 % |
Late lumen loss at 12 months [E] | 0.61 mm ± 0.78 vs 0.62 mm ± 0.78 | ||
Composite of death, amputation, and CD-TLR at 6 months [S] | 17.7 % vs 15.8 % |
Percutaneous Transluminal Angioplasty
Some of the earliest angioplasty data were reported in the Trans-Atlantic Inter-Society Consensus document for the management of peripheral arterial disease (TASC I) which summarized the results of 19 different registries dating back to the early 1990s [16]. Primary technical success rates ranged between 80 and 100 % and limb salvage rates between 88 % and 54 % at 17 and 24 months, respectively, with low rates of complication between 2 and 6 %. Dormandy et al. published these results showing that the endovascular treatment of lower limb ischemia with unassisted balloon angioplasty has primary patency rates of 61 %, 51 %, and 48 % at 1, 3, and 5 years, respectively [16].
Subsequently, based on their results in a study of 53 patients with infrapopliteal disease, Bakal et al. proposed that the restoration of linear flow to the distal leg was the most important predictor of success [17]. They found the rate of limb salvage was 80 % when straight-line flow was restored and approached 0 % when distal flow remained obstructed. Dorros et al. published their results of a prospective, nonrandomized consecutive series of 235 CLI patients (284 ischemic limbs, 529 tibioperoneal lesions) treated between 1983 and 1996 [18]. Primary success was reported in 95 % (270) of ischemic limbs. At 5-year follow-up, the rate of limb salvage was 91 % (5 above-the-knee (ATK ) and 18 below-the-knee (BTK ) amputations) with a cumulative survival of 56 %. Söder and associates subsequently published results of a prospective trial of 60 patients (72 limbs) in which primary angiographic success was 84 % in treatment of stenosis and 61 % in chronic total occlusions, with 10-month restenosis rates of 32 and 52 %, respectively [19]. Limb salvage at 18 months based on Kaplan–Meier analysis was found to be 80 %. These results in conjunction with the fact that PTA carries lower morbidity and mortality, shorter hospitalization, and does not preclude surgery are a very attractive option for the management of CLI [20].
While PTA alone as an intervention has showed promising results, it is imperative to understand how PTA compares to PTA with adjunctive therapies . In a retrospective review published by Trocciola et al., the clinical success, patency, and limb salvage rates after endovascular repair were evaluated in patients with PAD who presented with either claudication (n = 58) or critical ischemia (n = 63) [21]. In all, 199 lesions were treated with PTA + subintimal angioplasty (tibial lesions) or adjunctive stenting (self-expanding nitinol stents if femoropopliteal lesions and balloon-expandable stainless steel for tibial lesions). At 3, 6, and 9 months in the claudicant group, primary patency rates recorded were 100 %, 98 %, and 85 %, respectively, while in the critical ischemia group, the 3-, 6-, and 9-month primary patency rates were reported as 89 %, 80 %, and 72 % with limb salvage rate of 91 % at 12 months. Results cemented the role of PTA ± adjunctive therapies as an acceptable mode for intervention with acceptable patency at 12 months for both claudication and CLI.
PTA vs Surgical Bypass
As CLI patients often present with multiple medical comorbidities and are often considered high risk for high-risk vascular surgery, it is imperative to understand the comparison between PTA and bypass surgery. The Bypass versus Angioplasty in Severe Ischemia of the Leg (BASIL) trial was a randomized controlled multicenter trial comparing PTA (n = 224) versus surgery (n = 228) as first-line therapy in 452 patients with severe limb ischemia secondary to infrainguinal disease [5]. Both groups were followed for a primary endpoint of amputation-free survival. At 6 months, there was no significant difference in amputation-free survival (48 vs 60, HR 1.07, 95 CI 0.720–1.6) and no difference in health-adjusted quality of life; there was however a significant increase in hospital cost (roughly one third higher for surgery arm over the first year). The authors, however, did report a significant reduction in amputation-free survival and all-cause mortality in the surgical bypass arm beyond 2 years of follow-up from randomization. Thus, surgery may be a better choice for patients expected to survive beyond 2 years of the procedure; otherwise, PTA is a viable option for intervention in these patients .
Bare-Metal Stents vs Angioplasty
There have been many trials comparing balloon angioplasty to balloon angioplasty with bare-metal stent (BMS) for superficial femoral artery (SFA) stenosis in patients with critical limb ischemia or claudication. Cumulative results published in a Cochrane review compared the two treatments head-to-head in regard to the duplex patency, angiographic patency, ankle-brachial index, treadmill walking distance, and quality of life [22]. The completed review included 11 trials with a total of 1387 patients (average age of 69). Patients were followed for up to 2 years. The results of the review found a significant improvement in duplex patency at 6 (six studies, 578 patients) and 12 months (9 studies, 858 patients) in the group who received PTA + stent versus PTA alone; this early benefit however was lost when followed out to 24 months. In regard to angiographic patency, four studies (329 patients) confirmed the short-term patency benefit in the PTA + stent group at 6 months; this too however was lost by 12 months (5 studies, 384 patients). ABI and treadmill walk test were found to have no statistically significant difference between groups. Perhaps the most interesting parameter followed in 3 of the 11 studies was assessment in quality of life (660 patients); there was unfortunately no significant difference between treatment groups. While the study showed that there was a statistically significant difference in short-term patency with PTA + stenting, there was no sustained benefit to the combination intervention over PTA alone.
