A 52-year-old man with hypertension for the past decade presented with poorly controlled blood pressure despite increasing doses of 4 antihypertensive medications including a diuretic, angiotensin-converting enzyme inhibitor, β-blocker, and calcium channel blocker. Office-based blood pressure measurements remained in the 160 to 180/100 to 110 mm Hg range despite escalation of medical therapy over a several-month period. Serum and urine testing revealed no evidence of hyperaldosteronism or pheochromocytoma. Renal artery ultrasonography demonstrated moderately elevated systolic flow velocities (295 cm/s) near the ostium of the left renal artery with an elevated renal resistive index; however, renal artery angiography was normal (Figure 38-1). What other treatment options might be considered for this patient with resistant hypertension?
In the United States, based on the most recent results of the National Health and Nutrition Examination Survey (NHANES), 29.1% of adults (>70 million individuals) have hypertension.1 Among them, 12.2% are estimated to have resistant hypertension defined as office blood pressure ≥140 mm Hg systolic and/or ≥90 mm Hg diastolic while being treated with ≥3 antihypertensive drugs, including a diuretic.2 Progression of previously controlled hypertension despite treatment is also common, with an incidence of resistant hypertension of 1.9% within a median of 1.5 years from initial treatment.3 When resistant, hypertension is associated with a substantially increased likelihood of cardiovascular events and worse prognosis (Figure 38-2).4 Renal denervation (RDN) and endovascular carotid baroreceptor modulation are novel strategies to improve blood pressure control in this challenging population and are currently being evaluated in clinical studies. The principles of these techniques and clinical experience to date will be reviewed in this chapter.
Figure 38-2
Cumulative event rates (all-cause mortality, myocardial infarction, or stroke) among 17,190 patients with coronary artery disease and resistant, uncontrolled, or controlled hypertension (HTN) enrolled in the International Verapamil SR-Trandolapril (INVEST) Study. (Reproduced, with permission, from Smith SM, Gong Y, Handberg E, et al. Predictors and outcomes of resistant hypertension among patients with coronary artery disease and hypertension. Journal of hypertension 2014;32:635-643.)

The sympathetic nervous system plays a fundamental role in blood pressure control and is primarily responsible for acute changes in blood pressure via (1) extensive innervation of capacitance vessels, which alter arterial resistance in response to norepinephrine release from nerve endings; and (2) adrenal medullary stimulation, leading to increased epinephrine production and release, resulting in increased cardiac contractility, and increased heart rate and cardiac output (Figure 38-3). Chronic increases in blood pressure related to augmentation of central sympathetic drive result from changes in intravascular volume and pathologic changes in the “resistance arteries” stemming from hormonal alterations, particularly in the renin-angiotensin-aldosterone axis.5 Efferent renal sympathetic nerve stimulation results in renin release by the kidney, tubular sodium reabsorption, and increased renal vascular resistance, all of which serve to raise blood pressure. Renal afferent nerves from mechanical-sensitive receptors in the renal pelvic wall and chemosensitive receptors in the renal interstitium increase central sympathetic output via hypothalamic integration.
Figure 38-3
Renal sympathetic nerve activity influences blood pressure regulation through the kidney via multiple pathways. RAAS renin-angiotensin-aldosterone system. (Reproduced with permission from Veelken R, Schmieder RE. Renal denervation: Implications for chronic kidney disease. Nat Rev Nephrol 2014;10:305-313.)

Therapeutic approaches aimed at modification of renal sympathetic innervation as a means to control hypertension date to the early 20th century. Surgical sympathectomy, first performed in the 1920s, was shown to reduce blood pressure by augmenting natriuresis, decreasing renal renin release, and modulating central sympathetic drive.6 Although at times quite effective, this invasive surgical procedure was associated with inconsistent reductions in blood pressure and was also limited by surgical mortality and postoperative complications including severe orthostatic hypotension, gastrointestinal disturbances, erectile dysfunction, and urinary and fecal incontinence.7 When medical sympathectomy became available in the 1940s via ganglion-blocking agents, the surgical procedure was abandoned. With the increasing burden of resistant hypertension and less invasive access to renal sympathetic nerves resulting from improved technology, the novel catheter-based strategy of RDN has led to a resurgence of interest in therapeutic sympathectomy over the past decade.
