identifiable pituitary mass on CT or MRI, pituitary hormones are stable following CPB (16).
![]() FIGURE 11.2. Plasma concentration of arginine vasopressin (AVP) during nonpulsatile bypass for mitral valve replacement (MVR, n = 8), aortic valve replacement (AVR, n = 5), or coronary artery bypass grafting (CABG, n = 5). Data are presented as means ± SEM. As indicated, measurements were obtained at (1) anesthesia induction, (2) sternotomy, (3) 10 minutes after initiation of cardiopulmonary bypass, (4) 10 minutes before termination of cardiopulmonary bypass, (5) upon arrival in the critical care unit, (6) 6 hours after bypass, (7) 18 hours after bypass, (8) 30 hours after bypass, and (9) 48 hours after bypass. All three groups of patients demonstrated significant increases in AVP concentrations during bypass. Only at sample 5 did the mitral valve patients demonstrate significantly greater AVP concentration than the CABG patients. p values on the figure indicate comparisons between sample 1 and subsequent samples in the same surgical group. (From Kaul TK, Swaminathan R, Chatrath RR, et al. Vasoactive pressure hormones during and after cardiopulmonary bypass. Int J Artif Organs 1990;13:293-299, with permission.) |
syndrome is related to inappropriately reduced ADH concentrations in those patients that had increased concentrations preoperatively, which may reduce the ability to increase ADH as part of the stress associated with cardiac surgery (32). A reduced EF and/or chronic treatment with ACE inhibitors were independently associated with vasoplegia and with this relative postoperative ADH deficiency (33). This finding is consistent with studies in sepsis associating inappropriately diminished ADH levels in the presence of chronic stimuli to ADH secretion (34).
TABLE 11.1. Blood pressure and plasma catecholamine concentrations during extracorporeal perfusion in patients undergoing aortocoronary bypass grafting | ||||||||||||||||||||||||||||||||||||||||||||||||||||||||
---|---|---|---|---|---|---|---|---|---|---|---|---|---|---|---|---|---|---|---|---|---|---|---|---|---|---|---|---|---|---|---|---|---|---|---|---|---|---|---|---|---|---|---|---|---|---|---|---|---|---|---|---|---|---|---|---|
|
epinephrine occurred when the heart and lungs were excluded from the circulation (38,39,40). However, norepinephrine and epinephrine concentrations peaked at different times. In a later study, patients undergoing cardiac surgery were randomly assigned to have CPB with mild (34°C) or moderate (28°C) hypothermia. With both bypass temperatures, peak norepinephrine concentrations were observed after release of the aortic crossclamp and rewarming, whereas peak epinephrine concentrations were observed at the target hypothermic temperature (42). A more recent study demonstrated a biphasic plasma norepinephrine concentration response to nonpulsatile CPB, with concentrations peaking at aortic declamping and again 2 to 4 hours after surgery. Epinephrine concentrations did not show this pattern, nor was it observed during pulsatile CPB (40,41). Neonates, infants, and young children, much like adults, demonstrate marked increases in catecholamine concentrations during CPB (2,41,43,44,45) (Fig 11.4).
to baseline 24 hours postoperatively. It is therefore not surprising that even “off-pump” coronary revascularization procedures are associated with increases in serum cortisol and other markers of the stress response despite the absence of the stress of CPB (54). It is apparent, however, that CPB modifies cortisol responses to surgery. Total plasma cortisol concentrations typically briefly decrease immediately upon initiation of bypass, likely as a consequence of hemodilution (55,56,57,58) (Fig. 11.7). During bypass, cortisol concentrations rise to values significantly above baseline (2,5,55,56,57,58,59,60). After CPB, patients exhibit markedly elevated concentrations of cortisol (both free and total) for more than 48 hours (58,59,60,61). In a recent study, cortisol increased 5-fold from baseline 2 hours after “on pump” coronary artery surgery versus a maximal 3-fold increase after “off pump” coronary artery surgery (60). Interestingly, the maximal increase occurred significantly earlier in the “off pump” group (4 hours after surgery) than in the “on pump” group (12 hours after surgery). The authors speculated that this finding resulted from differences in inflammatory processes between the two forms of coronary artery surgery. Cortisol levels slowly decreased thereafter in both groups.