A meta-analysis published by Mwipatayi et al. compared the short- and long-term results of primary PTA versus primary stenting in 1362 patients from seven RCTs (934 patients underwent angioplasty and 482 underwent stenting) [23]. Both groups were assessed in regard to primary patency at 12 months and post-intervention ABI. Primary patency at 12 months ranged from 45 to 84.2 % in the angioplasty arm and 63–90 % in the stenting arm; there was no statistically significant difference between groups (OR 0.989, 95 % CI 0.623–1.57, p = 0.962). Additionally, both groups exhibited similar occlusion rates at 12 months (19 and 22 %, respectively). Despite these results, there was however a nonstatistically significant trend toward improved ABI in the angioplasty group as compared to the primary stenting group (three studies with balloon-expandable stents, two studies with self-expandable stents) .
Nitinol Self-Expanding Stents
The practice of stenting in peripheral arterial disease (predominantly using balloon-expandable and self-expanding stainless steel stents) was primarily in the setting of suboptimal results with PTA alone [16]. The advent of self-expanding nitinol stents, which allow for constant radial force to be exerted on the arterial wall and increased flexibility, made it possible to consider deployment as an option for primary therapy in femoropopliteal lesions. Individual trials and stents will be reviewed to highlight their utility.
Vogel et al. reported their results of a retrospective analysis of 41 patients who underwent placement of a nitinol stents either in the femoral (n = 35) or above-the-knee popliteal arteries (n = 6) [24]. They reported that 68 % of patients studied underwent intervention for the indication of limb salvage. They were followed from 1999 to 2002 for both primary patency and limb salvage. The 6-month, 1-year, and 2-year primary patency rates and limb salvage rates were 95 %, 84 %, and 84 % and 92 %, 89 %, and 89 %, respectively.
Laird et al. published their results from the prospective, Randomized Study Comparing the Edwards Self-Expanding Lifestent versus Angioplasty Alone in Lesions Involving the SFA and/or Proximal Popliteal Artery (RESILIENT) trial [25]. Two Hundred and six patients were enrolled and randomized to either intervention with a self-expanding nitinol stent (n = 134) or PTA (n = 72). Immediate technical success (<30 % stenosis) was superior in the stent group compared to the angioplasty group (95.8 % vs 83.9 %, p < 0.01), with stents also having better primary patency (81.3 % vs 36.7 %, p < 0.0001) and freedom from TLR at 12 months (87.3 % vs 45.1 %, p < 0.0001), respectively. Stent fractures occurred in 3.1 % of stents at 12 months with none resulting in loss of patency or TLR.
Gray et al. published the results of the S.M.A.R.T. Self-Expanding Nitinol Stent for the Treatment of Atherosclerotic Lesions in the Superficial Femoral Artery (STROLL) trial [26]. The trial was a single-arm, prospective, open-label multicenter trial (39 US centers) comprising of 250 patients to assess safety and efficacy of the S.M.A.R.T. Vascular Stent System stent (Cordis, Johnson & Johnson, Warren, NJ) in the treatment of SFA disease in patients with symptomatic limb ischemia. Primary patency rates at 1 year were reported as 81.7 % by Kaplan–Meier estimate. There were no major adverse events reported in the first 30 days (death, index limb amputation, clinically driven TLR), and only four patients (2 %) experienced single-stent strut fracture at 1 year without associated loss of stent patency .
Supera Stent
The Supera Peripheral Stent System (Abbott Vascular, Santa Clara, CA) is an interwoven nitinol stent constructed from six pairs of nitinol wires forming closed loops at both ends. The design of this stent aims to counteract concerns for stenting in femoral and popliteal vessels relating to rate of stent fractures and consequent restenosis. The biomechanics of this helical stent impart greater radial compression resistance and flexibility without unwanted chronic outward force or barotrauma against the artery, theoretically making it ideal for SFA and popliteal interventions. Clinical experience has been very encouraging. Registry data from Leipzig, Germany, have reported accumulated experience in SFA (137 stents) and popliteal (125 stents) lesions, with 12-month patency rates of 84.7 % ± 3.6 % and 87.7 ± 3.7 %, respectively [27, 28]. In the second study with popliteal lesions, about 23 % of the patients had CLI. In another registry from the United States with 39 stent cases, primary patency rate at 1 year remained at 79.2 % [29]. Most patients (74 %) in this study had CLI. Another Chinese registry also reported a patency rate of about 79 % in 82 cases [30], with 42 % of the study sample having CLI at baseline. Similarly, George et al. reported a primary patency of about 86 % at 1 year in 98 limbs with Supera stents [31]. Thirty-one percent of the cases had CLI. None of these studies have reported any stent fractures.
