Abstract
The endocrine system plays an important role in generation of the stress response that occurs after cardiac surgery in pediatric patients. Surgery with cardiopulmonary bypass has been associated with hormonal perturbations and release of inflammatory cytokines and metabolic mediators. Particular attention has been directed toward glycemic control and alterations in thyroid and adrenal function. Hyperglycemia is very common after pediatric cardiac surgery and has been repeatedly associated with adverse outcomes, but efforts to maintain tight glycemic control have resulted in conflicting results. Similarly, low levels of thyroid hormones have been associated with surgery with cardiopulmonary bypass, but replacement therapy, although successful in establishing normal levels, has produced beneficial results only in selected populations. Suppression of adrenal function has also been recognized to ensue after cardiac surgery, and several studies have investigated the results of pharmacologic steroid replacement therapy. The results are variable and far from conclusive. Finally, calcium homeostasis has been increasingly recognized as an important element of myocardial contractility, and calcium infusion therapy has been suggested as potentially beneficial therapy in cases of hypotension associated with myocardial dysfunction. Again, data are insufficient for evidence-based recommendations.
Key Words
pediatric cardiac surgery, cardiopulmonary bypass, glycemic control, thyroid replacement therapy, adrenal insufficiency, low cardiac output syndrome
The role of the endocrine system in regulation of the stress response to cardiac surgery and postoperative recovery has been increasingly recognized over recent years. Evidence associating the magnitude of hormonal stress response to postoperative morbidity have led to efforts to suppress this response. As we will discuss later in this chapter, the results of this strategy have been conflicting. It has become increasingly appreciated that some of the hormonal alterations following cardiac surgery are adaptive and necessary. However, maladaptive responses that are likely to lead to unfavorable outcomes also exist and have been linked to the release of proinflammatory cytokines and activation of chemokine responses that in turn propagate endocrine and metabolic alterations. Changes in plasma levels of catecholamine, thyroid-stimulating hormone (TSH), thyroid hormones, cortisol, vasopressin, insulin, growth hormone, and the renin-angiotensin-aldosterone axis have been documented as part of the hypermetabolic stress response to cardiac surgery and critical illness.
The most prominent hormonal response to cardiopulmonary bypass (CPB) in children, especially neonates, is the tremendous increase of endogenous catecholamines. Surgical stress, pain, acidosis, lack of pulsatile flow, and relative ischemia have all been implicated as potential causes. Elevation of plasma epinephrine levels has been described in neonates after sternotomy and before initiation of CPB, after deep hypothermic circulatory arrest, at the end of the operation and 6, 12, and 24 hours postoperatively and has been associated with decreased survival. Norepinephrine levels, in some studies, appear to increase intraoperatively and normalize more quickly. Both contribute to postoperative vasoconstriction and impaired cardiac function. Given that sympathetic activation plays a key role in mediation of the stress response, opioid use to achieve deep level of anesthesia and postoperative analgesia has been shown to attenuate the catecholamine surge and potentially decrease postoperative complications.
Alterations in levels of TSH, thyroid hormones, and cortisol will be discussed later in detail. Briefly, children after cardiac surgery develop a nonthyroidal illness (sick euthyroid) syndrome (NTIS) with low thyroid hormone levels and inappropriately low or normal TSH levels. Cortisol levels, if not confounded by the use of perioperative steroids, are elevated in most patients as a result of hypothalamic-pituitary-adrenal (HPA) axis activation and increased responsiveness of the adrenal cortex. However, a significant minority of children after cardiac surgery have low baseline cortisol levels and an inadequate response relative to severity of illness.
The release of vasopressin is also part of the stress response. Significant elevations of plasma vasopressin levels have been documented in children after CPB. However, recent reports have indicated that a small subgroup of patients have absolute or relative (for the severity of illness) vasopressin deficiency and if hypotensive, exhibit a favorable hemodynamic response to low-dose vasopressin (that will not cause vasoconstriction in healthy subjects).