Afferent and efferent sympathetic nerve fibers both follow the course of the renal artery, extending along the vessel adventitia from the aorto-ostium into the kidney. A recent study demonstrated evidence that fewer nerves are present adjacent to the distal compared to the proximal renal artery; however, the depth of the nerve fibers relative to the vessel lumen decreases as the nerves course distally, potentially rendering the distal fibers more accessible to energy delivered from within the vessel lumen8 (Figure 38-4). Therefore, preferential ablation of the distal renal artery segments during RDN may improve the effectiveness of therapy but at a potential increased risk of injury to the kidney.
Figure 38-4
Depiction of the course depth, density, and distribution of sympathetic nerve fibers as they course adjacent to the renal artery (RA). (Reproduced, with permission, from Sakakura RH, Ladich E, Cheng Q et al. Anatomic Assessment of Sympathetic Peri-Arterial Renal Nerves in Man. J Am Coll cardiol. 2014;64:635-643.)

With current systems, vascular access is typically obtained in the femoral artery, and a 5-Fr ablation device is advanced to the renal artery through a 6-Fr guide catheter. Renal angiography is performed to assess anatomic suitability for the procedure. Anatomic exclusion criteria in the Symplicity-HTN 3 trial included >50% renal artery stenosis, renal artery aneurysm, prior renal artery intervention, multiple renal arteries, renal artery diameter <4 mm, or treatable segment <20 mm in length.
Under fluoroscopic guidance, the ablation catheter is placed in the distal segment of the renal artery, and radiofrequency energy is delivered in a helical pattern from the distal artery to the ostium (Figure 38-5). Bilateral renal artery ablation is recommended, and the procedure takes about 15 minutes per renal artery. With the first-generation Symplicity catheter (Medtronic, Minneapolis, MN), up to 4-mm depth of tissue from the tip of the catheter is destroyed by the heat produced during each ablation. Factors required to provide safe and effective treatment include good catheter apposition to the vessel wall and appropriate duration and level of power applied for each ablation. Injury to the endothelium is mitigated by cooling provided by native renal blood flow and by saline irrigation delivered via some of the available catheters. Nitrates and nondihydropyridine calcium channel blockers are given to avoid vasospasm. Activation of C fibers in the adventitia during ablation produces significant pain, necessitating conscious sedation and opioid analgesia. Second-generation catheter designs currently being used in ongoing clinical trials involve multiple emitters mounted around an expandable catheter or on a balloon that can be inflated in the renal artery, permitting simultaneous ablation of several foci with shortened procedure time (Figure 38-6). Newer catheter designs also ensure good electrode apposition to the vessel endothelium, a circumferential ablation pattern, and uniform energy delivery.
Figure 38-6
Various catheter designs for RDN. (A) Medtronic Symplicity control console (Used with permission by © Medtronic 2016); (B) Medtronic Symplicity Spyral Multi-Electrode catheter (Used with permission by © Medtronic 2016); (C) Boston Scientific Vessix System (Image provided courtesy of Boston Scientific. © 2016 Boston Scientific Corporation or its affiliates. All rights reserved); and (D) St. Jude Medical EnligHTN Multi-Electrode System (EnligHTN and St. Jude Medical are trademarks of St. Jude Medical, Inc. or its related companies. Reproduced with permission of St. Jude Medical, ©2016. All rights reserved).

Early single-arm studies of RDN among patients with resistant hypertension demonstrated procedural safety and brought about much enthusiasm in the medical community regarding potential efficacy with respect to blood pressure control. The concept of RDN, however, suffered a setback in 2014 with publication of the large randomized controlled Symplicity-3 trial, which failed to demonstrate superiority of denervation compared to medical therapy.9 As will be discussed, the Symplicity-3 results led to reassessment of several fundamental technical and trial design issues surrounding RDN, and multiple new trials of RDN are currently enrolling patients.
Multiple nonrandomized clinical studies published since 2009 examined the safety and putative efficacy of RDN among individuals with resistant hypertension and yielded uniformly encouraging results. A large “real-world” registry of nearly 1000 patients is also available.10 These studies are summarized in Table 38-1, and the following general conclusions can be made:
RDN appears safe from a technical standpoint. Major adverse procedural events (eg, death, myocardial infarction, stroke) were not reported in the listed trials, and minor procedural complications (eg, access-related vascular events, renal artery dissection) were uncommon. One concern that emerged was the potential for new renal artery stenosis after RDN. Although no stenosis of >70% was detected following RDN, among one cohort of 76 patients who underwent magnetic resonance imaging (MRI) or computed tomography (CT) scanning 6 months after the procedure, more than 30 instances of new renal artery stenosis or stenosis progression have been reported to date after RDN.11,12
RDN was consistently associated with significant blood pressure (BP) reduction at intermediate-term follow-up (typically 6 months). In most studies, compared to baseline, mean reductions of office-based systolic BP by 20 to 30 mm Hg and of diastolic BP in the 10 mm Hg range were observed following RDN.