In order to further evaluate this stent system, the Comparison of the SUpera PERipheral System to a Performance Goal Derived from Balloon Angioplasty Clinical Trials in the Superficial Femoral Artery (SUPERB) was designed as a single-arm study which evaluated a total of 265 lesions (264 patients) in the SFA or proximal popliteal artery with the use of the Supera stent [32]. Primary patency at 1 year was 78.9 % which was statistically significant when compared with the performance goal of 66 %. Primary safety endpoint at 1 year was freedom from death, target lesion revascularization (TLR) , or amputation in the index limb and was reported at 99.2 %, again comparing favorably with the performance goal (88 %). At VIVA 2014, 3-year follow-up results were reported [33]. TLR rates were 89 %, 84 %, and 82 % at 1, 2, and 3 years’ follow-up. Post hoc analysis also revealed differences in primary patency varying with the deployed stent length. Nominally deployed stents (i.e., post-deployment stent being within ±10 % of labeled length) had a higher patency than those deployed elongated (i.e., greater than their labeled length). Of note, during the 3-year follow-up, there was only one case of stent fracture .
Stent Grafts
The role of stent grafts in the treatment of peripheral vascular disease of the lower limb has been extensively evaluated, yet consensus about its role is difficult to achieve. The stent grafts (also known as covered stents, PTFE vascular prosthesis, etc.) were initially used for percutaneous interventions for aneurysms and pseudoaneurysms as well as to deal with complications relating to endovascular interventions [34], though later were also extensively evaluated for primary use in PAD especially femoropopliteal and aortoiliac disease . The main advantage of the covered stent is that the expanded polytetrafluoroethylene (ePTFE) graft material lining the stent serves to limit neointimal hyperplasia. The stent can also cover friable lesions potentially limiting distal embolization [34]. There are however a number of potential disadvantages which include problems with edge restenosis, higher rates of thromboses in the covered stents, and potential coverage of side branches or collaterals in the vascular bed, which can potentially worsen symptoms if the stent graft fails [35]. Almost all experience in the femoropopliteal vasculature comes with the Viabahn (formerly Hemobahn; W. L. Gore and Associates, Flagstaff, AZ) endoprosthesis [36–40] which is FDA approved for this indication. The Viabahn stent consists of a self-expanded nitinol stent encapsulated in ePTFE and more recently has undergone modifications to the device including heparin-bound luminal surface to reduce device thrombogenicity [34].
Saxon and colleagues reported on a randomized, premarket approval, multicenter trial of the Viabahn endoprosthesis compared to the PTA for treatment of symptomatic SFA PAD [41]. A hundred patients were assigned to the PTA and 97 to the stent-graft arm, with about 11 % of total patients having CLI. Immediate technical success was substantially higher in the stent graft group as compared to the PTA (95 % vs 66 %) and the 1-year primary patency was also reported to be higher in the stent-graft group (65 % vs 40 %).
Subsequently, further research accumulated and stent grafts were also compared with bypass grafts. Kedora et al. reported a randomized controlled trial (RCT) in which stent grafts were used in 40 patients (50 limbs), with another 40 patients (50 limbs) having open surgical femoral above-knee popliteal bypass [42]. Proportion of the study sample with CLI was 26 %. At median follow-up of 18 months, no significant difference was present between stent-graft and surgical bypass arms in terms of primary or secondary patency. Two-year and later 4-year follow-up on these patients still showed no difference between stent grafts and surgical bypass (59 % vs 58 % primary patency at 4 years) [43, 44]. This trial, thus, gave some evidence that stent grafts may be equal to surgical bypass for femoropopliteal disease.
In order to evaluate expanded indications for the use of stent grafts, particularly in TASC I C and D lesions, the GORE VIABAHN Endoprosthesis versus Bare Nitinol Stent in the Treatment of Long Lesion Superficial Femoral Artery Occlusive Disease (VIBRANT) trial was conducted to compare the Viabahn-covered stent with bare-nitinol stent in TASC I C and D lesions [45]. At 3-year follow-up, there was no difference in primary patency for the Viabahn stent as compared to the BMS (24.2 %; 95 % CI, 12.2–38.5 % vs 25.9 %; 95 % CI, 10.3–45.0 %). Even though higher-assisted primary and secondary patency rates were achieved in both arms, no additional benefit was demonstrated with the use of the stent graft in such cases (BMS had significantly higher-assisted primary patency, no difference in rates of secondary patency between the two arms).
Following this, the heparin-bonded Viabahn was studied in a single-arm multicenter registry, the Gore Viabahn Endoprosthesis with Heparin Bioactive Surface in the Treatment of Superficial Femoral Artery Obstructive Disease (VIPER) study [46]. One hundred and thirteen patients with 119 limbs were included in this trial, with about 61 % TASC II C and D lesions and 12 % with CLI. The mean lesion length was 19 cm. Primary patency rate at 12 months’ follow-up was 73 %, with secondary patency rate of 92 %. A logistic regression analysis done to identify factors affecting loss of patency identified age and oversizing at the proximal end were independently associated with loss of patency.
The efficacy of the Viabahn stent graft in long femoropopliteal lesions was retested in the Viabahn Endoprosthesis with PROPATEN Bioactive Surface Versus Bare Nitinol Stent in the Treatment of Long Lesions in Superficial Femoral Artery Occlusive Disease (VIASTAR) trial [47]. A total of 141 patients with femoropopliteal lesions 10–35 cm in length were randomized 1:1 in the Viabahn and BMS subgroups. About 16 % of the patients presented as CLI. Primary patency rates at 1 year showed a trend toward benefit for the stent graft in the intention-to-treat (ITT) analysis (70.9 %; 95 % CI, 0.58–0.80 vs 55.1 %; 95 % CI, 0.41–0.67); this difference became significant when analyzed as treatment-per-protocol (TTP) (78.1 %; 95 % CI, 0.65–0.86 vs 53.5 %; 95 % CI. 0.39–0.65). No differences however were present in TLR rates. In lesions >20 cm, however, stent grafts were significantly superior in terms of primary patency to the BMS (71 % vs 37 %).