Similar to adults undergoing cardiac operations, plasma insulin concentration in children usually decreases during the initial stages of CPB and hypothermia, partly related to impaired splanchnic perfusion. Insulin levels frequently then increase at the end of the surgery and remain high for the first 24 postoperative hours. However, this is associated with a decreased peripheral response to insulin and elevation of glucagon and growth hormone levels, with the net effect of transient postoperative hyperglycemia in the majority of cases. Glucagon secretion is also proportional to the magnitude of insult, being markedly elevated after complex surgery. Indeed, it is very likely that the observed postoperative elevation in insulin levels is secondary to perioperative hyperglycemia rather than a primary stress response. Notably, the insulin/glucagon molar ratio is decreased after cardiac surgery in children and can be viewed as the primary driver to postoperative hyperglycemia.
Although many pediatric cardiac patients have elevated plasma aldosterone levels preoperatively related to fluid status and heart failure, aldosterone levels have been found to decrease below preoperative levels during the first 24 postoperative hours. Conversely, brain natriuretic peptide (BNP) level commonly peaks 6 to 12 hours postoperatively, but the timing and magnitude of its elevation demonstrates age dependency. Neonates exhibit earlier peak and higher levels than older children. Perioperative BNP levels have been associated with morbidity and postoperative outcomes. Higher BNP levels have been associated with longer ventilation time, duration of intensive care unit (ICU) stay, and higher inotropic needs.
Summarizing, major catabolic stress responses under neuroendocrine axis control have been found in children undergoing cardiac surgery. Neonates are particularly affected due to the enhanced metabolic response to surgical stress and the maturational variation in endocrine function occurring in the first month of life.
Glycemic Control After Pediatric Cardiac Surgery
Transient hyperglycemia in children with previously normal glucose homeostasis is ubiquitous after cardiac surgery. The prevalence of blood glucose levels above 126 mg/dL is reported as high as 90% in some series. Hyperglycemia in an individual patient resolves over time, and the majority of the hyperglycemic patients have normalized blood glucose levels within 24 to 48 hours after cardiac surgery.
The etiology of critical illness–associated hyperglycemia is multifactorial. It involves both increased hepatic production of glucose (glycogenolysis and gluconeogenesis) and decreased peripheral utilization and so is part of the response to the severe physiologic stress of surgery and illness. This response involves activation of the HPA axis, release of stress hormones such as cortisol, epinephrine, norepinephrine, glucagon, and adrenocorticotropic hormone (ACTH) and generation of proinflammatory cytokines (interleukin-6 [IL-6], IL-8, tumor necrosis factor-α) that are implicated in the development of peripheral resistance to insulin. Furthermore, as indicated by low endogenous C-peptide production, primary beta-cell dysfunction with relative insulin deficiency relative to the degree of hyperglycemia has been documented in children with cardiorespiratory failure and can contribute to the development of hyperglycemia after cardiac surgery.
Several retrospective studies have associated postoperative hyperglycemia after cardiac surgery with adverse clinical outcomes such as higher mortality, lactic acidosis, cardiac arrest, vasopressor use, and longer ventilation and ICU stay. However, no evidence exists to date to associate hyperglycemia with adverse neurodevelopmental outcomes in children with cardiac disease.
In a large two-center retrospective study of 378 children undergoing complex heart surgery, average (≥143 mg/dL) and peak early postoperative glucose levels (≥250 mg/dL) were independently associated with a composite outcome of morbidity and mortality. In the same study the duration of hyperglycemia in the first 72 hours was associated with prolonged hospital stay. Similarly, low average glucose level (<109 mg/dL) and lower minimum glucose levels were also associated with higher odds of morbidity. The authors concluded that both hyperglycemia and hypoglycemia are associated with adverse outcomes, and the optimal blood glucose level in children after heart surgery might be between 110 and 126 mg/dL. Nevertheless, it appears that hyperglycemia and the odds of adverse outcome have a dose-dependent relationship. Increased duration and severity of hyperglycemia renders more likely an association with inadvertent outcomes.