![]() FIGURE 11.5. Effects of thoracic epidural anesthesia with bupivacaine 0.5% (▪, n = 8) versus control (♦, n = 9) on catecholamine concentrations measured during coronary artery surgery. All 17 patients studied received general anesthesia with sufentanil 20 µg/kg. Samples were obtained (1) before anesthesia, (2) after anesthesia induction, (3) after 30 minutes of surgery, (4) after 30 minutes of cardiopulmonary bypass (CPB), (5) after 60 minutes of CPB, (6) 1 hour after CPB, (7) 2 hours after CPB, (8) 4 hours after CPB, (9) 6 hours after CPB, and (10) 24 hours after CPB. *p < 0.05, **p < 0.01 for between-group differences. Adrenaline, epinephrine; NA, noradrenaline or norepinephrine. (From Lee TW, Grocott HP, Schwinn D, et al. High spinal anesthesia for cardiac surgery: effects on beta-adrenergic receptor function, stress response, and hemodynamics. Anesthesiology 2003;98:499-510, with permission.) |
concentration during CPB can be blunted by perfusion with blood at 20°C compared to 28°C. Peak CPB cortisol concentrations were decreased by deeper planes of anesthesia in both adults and children (2,58,59) (Fig. 11.8). Winterhalter et al. (48) showed that when continuous remifentanil (0.25 µg/kg/min) was compared to intermittent bolus dose fentanyl (2.6 ± 0.3 mg/kg total dose) corticotropin (adrenocorticotropic hormone, ACTH), cortisol, and vasopressin were all significantly decreased. This result could be secondary to the specific agent (remifentanil vs. fentanyl), variations in the depth of anesthesia, or the steady state produced by continuous anesthetic infusions. Stenseth et al. (49) found that, compared with high-dose fentanyl anesthesia alone, high-dose fentanyl anesthesia plus thoracic epidural anesthesia delayed the increase in cortisol concentrations during coronary artery surgery and reduced concentrations during bypass. Similarly, Moore et al. (50) found that thoracic epidural anesthesia combined with sufentanil 20 µg/kg was associated with markedly lower cortisol concentrations as compared to sufentanil anesthesia alone. On the other hand, spinal anesthesia failed to attenuate cortisol responses (compared to intravenous general anesthesia) in children undergoing correction of congenital heart defects (3).
already maximal. Amado and Diago (67) observed a blunted response to corticotropin-releasing hormone during bypass, similar to responses seen in patients with hypothalamic corticotropin-releasing hormone deficiency. In contrast, an earlier study revealed that when patients undergoing extracorporeal perfusion received ACTH, cortisol concentrations increased (55).
is the only organ exposed to the entire cardiac output (except during CPB). Early investigations have studied small numbers of cardiac surgery patients randomized to variable doses of different corticosteroids (most commonly 1 mg/kg dexamethasone or 30 mg/kg methylprednisolone) initiated at varying intervals between induction of anesthesia and the start of CPB (72). Overall, study results generally demonstrate an amelioration of the inflammatory response, with decreases in cytokine formation (tumor necrosis factor and the interleukin [IL]-1, -6, and -8) but inconsistent effects on C3a and elastase concentrations. Leukotrienes such as LTB4 are decreased in a dose-dependent fashion (72,73). IL-10, a cytokine with actions that are principally anti-inflammatory, demonstrates increased concentrations with steroid administration, supporting an anti-inflammatory effect (73). In addition, large doses of methylprednisolone can block upregulation of neutrophil integrin adhesion receptors, whereas dexamethasone decreases endothelial production of certain adhesion molecules (74). Clinically, glucocorticoid therapy may increase cardiac index and decrease systemic vascular resistance (75). Dietzman et al. (76) showed improvement in tissue perfusion and a decrease in peripheral vascular resistance when a large glucocorticoid dose was given just before CPB. Routine glucocorticoid supplementation has also been advocated as part of an accelerated recovery program (77), albeit without much supporting evidence. A small study has shown that cardiac surgery patients receiving glucocorticoids had shorter lengths of stay and improved quality of life as compared to patients not receiving glucocorticoids (78). The same research group found that steroid treatment reduced the duration of catecholamine support, length of intensive care unit (ICU) stay, and likelihood of postoperative atrial fibrillation (79). A 2013 meta-analysis that included 48 randomized controlled trials (RCTs) of patients receiving corticosteroids undergoing CPB failed to show any difference in the incidence of myocardial infarction, stroke, renal insufficiency, or death. The only outcome effect identified was a modest and heterogeneous decreased ICU and hospital length of stay in the groups receiving steroids (73).