Drug-Eluting Stent
Drug-eluting stents (DES) have been the mainstay of percutaneous revascularization strategies for coronary arteries. With the incidence of restenosis seen in peripheral artery disease interventions, DES technologies have been specifically developed to respond to the specific needs of the femoropopliteal as well as below-the-knee arterial revascularization.
Review of the Basics
Ideal Drug to Inhibit Restenosis
Complex regulation and our incomplete understanding of the cell cycle justify some of the difficulties in developing appropriate drug therapy to inhibit neointimal hyperplasia and restenosis (Fig. 35.1). The presence of redundant systems of promoting neointimal growth makes it unlikely that drugs with a highly specific target and mechanism will be effective inhibitors or restenosis [6]. An ideal drug would act locally, without systemic effects, and selectively inhibit the activation of vascular smooth muscle cells while allowing normal progression of re-endothelialization [10]. The base metal of the stent and polymer aiding in drug adherence must both be inflammatorily unreactive. This was especially problematic with early stent materials and copolymers that stimulated restenosis due to their pro-inflammatory or prothrombotic properties [10, 12, 13, 48].


Fig. 35.1
Simplified diagrammatic representation of the cell cycle with stages affected by sirolimus and paclitaxel identified. G gap phase, S synthesis phase, M mitosis phase
Overview of Candidate Drugs
Recovery from endovascular injury parallels early tumor development and the normal injury repair process [7, 13]. As such, immunosuppressant and antitumor drugs have been a major area of focus in trying searching for locally deliverable, effective agents in preventing neointimal hyperplasia [7, 13]. Paclitaxel and sirolimus are currently the drugs with the most encouraging results for usage in peripheral arteries.
Paclitaxel
In the late 1960s, paclitaxel was first isolated from the bark of the Pacific yew tree, Taxus brevifolia, and has since become an important chemotherapeutic agent. The mechanism of action for paclitaxel, and others in the taxane family of drugs, is to inhibit cellular division by preventing breakdown of tubulin. Taxanes accomplish this by binding with high affinity to the β subunit of assembled tubulin while poorly binding to the soluble tubulin components. Polymerized tubulin with bound paclitaxel is stabilized against degradation [49, 50]. By interfering with normal tubulin activity, multiple regulatory pathways in the cell cycle are disrupted creating a mitotic block at the transition between G2 and M (Fig. 35.1) along with disturbing signal transduction and migration of smooth muscle cells [6, 13, 50]. The lipophilic characteristics of paclitaxel make it ideally suited for absorption into damaged vascular tissue [6, 51].
Sirolimus (Rapamycin) and Rapamycin Analogs
In the 1970s, sirolimus was isolated from the fermentation products of the soil bacteria Streptomyces hygroscopicus on Easter Island. Sirolimus is a macrocyclic lactone with weak antibiotic and was initially being developed as an antifungal but was found to strongly suppress the immune system. Sirolimus and a structurally similar macrolide, tacrolimus (FK506), share an intracellular receptor; however, there functions differ. Both bind to FK-binding protein 12 (FKBP12), forming a tertiary complex, but the complexes then interact with different regulatory mechanisms. The tacrolimus–FKBP12 complex inhibits calcineurin halting the cell cycle at the G0/G1 transition, whereas sirolimus–FKBP12 prevents the cytokine-mediated G1/S transition (Fig. 35.1) [9].
Inside the cell, the mechanism of inhibition begins with the complexing of sirolimus to FKBP12 and their interaction with the mammalian target of rapamycin (mTOR). The kinase activity of mTOR is essential in proper regulation of the cell cycle. Some of its targets with regulatory roles are 70 kd S6 protein kinases (regulators of translation that are critical to the cell cycle), the eukaryotic initiation factor eIF4F, G1-controlling cyclin-dependent kinases, and p27 (reduces phosphorylation of Rb) [6, 9, 12, 13, 52]. The combined effect on inhibiting mTOR is to arrest the cell cycle in G1. Sirolimus has additional inhibition of cytokine signals that would activate stimulated T-cells and promote migration of smooth muscle cells. One suggested reason for how sirolimus halts the cell cycle is that it induces a cellular survival process reminiscent to nutrient deprivation resulting in autophagy where the G1/S transition is prevented by the release of intracellular amino acids [53].
Several stents using analogs and derivatives of sirolimus were developed, and two were approved for use in coronary artery disease. These second-generation drug-eluting coronary stents used everolimus (a sirolimus analog) and zotarolimus (a sirolimus derivative), both of which are more lipophilic than sirolimus, increasing their cellular uptake [54].
