Fig. 9.1
Classification of endothelial cell colonies. Endothelial cell colonies are isolated from mononuclear cell fractions of cord blood, peripheral blood circulation, or derived from endothelial cell cultures after single cell sorting. Mature, fully differentiated endothelial cells (ECs) are non-proliferating individual cells. Endothelial cell clusters (ECCs) comprise colonies of 2–50 cells. Low proliferative potential endothelial colony-forming cells (LPP-ECFC) contain more than 50 cells that form ECCs on replating. High proliferative potential endothelial colony forming cells (HPP-ECFC) are macroscopic colonies capable of forming secondary HPP-ECFC and LPP-ECFC. HPP-ECFCs maintain high telomerase activity. LPP-ECFCs and HPP-ECFCs typically contain cells that have a smaller size compared to EC or ECC
The ancestor cell of ECFCs is unknown. During development, endothelial cells originate from the hemangioblast, a common hematopoietic/endothelial stem cell (Samokhvalov et al. 2007; Nishikawa et al. 1998; Tavian et al. 2001; de Bruijn et al. 2002). Two detailed studies analyzed whether ECFCs are derived from this bi-potent stem cells during postnatal life. Isolation of circulating ECFCs from patients with clonal hematopoietic stem cell disorders showed that 3 of 89 ECFCs obtained from 11 patients carried a mutation identical to the hematopoietic stem cell, providing evidence for a common precursor for circulating ECFCs and hematopoietic stem cells for at least a minority of ECFCs in the peripheral blood (Yoder et al. 2007). On the other hand, in a larger cohort of 76 patients with clonal myeloproliferative disorders, all ECFCs were mutation negative (Piaggio et al. 2009). Study of circulating ECFCs in non-human primates over the lifespan from birth to elderly showed that clonogenic and proliferation capacity and in vivo vasculogenesis potential significantly decreased with age, suggesting that ECFC mobilization and function are adversely affected by aging (Shelley et al. 2012).
9.2 Mechanisms of Mobilization, Homing, and Angiogenic Function
ECFCs are mobilized and recruited to ischemic sites to contribute to neovasculogenesis and repair of injured tissues. However, little is known about the mechanisms via which ECFCs are released from the endothelium and become blood-borne. Whether mobilization of ECFCs occurs by passive shedding from the endothelium or an active migration out of the endothelium, and the molecular activators of this process have yet to be revealed.
Interestingly, in patients with acute myocardial infarction circulating ECFCs numbers were higher than cord blood, the richest healthy source of circulating ECFCs, suggesting that ischemia/injury governs the mobilization of these cells (Massa et al. 2009). Tracing of intracoronary-delivered lacZ-labeled ECFCs into acute myocardial infracted pigs showed specific incorporation of the cells into the ischemic/injured area and not in other organs, except for the splenic sinusoids (Dubois et al. 2010). C-X-C chemokine receptor type 4 (CXCR-4) expression on ECFCs played a critical role in ex vivo migration of the cells towards stromal cell-derived factor-1α (SDF-1α). Sorting of cord blood and human umbilical cord ECFCs based on the level of CXCR-4 expression showed that the cells displaying high numbers of receptors homed more efficiently to ischemic sites in mouse hindlimb injury model and exhibited increased vasculogenesis potential compared to ECFCs with low-CXCR4 expression (Oh et al. 2010). The expression of CXCR-4 on cord and adult blood ECFC is suppressed by thrombospondin-1, an endogenous angiogenesis inhibitor. Blocking of thrombospondin-1 in ECFCs resulted in increased migration in vitro and boosted the angiogenic potential of these cells in the hindlimb ischemia model (Smadja et al. 2011a).
More recently, synthetic peptide WKYMVm, a selective agonist of G-protein-coupled formyl peptide receptor-2, was identified as potent promoter of homing and incorporation of intravenously injected cord blood ECFCs into ischemic limb in a mouse hindleg ischemia model. Activation of the formyl peptide receptor-2 on the cell surface of ECFCs by WKYMVm augmented migration in scratch wound assays, cell proliferation, and in vitro tube formation in Matrigel assays. Intramuscular injection of WKYMVm at the site of injury increased ECFC homing and engraftment into newly formed blood vessels resulting in greater blood perfusion and limb salvage (Heo et al. 2014).