Office-based BP readings, in almost all studies, showed greater improvement following RDN than 24-hour ambulatory BP readings (typically by a factor of 2- to 3-fold).
Patients with moderate degrees of hypertension demonstrated a lower absolute decline in BP following RDN than patients with more severe hypertension at baseline.10,13
A substantial percentage of patients (60%-87%) treated with RDN were termed “responders,” defined as having a ≥ 10 mm Hg drop in systolic BP following treatment.
Several issues were unanswered by these studies, including whether treated patients were truly medically refractory, the durability of the reduction in BP following RDN (only 1 study had follow-up beyond 1 year), and most importantly, whether the benefits of RDN would remain apparent if examined in the context of a rigorous randomized trial with a blinded control group.
Study | Design | Catheter System | No. of Patients | Follow-Up | BP Reduction (S/D, mm Hg)a | Responders (%) | Adverse Events and Comments | |
---|---|---|---|---|---|---|---|---|
Office | Ambulatory | |||||||
Symplicity-150,51 | Single arm | Medtronic Symplicity | 50 | 1 year 3 years | 27/17 32/14 | NR | 87 | 1 renal artery dissection, 1 access site pseudoaneurysm |
RAPID52 | Single arm | Covidien OneShot | 50 | 6 months 1 year | 20/8 22/8 | 11/6 | NR | No adverse events |
EnligHTN53,54 | 12 “matched” controls | St. Jude EnligHTN | 31 | 6 months 1 year | 26/10 27/11 | 10/6 7/4 | 74 | 1 new renal artery stenosis at 12 months |
REDUCE-HTN55 | Single arm | Boston Scientific Vessix | 146 | 6 months | 25/10 | 8/6 | NR | 1 new renal artery stenosis at follow-up |