Femoropopliteal Disease
Initial enthusiasm for DES in femoropopliteal disease was marred with the less than ideal results for sirolimus and everolimus DES technologies. The Sirolimus -Coated Cordis Self-expandable Stent (SIROCCO) I trial was the first study, which utilized a sirolimus-eluting stent (SES) in above-the-knee lesions [55]. In this study, participants were randomized to the sirolimus-coated shape-memory alloy-recoverable technology (S.M.A.R.T.) stent (Cordis, Johnson & Johnson, Warren, NJ) or the same stent without the sirolimus coating. The study was designed as a multicenter, randomized controlled trial, in which 36 patients have obstructive, de novo, native, or restenotic lesions with a diameter stenosis of >70 % in the superficial femoral artery (SFA). The primary endpoint of the study, namely, the mean in-stent percent stenosis as detected by quantitative angiography at 6 months, was 22.6 % as compared to 31 % for the uncoated stent group. Though the difference was nonsignificant, there was a trend toward benefit for the SES group. The occlusion and in-stent restenosis rates (ISR) were also 0 % for the SES as compared to 5.9 and 23.5 %, respectively, in the uncoated stent group. Improvements in the Rutherford–Becker class (RBC) and ankle-brachial indices (ABIs) were uniformly seen in both groups at 6 months.
The findings of this study were expanded upon by the Sirolimus -Coated Cordis SMART Nitinol Self-expandable Stent for the Treatment of Obstructive Superficial Femoral Artery Disease (SIROCCO) II trial which randomized 57 patients in the sirolimus -coated SMART stent and uncoated stent groups [56]. Inclusion criteria were similar, and the study demonstrated that there was no difference in the primary endpoint of in-stent mean luminal diameter between the sirolimus-coated and uncoated groups (4.94 mm ± 0.69 and 4.76 mm ± 0.54). There was a trend toward reduction in stent late lumen loss in DES as compared to the uncoated stent which was however nonsignificant. Follow-up at 24 months demonstrated that improvement in ABI and freedom from claudication were maintained in both groups with no difference seen in the restenosis rates (23 % in the DES vs 21 % in the uncoated group) [57]. It has been hypothesized that the failure of the sirolimus-coated SMART stent is due to the lower-dose concentration of sirolimus on the stent as compared to its coronary counterpart (90 mcg/cm2 vs 140 mcg/cm2 of stent area) or due to its rapid eluting kinetics (7 days vs 30 days) [58], though this potential problem has not been studied further.
It seems that mTOR inhibitors did not have much success overall in PAD of above-the-knee vessels . In another single-arm study, the first-in-human Superficial Femoral Artery Treatment with Drug-Eluting Stents (STRIDES) study, everolimus -eluting stents were evaluated for the treatment of de novo or restenosis lesions in the femoral and proximal popliteal arteries [59]. All patients had chronic PAD with RBC of 2–5 (17 % of patients having CLI). A total of 104 patients with 106 lesions were enrolled in 11 European centers. Ninety-eight percent device success rate was immediately achieved and 6-month rates of ISR remained at 14 %. Six-month primary patency rates were 94 % ± 2.3 dropping to 68 % ± 4.6 however at 12 months. These are very much comparable to historical rates observed for bare-nitinol stents ; hence, this study failed to create a role for everolimus DES in the treatment of femoropopliteal PAD lesions.
A breakthrough, however, came in the form of the paclitaxel -eluting stent, the Zilver PTX (Cook Medical, Bloomington, IN). The Zilver PTX trial was a randomized controlled trial designed to compare percutaneous transluminal angioplasty (PTA) with the Zilver PTX DES in treatment of symptomatic de novo or restenotic lesions of above-the-knee femoropopliteal artery segment [60]. Since PTA often fails acutely, a secondary randomized assignment post-PTA failure compared assignment of the Zilver PTX with the Zilver bare-nitinol stent (Cook Medical, Bloomington, IN). Four hundred seventy-nine patients (238 in the PTA group and 241 in the DES group) were enrolled in this multicenter trial. Nine percent of the total patients were with CLI. Due to acute PTA failure, secondary randomization was done in 120 patients which were divided in the provisional BMS (59 patients) and provisional DES (61 patients) groups. The primary endpoints were 12-month event-free survival and primary patency rates. Paclitaxel DES showed significantly improved event-free survival (90.4 % vs 82.6 %, respectively) and primary patency (83.1 % vs 32.8 %). Of note, the patency rates with optimal PTA (defined as the group excluding acute PTA failure) and PTA with provisional stent (again not counting acute PTA failure as loss of patency) also remained at only 65.3 and 73.4 %; thus, paclitaxel DES patency rates, regardless, remained significantly superior. Secondary outcomes showed that provisional DES was significantly superior to provisional BMS in the acute PTA failure group (90 % vs 73 %, respectively). These differences have remained at 2 years follow-up as well [61] suggesting that the Zilver PTX does not demonstrate the adverse late catch-up phenomenon seen in STRIDES and SIROCCO studies. The trial, however, was not designed to evaluate the stent’s usefulness in the CLI subgroup.