ECFC function is critically dependent on migration and luminalization. Vascular endothelial cell growth factor (VEGF)-induced migration and in vivo capillary formation by cord blood-derived ECFCs are critically regulated by urokinase-type plasminogen activator receptor (uPAR), which has an established role in extracellular matrix degradation and cell adhesion (Margheri et al. 2011). Lumen formation is a critical step in neovascularization. Angiopoietin-like protein 2 has recently been shown to control ECFC luminalization in three dimensional collagen assays by activation of c-Jun NH2-terminal kinase and up-regulation of membrane type I matrix metalloproteinase (Richardson et al. 2014). There is conclusive evidence that ECFCs are able to form de novo endothelium in newly formed blood vessels. ECFCs in collagen-1/fibronectin or Matrigel implanted subcutaneously into NODSCID mice promoted vascularization (Yoder et al. 2007; Melero-Martin et al. 2007). In addition to structural contribution to the nascent endothelium, ECFCs also stimulate new vessel formation in a paracrine fashion by activation of local ECFCs (Alphonse et al. 2014; Baker et al. 2013). The soluble factors driving this paracrine angiogenic activity remain to be determined.
9.3 ECFCs in Healthy Lungs and Pulmonary Arterial Hypertension
ECFCs with robust self-renewal and de novo vessel formation potential have been isolated from neonatal rat lungs and human fetal lungs at 17–22 weeks of gestation, indicating that these cells are critical in alveolar development (Alphonse et al. 2014). ECFC-like cells with high proliferation capacity, self-regeneration potential, and in vivo vasculogenesis activity were also found to be enriched in adult mouse or rat lung mircovascular endothelium as compared to the pulmonary artery (Alvarez et al. 2008; Schniedermann et al. 2010).
Pulmonary arterial hypertension (PAH) is characterized by severe angiogenic remodeling of the pulmonary circulation (Heath and Edwards 1958; Tuder et al. 2007). Emerging evidence suggests that ECFCs may play an active role in the angiogenic remodeling (Alphonse et al. 2014; Duong et al. 2011; Toshner et al. 2009). Both healthy and PAH pulmonary artery endothelial cells harbor similar numbers of ECFCs (Duong et al. 2011). However, ECFCs from PAH PAECs exhibited significantly higher cell numbers in culture and gave rise to more HPP-ECFCs (Duong et al. 2011) and increased vasculogenesis in vivo (Fig. 9.2). These findings demonstrate that ECFCs are enriched among adult PAECs. In PAH, ECFC frequency in the pulmonary endothelium is unaltered, but the expansion capacity of these ECFCs is amplified. In children with PAH, ECFCs have been isolated from the peripheral blood mononuclear cell fraction (Smadja et al. 2011b). Their numbers, cell proliferation, and vascular injury repair capacity in a nude mouse hindleg ischemia model increased after prostacyclin therapy, suggesting that this class of drug may provide clinical benefits to patients by enhancing ECFC function (Smadja et al. 2011b). Circulating ECFCs cultured from adult PAH patients had a higher proliferation rate compared to ECFCs obtained from healthy individuals and impaired angiogenic capacity in ex vivo Matrigel assays (Toshner et al. 2009). However, enthusiasm that peripheral blood circulation may be a useful source of pulmonary circulation-derived ECFCs was dampened by analysis of circulating ECFCs from a pair of monozygotic twins carrying bone morphogenetic protein type 2 receptor (BMPR2) mutation. One of the twins received a heart and double lung transplant for severe PAH. Five years after the transplantation of the afflicted twin, ECFCs from both twins were analyzed for host or donor origin by sequencing of the specific BMPR2 mutation. Circulating ECFCs from both twins after transplantation carried equal amounts of the mutated allele, demonstrating that they did not come from the donor lungs or heart. This case study suggested that ECFCs in the peripheral blood circulation may not be derived from a pulmonary or cardiac origin (Ormiston et al. 2013).