TABLE 11.2. Effect of nonpulsatile (n = 18) or pulsatile (n = 20) cardiopulmonary bypass on glucose metabolism | ||||||||||||||||||||||||||||||||||||||||||||||||||||||||||||||||||||||||||||||||
---|---|---|---|---|---|---|---|---|---|---|---|---|---|---|---|---|---|---|---|---|---|---|---|---|---|---|---|---|---|---|---|---|---|---|---|---|---|---|---|---|---|---|---|---|---|---|---|---|---|---|---|---|---|---|---|---|---|---|---|---|---|---|---|---|---|---|---|---|---|---|---|---|---|---|---|---|---|---|---|---|
|
peptide (ANP, A-type natriuretic peptide), brain natriuretic peptide (BNP, B-type natriuretic peptide), and C-type natriuretic peptide (CNP), among others (100). ANP and BNP are expressed in cardiac myocytes. The designation of BNP as “brain” natriuretic peptide derives from its initial discovery in porcine brain, but the role of BNP and ANP in the central nervous system remains unclear. ANP is produced and stored predominantly in the atrium. BNP is present in both atrial and ventricular tissue, but in myocardial disease states the ventricle becomes the primary source of BNP secretion. The nervous system and endothelial cells are the major sites of expression of CNP, which has significant paracrine vasodilatory effects. The heart is not a significant source of CNP. ANP is released in response to atrial distention, whereas BNP levels tend to be elevated in the presence of ventricular dysfunction. Other stimuli, some of which are commonly associated with CPB, may promote ANP and/or BNP release, including myocardial ischemia (101), catecholamines, endothelin-1, prostacyclin, and cytokines (102). Both peptides increase glomerular filtration, inhibit renin release, reduce aldosterone concentrations in blood, antagonize renal vasoconstrictors (such as vasopressin, norepinephrine, and angiotensin), and reduce arterial blood pressure. They regulate vascular volume by increasing sodium excretion and decreasing vasomotor tone (103). Within the heart natriuretic peptides regulate myocyte growth and inhibit fibroblast proliferation and extracellular matrix deposition. Natriuretic peptides have an anti-ischemic (preconditioning-like) function, and influence coronary endothelial and vascular smooth muscle proliferation and contractility through activation of guanylate cyclase (104,105).