It is unclear whether hyperglycemia is a marker or a cause of adverse outcomes. In critically ill adult surgical patients, an initial single-center randomized controlled trial showed that tight glycemic control reduced morbidity and mortality, but subsequent trials have not shown benefit. In children following cardiac surgery, three randomized clinical trials so far have attempted to investigate these associations and evaluate the effect of targeted glucose management. First, in a single-center trial of 700 critically ill children (75% after cardiac surgery), Vlasselaers et al. showed that tight glycemic control (blood glucose level 50 to 80 mg/dL) led to reduction in inflammation and length of ICU stay. Although the study was not powered to determine differences in mortality, the tight control group had fewer deaths. The second trial to discuss is the Safe Pediatric Euglycemia After Cardiac Surgery (SPECS) trial, which enrolled 980 children up to 3 years of age after cardiac surgery. No differences existed between the tight glycemic (blood glucose 80 to 100 mg/dL) group and standard care group in the primary outcome (health care–associated infections) or secondary outcomes such as mortality, length of stay, or measures of organ failure. However, a secondary analysis of this trial indicated that tight glycemic control may lower the risk of infection in children older than 2 months at the time of cardiac surgery. Finally, the Control of Hyperglycaemia in Paediatric Intensive Care (CHiP) trial, randomized 1369 critically ill children (60% after cardiac surgery) to tight (blood glucose level 72 to 126 mg/dL) or conventional glycemic control (blood glucose level <216 mg/dL). No difference in mortality or other major clinical outcomes were found. In the noncardiac cohort, tight glycemic control led to decreased length and reduced cost of hospital stay. This benefit was not apparent in the children after cardiac surgery. Although it excluded patients after cardiac surgery, recently the Heart and Lung Failure—Pediatric Insulin Titration (HALF-PINT) trial that randomized children with cardiovascular or respiratory failure and confirmed hyperglycemia to a lower target (80 to 110 mg/dL) versus a higher target (150 to 180 mg/dL) of glycemic control was interrupted early due to lack of benefit and possibility of harm, given the higher incidence of catheter-associated infections and severe hypoglycemia in the lower target group.
When used, the treatment of hyperglycemia in critically ill children is with regular insulin. Insulin acts on liver receptors to inhibit hepatic glucose production and synthesis of fatty acids and on peripheral tissues (muscle and adipose tissue) to stimulate the uptake and metabolism of glucose. It also has an anabolic effect through increased synthesis and decreased breakdown of protein and decreased lipolysis.
Insulin treatment in the pediatric cardiac ICU (CICU) setting is usually in the form of a continuous intravenous infusion at a dose of 0.01 to 0.1 units/kg/h. Subcutaneous intermittent injections are used less frequently due to variable bioavailability depending on preparation used and perfusion of injection site. Regular IV insulin has a half-life of 5 to 15 minutes and will reach a steady state by approximately 45 to 60 minutes. Insulin metabolism takes place mainly in the liver (50%) but also in the kidneys (30%) and peripheral tissues (20%).
The major adverse effect of insulin treatment is hypoglycemia with its associated concerns regarding deleterious effects to brain and neurodevelopment. The study by Vlasselaers et al. had a very high incidence of hypoglycemia (25%) but did not demonstrate a difference in neurodevelopment between the two groups. However, children were followed up at an older age (4 years) with no information regarding early neurodevelopment. In the CHiP trial, severe hypoglycemia occurred in only 1.5% of the conventional treatment group, but its incidence increased to 7.3% in the tight control group. Furthermore, in the subgroup of patients after cardiac surgery, patients who had at least one hypoglycemic episode had a higher rate of mortality compared to those with no hypoglycemia. This is consistent with data from critically ill adults that associate hypoglycemia during intensive glycemic control with mortality. In the SPECS trial the incidence of severe hypoglycemia was 3% in the glycemic control group compared to 1% in the standard care group. This study showed that safe insulin therapy can be achieved with a novel continuous glucose monitoring system and an explicit insulin infusion algorithm. Follow-up of this study indicated that, although tight glycemic control did not offer any measurable neurodevelopmental benefit at 1 year of age, moderate and severe hypoglycemia were associated with worse functioning in the cognitive, language, and motor domains.
Summarizing, the current evidence does not support tight glycemic control in children after cardiac surgery. Given reported benefits in critically ill children without cardiac disease and apparent positive effects in some cardiac studies, it is plausible that tight glycemic control might be beneficial in a subgroup of children after cardiac surgery. Identification of this speculative cohort is currently elusive. Emerging evidence on metabolic, inflammatory, and biomarker profiling that can be used to identify cases in which the perioperative inflammatory and metabolic responses are suggestive of adverse clinical outcomes could be potentially useful to this direction. However, at present, a strategy of tight glycemic control cannot be recommended in children after cardiac surgery.