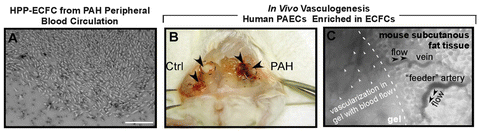
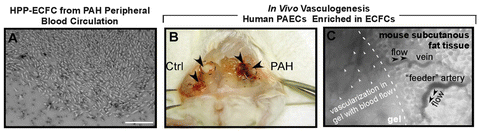
Fig. 9.2
ECFC in pulmonary arterial hypertension blood circulation and pulmonary artery endothelium. (a) HPP-ECFC obtained from PAH patient’s peripheral blood. Bar = 200 μm. (b) Increased de novo vasculogenesis by PAH PAECs enriched in HPP-ECFCs. Healthy control PAECs and PAH PAECs, enriched in ECFCs, were inoculated in collagen-I/fibronectin extracellular matrix gel. After overnight incubation at 37 °C in EGM-2 medium, the gels were surgically implanted into the abdominal wall, between the dermis and peritoneum of humanized NODSCID mice (Yoder et al. 2007). In contrast to the traditional matrigel assay, this technique allows the study of vasculogenic capacity of ECFCs without invasion of the gel by mouse cells. Animals were analyzed after 1 week. (c) Sidestream dark field (SDF) imaging (MicroScanTM, MicroVisionMedical, Wallingford, CT) was used to visualize vasculogenesis in the gel. This is a newly available technique in which tissue-penetrating green light (530 nm) is emitted by a probe positioned on top of the collagen-I gel in an anesthetized mouse. The light is absorbed by the hemoglobin in the red blood cells. Stroboscopic illumination and capturing of the reflected light allows the observation of flowing cells. A captioned still image of this video is shown. Dark network (white arrows) indicates newly formed blood vessels in the gel connected to the mouse circulation
Animal models support that ECFCs may play a role in PAH. Studies of chronic hypobaric hypoxic calves revealed increased frequency of HPP- and LPP-ECFCs in the adventitial vasa vasorum of the thickened pulmonary artery wall compared to normoxic calves. Of note, vasa vasorum ECFCs’ number in hypoxic animals was considerably higher compared to the frequency among aortic and main pulmonary artery endothelial cells. Furthermore, in Matrigel tube formation assays, vasa vasorum ECFCs from hypoxic calves exhibited increased angiogenesis compared to control ECFCs (Nijmeh et al. 2014).
The greater presence of highly proliferation angiogenic ECFCs among pulmonary artery endothelial cells and pulmonary artery vasa vasorum endothelial cells suggests that these cells may play an active role in the pathological angiogenesis of PAH (Fig. 9.3).