The Zilver PTX stent was also examined in TASC II C/D lesions. The Zilver PTX single-arm study was a prospective, multicenter study involving 787 patients with de novo or restenotic femoropopliteal lesions >50 % stenosis, with a total of 900 lesions treated with the Zilver PTX stent [62]. Among these, a subgroup analysis was performed on 135 lesions >15 cm corresponding to the TASC II C/D subgroup lesions, with 84 % of these lesions having total occlusion. The primary patency rate for these lesions remained at 77.6 % at 12 months with freedom from target lesion revascularization (TLR) at about 85 %. Twenty-one major adverse events (MAEs) were observed in this population including two deaths, while the remaining being TLRs. Stent fracture rates remained at 2 %. Compared with historical surgical bypass patency rates of 85 % for venous bypass and 67 % for prosthetic grafts, the authors concluded that the Zilver PTX offers a viable option for these complex lesions in patients with comorbidities or other factors precluding surgical bypass. This, however, remains to be explored in further randomized controlled studies.
Infrapopliteal Disease
In contrast with above-the-knee disease, drug-eluting stents found early application and use for below-the-knee disease. Coronary stents have been studied in the tibial vessels with significant success reported in smaller studies [63–65]. These have been subsequently replicated in RCTs as well. Among the nonrandomized studies, sirolimus for below-the knee (SiroBTK) was a nonrandomized, single-arm study using the Cypher sirolimus-eluting stent (SES) (Cordis, Johnson & Johnson, Warren, NJ) for treatment of patients with severe intermittent claudication or critical limb ischemia (CLI) due to infrapopliteal PAD [66]. One hundred and six SES were implanted in 62 arteries in 30 patients. The overall primary patency rate at 1 year measured by TLR was 97 % with the event-free survival calculated at 92.5 %. Eighty-seven percent of the patients were CLI prior to treatment, whereas at 12 months the number had decreased to 3.7 %. Recurrence of CLI or failure to heal chronic ulcers was often due to new, de novo lesions. No adverse effects as a direct consequence of the stent were observed.
Similarly, the PReventing Amputations using Drug eluting StEnts (PaRADISE) trial was another single-arm, nonrandomized trial designed to study the utility of DES in the treatment of BTK lesions in patients with CLI [67]. Interestingly, there were no exclusion criteria as everyone, even patients with concomitant above-the-knee lesions, was treated with DES. The CYPHER SELECT SES and the Taxus paclitaxel -eluting stents (PES) (Boston Scientific, Maple Grove, MN) were used in this study. One hundred and six patients (118 limbs) received a total of 228 stents. The 3-year cumulative risk of amputation was 6 ± 2 % with survival rates of 71 ± 5 % and amputation-free survival of 68 ± 5 %. Overall RC scores 4 and 5 did significantly better than score 6 (both improving to 97–98 % vs 86 % for score 6 on follow-up). These results compared favorably by the comparison with historical controls from the TASC II document and the BASIL study as reported by the authors [5, 68].
Among randomized controlled trials (RCT) , earlier trials were rather limited in their scope for examining the effectiveness of DES for the treatment of below-the-knee lesions. Falkowski et al. presented the first trial which examined the role of Cypher stent compared with a BMS counterpart in reducing the restenosis rates in crural arteries at 6 months’ follow-up. Fifty patients were randomized 1:1 in the two groups (32 % with CLI), and at 6 months, restenosis rates were significantly lower in the SES group (16 % vs 76 %) [69].
The ACHILLES trial was a multicenter randomized controlled trial comparing the efficacy of SES with PTA in the treatment of de novo as well as restenotic infrapopliteal PAD lesions using angiographic 1-year binary restenosis rates as endpoint [70]. Two hundred patients were enrolled in the trial with 99 randomized to the CYPHER SELECT arm. The primary endpoint was reached by 22 % of SES patients as compared to 42 % in the PTA arm (p = 0.019). The difference was even more significant in diabetics (17 % vs 53 %). No difference was however there in rates of death, target lesion revascularization rates, or index limb amputation rates though there was a trend favoring the SES arm. This trial, like others, suggests that long-term patency is improved with DES, but may not be necessary for initial limb salvage.
Another SES, Yukon (Translumina, Hechingen, Germany), was compared with BMS in the YUKON Below-the-Knee (YUKON-BTK ) randomized, double-blind trial [71]. A total of 161 patients (82 randomized to the SES arm) were enrolled with 47 % having CLI. The primary endpoints of 1-year freedom from TLR significantly favoring the SES arm (44 % in the SES arm vs 19 % in the BMS) with primary patency rates at 1 year significantly higher in the SES subgroup (81 % vs 56 %). The hazard ratio for restenosis calculated by the authors for the BMS was 3.2 (95 % confidence interval 1.5–6.7), with significant improvements in the RBC and ABI observed. Subgroup analysis in the CLI patients showed benefit from SES for primary patency but did not reach statistical significance (75 % vs 56.5 %). Again, no significant differences were seen in median RBC score improvement, healing of chronic ulcers at baseline, or improvement in ABI among the two subgroups, though there was a trend favoring the SES. Long-term follow-up to about 3 years for this trial reported the SES showed significantly higher event-free survival than the BMS (66 % vs 44 %) with significantly improved RBC scores for the SES as compared to the BMS groups [72]. The long-term event-free survival benefit becomes even more significant in the CLI subgroup (58 % vs 32 %). These late findings becomes all the more important as 1-year results did not show statistical difference between SES and BMS arms in the CLI subgroup, thus indicating that in the long run, SES may be the better option for revascularization in CLI patients.