ENCOReD56 | Multicenter registry | Medtronic Symplicity used in 90% of cases | 109 | 6 months | 18/7 | 6/4 | 60 | 8 access site hematomas, 3 patients with progression of renal artery stenosis |
Ewen et al57 | 10 selected controls | Medtronic Symplicity | 60 | 6 months | 26/7 | NR | 86 | BP also reduced during exercise following RDN |
Mahfoud et al58 | 17 selected controls | Medtronic Symplicity | 72 | 6 months | 22/8 | NR | 67 | MRI imaging: improved LV mass, LVEF and LV strain after RDN |
Mahfoud et al59 | 12 selected controls | Medtronic Symplicity and Flex | 100 | 6 months | 27/10 | NR | 83 | 2 access site pseudoaneurysms |
Ott et al13 | Single arm | Medtronic Symplicity | 54 | 6 months | 13/7 | 14/7 | 61 | No complications Enrolled patients with moderate resistant HTN (BP >140/90 mm Hg but <160/110 mm Hg) |
Ukena et al60 | Single arm | Medtronic Flex | 136 | 6 months | 28/10 | NR | 83 | 2 access site pseudoaneurysms |
Lambert et al11 | Single arm | Medtronic Symplicity | 76 | 6 months | 15/5 | 5/3 | 65 | No procedural complications |
Dores et al61 | Single arm | Symplicity, EnligHTN, or OneShot | 34 | 6 months | 22/9 | 5/2 | 82 | No procedural complications |
Global Symplicity Registry10 | Registry | Medtronic Symplicity | 998 | 6 months | 12/4 | 7/4 | 67 | 1 new renal artery stenosis at follow-up BP reduction greater among subset with “severe” HTN (office BP reduction 20/7 mm Hg) |
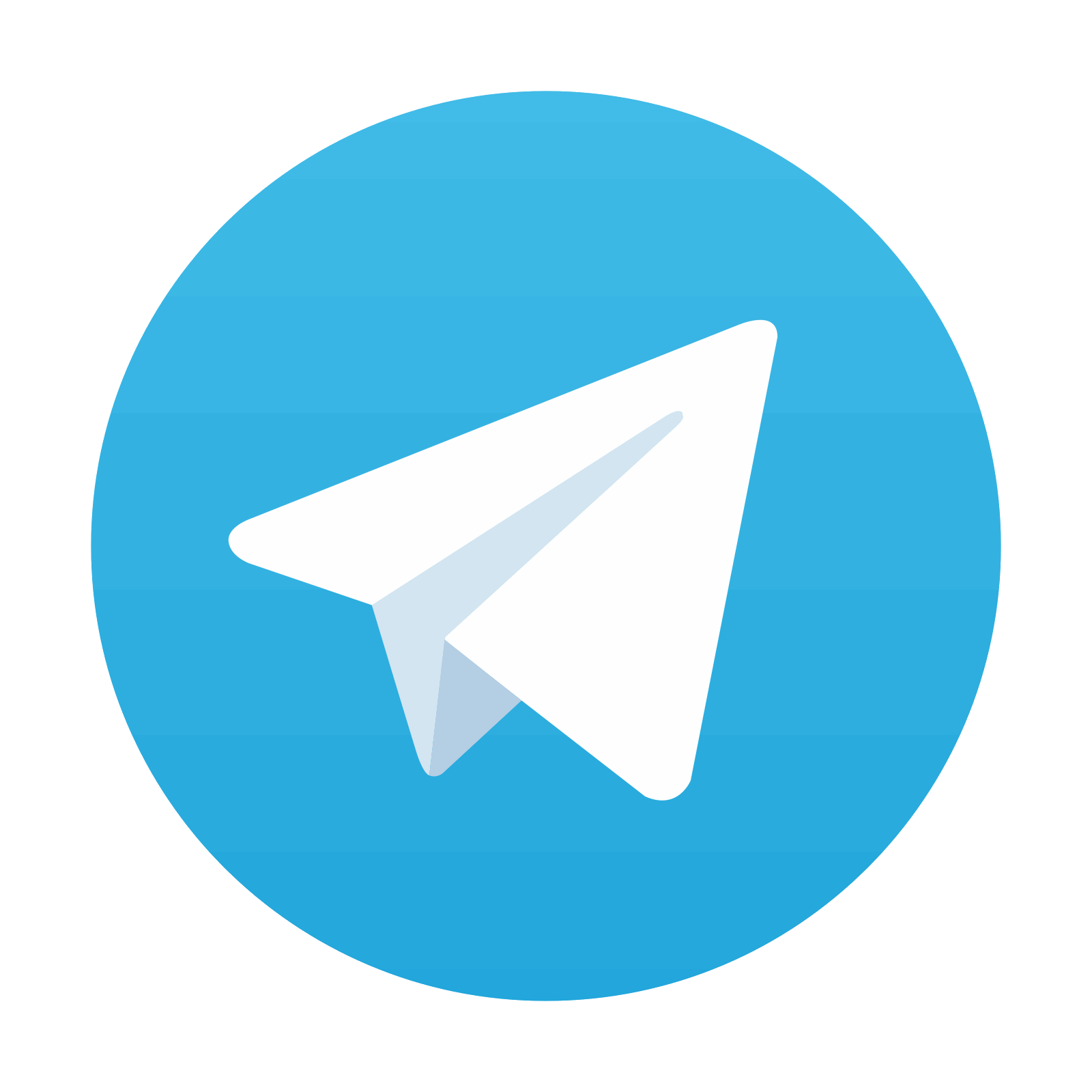
Stay updated, free articles. Join our Telegram channel

Full access? Get Clinical Tree
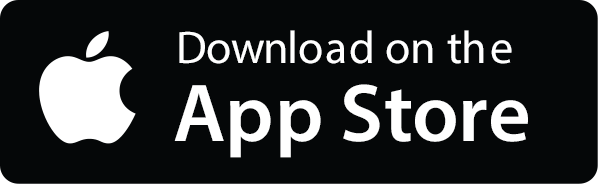
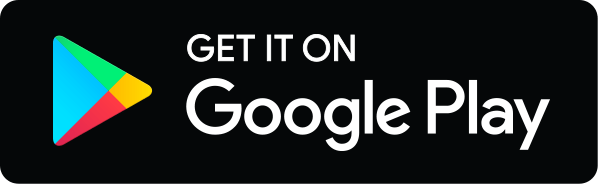