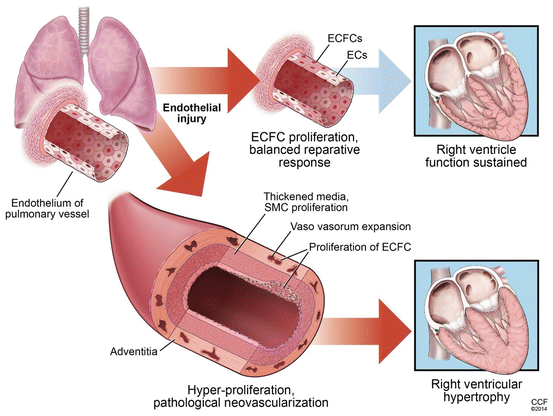
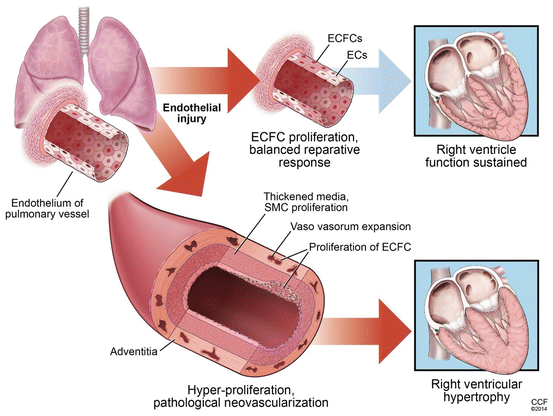
Fig. 9.3
ECFCs in pulmonary arterial hypertension. Our knowledge of ECFC biology in lung health or disease is at a primordial stage. Likewise, very little is known about the exact function of ECFCs in pulmonary arterial hypertension. A following working model could be proposed based on the current literature. ECFCs play both beneficial and pathological roles in pulmonary arterial hypertension. After an initial insult to the pulmonary artery endothelium, ECFCs are activated to repair the injury. Both, resident and circulating, ECFCs may participate in this process. In pulmonary arterial hypertension with intrinsic impaired ECFC function, prostacyclin therapy or administration soluble factors derived from healthy ECFCs (such as cord blood) could be used to restore ECFC activity to promote a reparative response. In situations with repetitive injury or uncontrolled propagation of the reparative response, proliferation of ECFCs in the pulmonary artery is increased contributing to pathological remodeling by expansion of vasa vasorum in the pulmonary adventitia wall and formation of angioproliferative lesions in the pulmonary endothelium
9.4 Therapeutic Potential of ECFCs in Cardiovascular Diseases
Several lines of evidence substantiate that ECFCs may hold promise for cell therapy. Infusion of autologous ECFCs in pigs, 1 week after the induction of experimental acute myocardial infarction, resulted in attenuated pathological remodeling and increased vascularization of the infracted zones with neoendothelium formation by the ECFCs and improvement of left ventricle function (Dubois et