The Drug Eluting Stents in the Critically Ischemic Lower Leg (DESTINY ) compared the everolimus -eluting XIENCE V (Abbott Vascular, Santa Clara, CA) with bare-metal stents for the treatment of de novo tibial arterial lesions in patients with chronic CLI [73]. A total of 140 patients were enrolled in this multicenter trial with 74 in the XIENCE V arm. Primary patency rates were significantly favorable for the XIENCE V arm (85 % vs 54 %) with significantly greater freedom from TLR also achieved (92 % vs 65 %); however, there was no difference obtained in survival rates or improvement in the Rutherford–Becker class between the two groups. This was attributed to the authors to the lack of power in the study design to test these hypotheses.
A meta-analysis of these trials by Fusaro et al. reported that drug-eluting stents reduce the rates of TLR (odds ratio (OR) 0.31; CI 0.18–0.54), restenosis (OR 0.25; CI 0.15–0.43), and amputation (OR 0.50; CI 0.26–0.97) significantly with no impact on the rates of death or improvement in the RBC score as compared to control therapy (balloon angioplasty or BMS implantation ) [74]. It must be noted that PES were not used in any of the RCTs; thus, their role in below-the-knee arterial lesions remains undefined. An older meta-analysis of nonrandomized studies by Biondi-Zoccar and colleagues does suggest that for BTK lesions, SES performed significantly better than PES in terms of primary patency (93 % vs 30 %) and target vessel revascularization (TVR) (7 % vs 31 %) [75]. These results, however, must be used with caution as the studies included generally had low sample sizes and only one study which examined the role of PES was included in this analysis.
Beyond Stents: New Technologies for Endovascular Interventions
As techniques/technologies have continued to advance, the limitations of PTA continue to become more evident, mostly secondary to the inability to address the immediate technical complications leading to procedural failure. In addition to the advent of stents, a number of balloon angioplasty technologies have been developed to address these major technical complications. Such advances include cryoplasty, cutting balloons , brachytherapy (not included in this review), and drug-eluting balloons.
Cryoplasty
The lone cryoplasty system available today, PolarCath (Boston Scientific, Natick, MA) catheter, utilizes a low-pressure (~8 atm) double-balloon system, which in addition to applying compressive pressure causes a cold-induced injury to the lesion. The application of the 30-s cryotherapy cools the vessel wall to roughly −10°C; the physiologic effects of cooling are believed to create a uniform rigidity in the vessel wall altering both collagen fibrils and inducing smooth muscle apoptosis. The PolarCath system thus allows for a uniform mechanical load to be achieved between the vessel wall balloon interface, which is believed to decrease significant amounts of plaque dissection, vessel recoil, and intimal hyperplasia.
Femoropopliteal Disease
The published results of the cryoplasty safety registry of noncomplex femoropopliteal lesions showed a 10-month patency rate of 82 % in the 102 patients studied [76, 77]. The COLD study was a prospective single-center trail to evaluate the efficacy and safety of cryo- versus conventional angioplasty in focal popliteal artery lesions [78]. Eighty-six patients were enrolled and randomized into each arm (46 in conventional PTA arm and 40 in cryo arm); patients were equally matched with a mean age of 72 and were assessed for a primary endpoint of target lesion patency and secondary endpoint of treatment success (without need for subsequent stenting). Both arms were followed at 3, 6, 9, and 15 months with duplex ultrasound. Initial success rate was 35 % in cryoplasty versus 54 % in the conventional PTA, with no statistically significant difference in rate of dissection, need for stent placement secondary to dissection, or target lesion patency at 9 months’ follow-up. Despite a lower primary anatomic success rate in the cryoplasty group, there was, however, a nonsignificant trend toward greater patency in the cryoplasty arm at 9 months’ follow-up (79.3 % ± 7.5 vs 66.7 % ± 8.1 (p = 0.14)).
Similar results were published by Spiliopoulos et al. in another prospective randomized controlled single-center trial comparing cryoplasty versus conventional PTA of femoropopliteal artery lesions in diabetic patients, with CLI being present in 41.4 % of the cryoplasty arm and 38.7 % of the conventional arm [79]. Initial technical success (<30 % stenosis and no need for adjunctive stenting) was 58 % in cryoplasty versus 64 % in conventional arm (p = 0.29), a nonsignificant trend toward increased binary restenosis (HR 1.3, 95 % CI 0.6–2.6, p = 0.45) and no statistically significant differences in patient survival or limb salvage at 3 years when compared to a Kaplan–Meier model. There were however significantly lower rates of primary patency (HR 2.2, 95 % CI 1.1–4.3) and higher repeat intervention secondary to recurrent symptoms in the cryoplasty group (HR 2.5, 95 % CI 1.2–5.3).