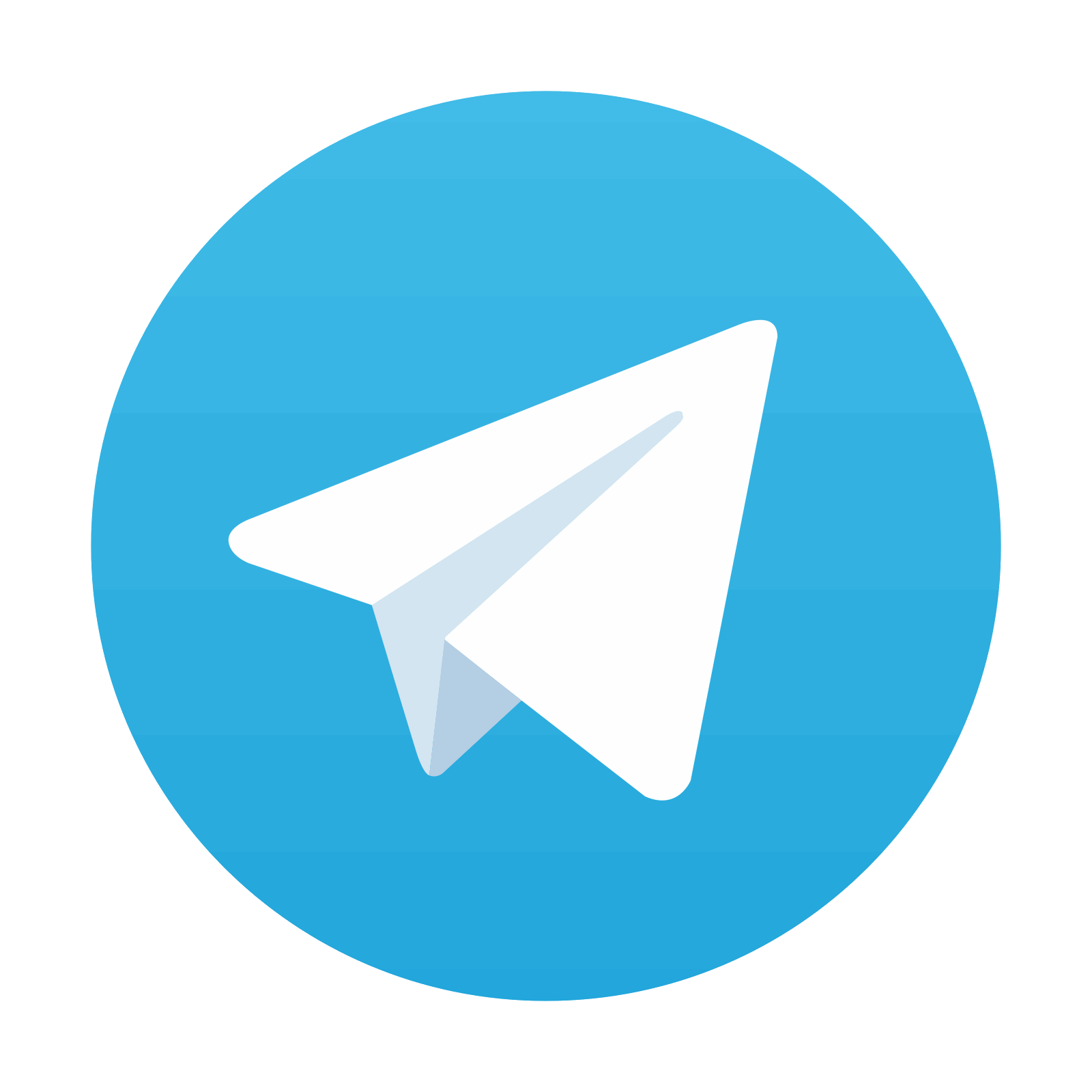
Stay updated, free articles. Join our Telegram channel

Full access? Get Clinical Tree
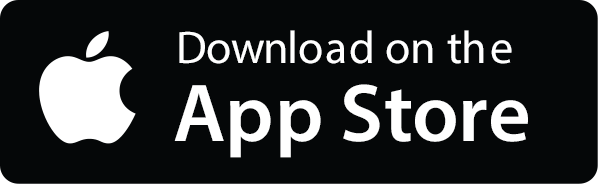
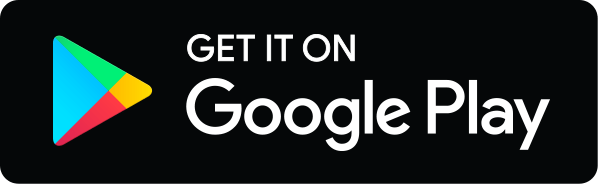