al. 2010). In a rabbit model of wire injury-induced intima hyperplasia, transfusion of autologous ECFCs inhibited neointima formation by facilitating restoration of the damaged endothelium (Liu et al. 2011). ECFCs isolated from hyperoxia-exposed neonatal rats or human fetal lungs subjected to hyperoxia ex vivo showed impaired proliferation, self-renewal, and reduced in vitro tube formation on Matrigel, all of which were restored after intra-jugular administration of cord blood ECFCs in the hyperoxic neonatal rats (Alphonse et al. 2014). Despite this therapeutic effect, a poor engraftment of the cord blood ECFCs in neonatal rats was observed and similar beneficial restoration of alveolar growth was obtained by infusion of ECFC-conditioned medium, indicating a potential paracrine mechanism (Alphonse et al. 2014). Interestingly, bronchopulmonary dysplasia-induced pulmonary hypertension in the hyperoxic newborn rats, including right ventricular hypertrophy and pulmonary artery acceleration time, a sonographic surrogate measure of pulmonary artery pressure, was attenuated by ECFC therapy (Alphonse et al. 2014). Similar restorative benefits were observed with cord blood ECFCs and cord blood ECFC-conditioned medium in bleomycin-exposed newborn rats, another experimental model for bronchopulmonary dysplasia (Baker et al. 2013). In this study, cord blood ECFC-conditioned medium inhibited right ventricular hypertrophy, but did not contribute to alveolarization
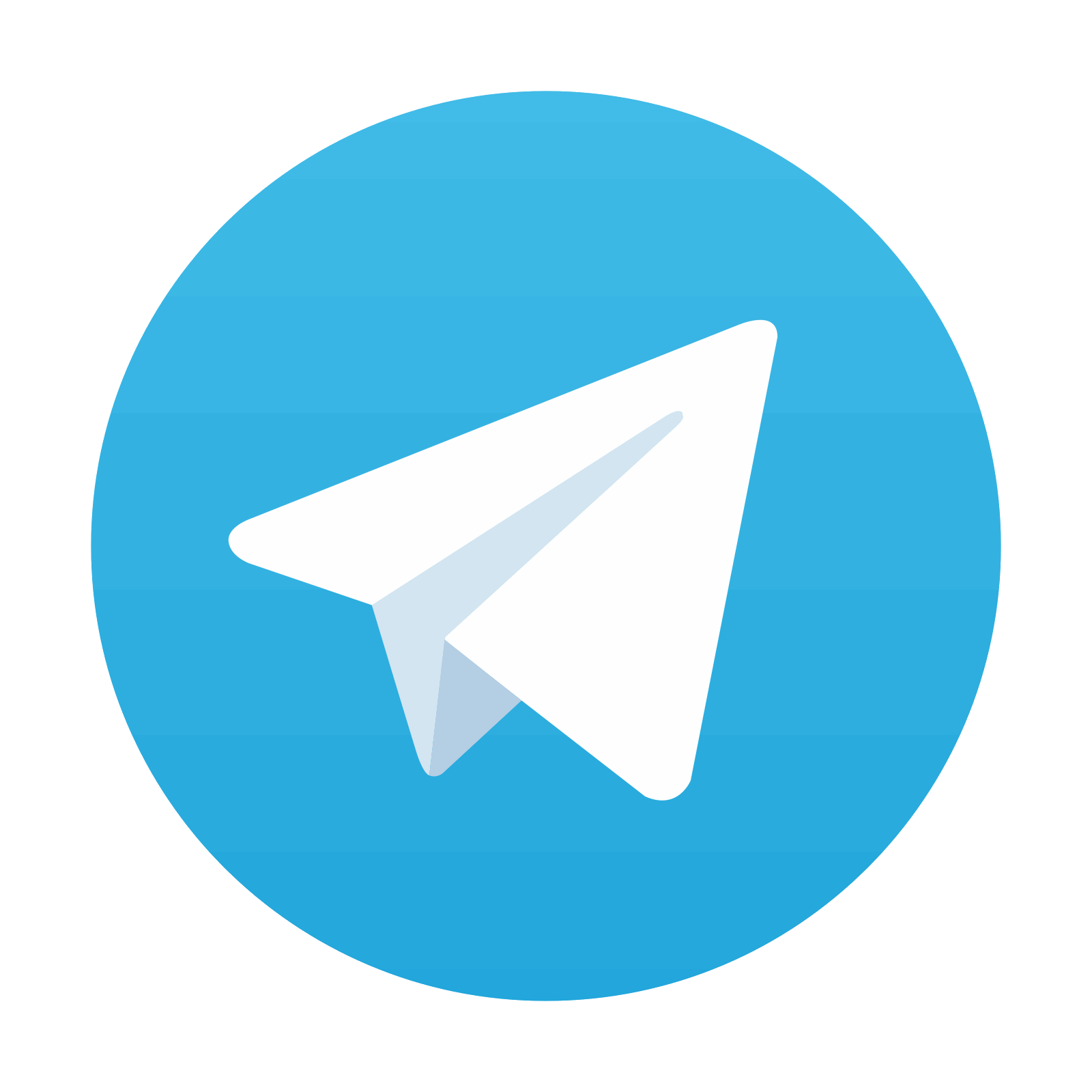
Stay updated, free articles. Join our Telegram channel

Full access? Get Clinical Tree
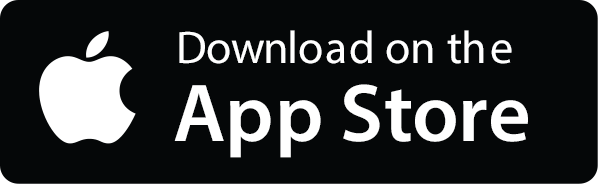
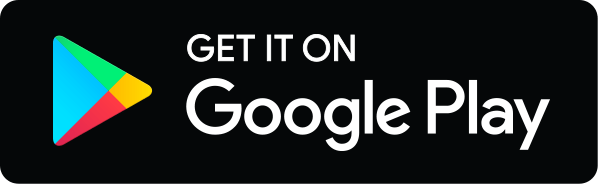