Infrapopliteal Disease
Das et al. published the 12-month follow-up results, from the 16-center prospective BTK Chill trial which included 108 patients to evaluate the efficacy of cryoplasty for below-the-knee (BTK) occlusive disease in patients with CLI [80]. Pts were followed at 1, 3, and 12 months for acute technical success (≤50 % stenosis and continuous in-line flow to the foot) and absence of major amputation at 6 months as primary endpoints and secondary endpoints of cryoplasty-associated complications and absence of major amputations out to 12 months’ follow-up. Immediate technical success was achieved for 97.3 % of threatened limbs, with major amputation avoided in 93.4 % and 85.2 % of patients at 6 and 12 months, respectively. There was only one clinically significant dissection, two patients with residual stenosis >50 %, three patients requiring adjunctive stenting, and only 21 % of patients requiring subsequent intervention at 1 year. Cryoplasty appears to be a reasonable, safe, and effective method of treating infrapopliteal disease in patients with CLI.
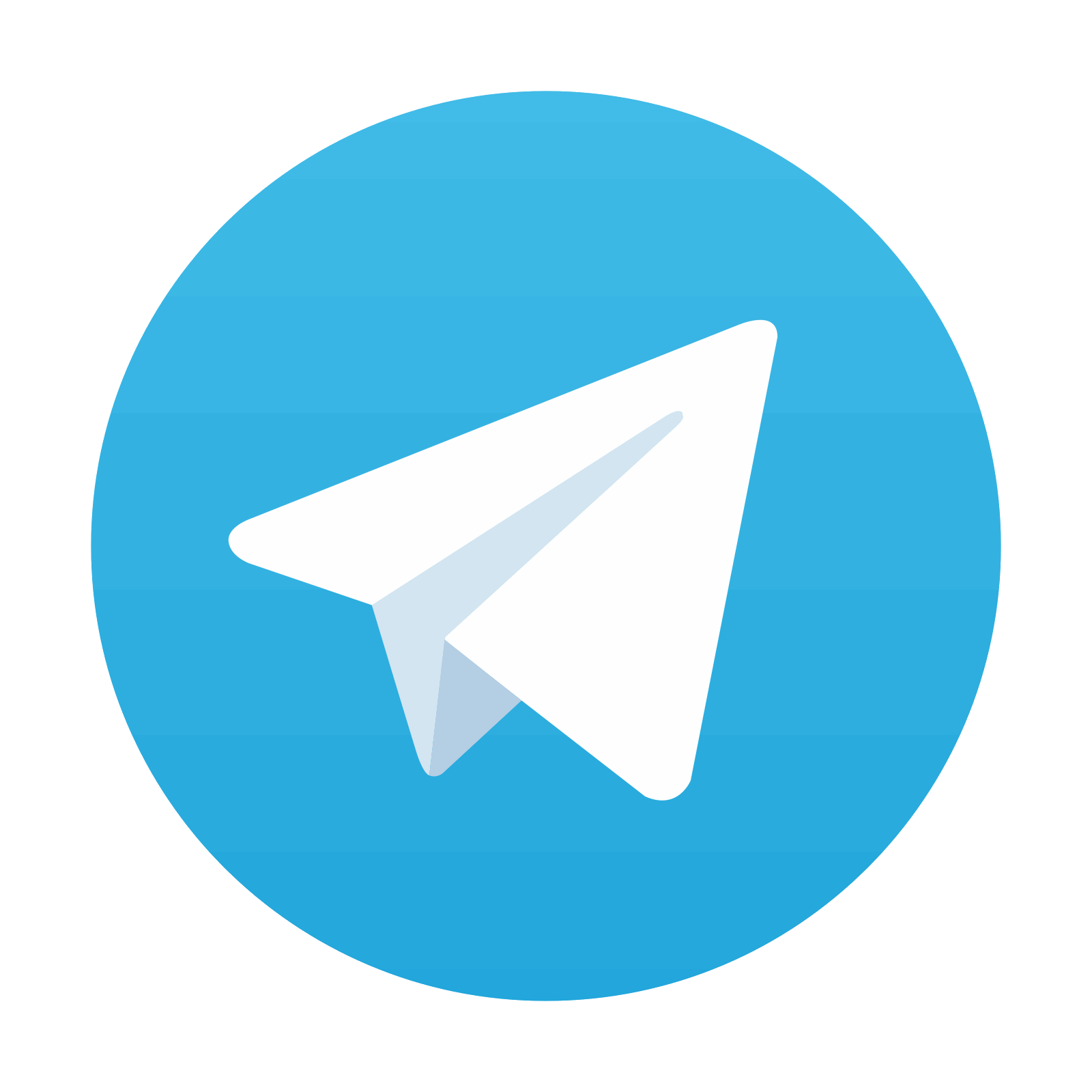
Stay updated, free articles. Join our Telegram channel

Full access? Get Clinical Tree
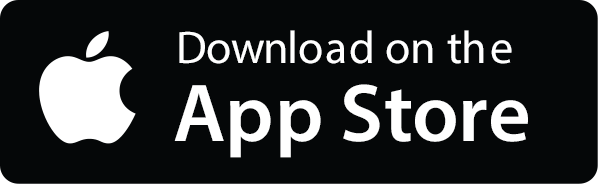
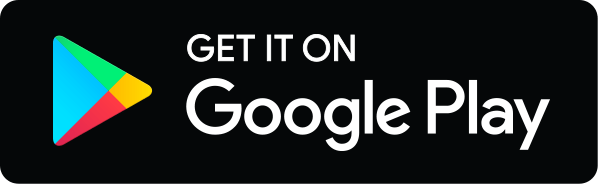