Thyroid Disease and Thyroid Hormone Therapy After Cardiac Surgery
Thyroid hormone production is regulated by a loop mechanism involving the hypothalamus, hypophysis, and thyroid gland. Hypothalamic thyrotropin-releasing hormone (TRH) stimulates secretion of TSH by the anterior hypophysis, which in turn promotes synthesis of thyroxine (T 4 ) and triiodothyronine (T 3 ) by the thyroid gland through iodination of the tyrosine residues into thyroglobulin. Circulating thyroid hormones (plasma free and bound to thyroid-binding globulin, transthyretin, and albumin) exert negative feedback on the release of TSH and TRH. T 3 is secreted directly by the thyroid gland (20%) and also produced in the liver and peripheral tissue (80%) by deiodination of T 4 , which can be considered a prohormone for T 3 .
Thyroid hormones bind to receptor proteins in the cell nucleus and have a number of physiologic effects, including brain development, bone maturation through influence on calcium and phosphorus metabolism, and increase of basal metabolic rate. T 3 promotes protein synthesis and affects carbohydrate, lipid, and vitamin metabolism. On the cardiovascular system, thyroid hormones increase contractility, chronotropy, and oxygen consumption and decrease systemic vascular resistance. Thyroid hormones also influence calcium transport pathways through the cell membrane and sarcoplasmic reticulum in cardiac myocytes. T 3 is also a nuclear transcription factor and contributes to upregulation and downregulation of genes important for cardiac contractility. The consequent end-organ effects depend on circulating levels of active T 3 and thyroid receptor occupancy.
Children with congenital hypothyroidism have been found to have up to 10-fold higher incidence of congenital heart disease (5.5% to 8%) compared to the general population. Therefore newborns with elevated TSH level on screening should be examined carefully for cardiac malformations in addition to confirmatory thyroid function testing. In the CICU environment, exposure to iodine, such as during cardiac angiography with iodinated contrast or iodine-containing antiseptics, has been implicated in developing hypothyroidism (Wolff-Chiakoff effect). This is characterized by high TSH level and low/normal T 4 level, and it is distinct to the sick euthyroid syndrome induced by CPB, although it may play a contributory role in postoperative thyroid hormone changes. However, other studies have indicated that use of povidone-iodine irrigation in cases of delayed sternal closure is not associated with abnormalities in thyroid function. Data are insufficient at present to advise on the need for thyroid function testing after cardiac angiography or prolonged exposure to iodine-containing antiseptics.
CPB induces thyroid hormone level alterations that exhibit features similar to sick euthyroid syndrome (or NTIS) seen during critical illness: an initial surge of free thyroid hormones is followed by marked and persistent decrease of circulating T 3 (total and unbound) with an inappropriately normal or low TSH level. This is due to impaired deiodination from T 4 to T 3 in the peripheral tissues, in addition to blood dilution and central inhibition of the HPA axis induced by nonpulsatile flow. An increase in biologically inactive reverse T 3 is also noted. Inflammatory cytokine levels such as IL-6, macrophage inhibitory factor, and IL-8 have been linked to the development of these abnormalities. In severe cases T 4 level is also decreased. Low thyroid-binding globulin levels can further influence the metabolism of T 4 . The nadir is seen at 24 to 48 hours, and recovery starts with a rise in TSH level within 1 to 2 weeks, although very sick patients can exhibit slower recovery. Suboptimal nutrition can delay the recovery of thyroid function. The NTIS syndrome changes have been correlated with increased severity of illness. Total and free T 3 levels and, even more, T 3 uptake have been suggested as the best predictors of postoperative clinical course.
Given the association of thyroid hormone deficiencies with unfavorable clinical outcomes, exogenous thyroid hormone administration to correct postoperative deficiencies and restore thyroid hormone homeostasis has been proposed. This strategy has been shown to offer a dose-dependent benefit in cardiac output and improved outcomes in adults undergoing open heart surgery. In children, five randomized controlled trials have attempted to date to investigate the effect of thyroid hormone therapy after cardiac surgery.
First, Portman et al. evaluated a small group of 14 infants after tetralogy of Fallot or ventricular septal defect repair. The treatment group received a bolus of T 3 (0.4 mg/kg) before CPB and at myocardial reperfusion. The treatment group, although found to have similar low T 4 levels to the control group, maintained free and total T 3 levels at prebypass values for 24 hours and higher than the control group for 72 hours. However, there was no difference in inotropic requirements between the groups. Heart rate was transiently elevated and peak pressure-rate product increased after 6 hours in the treatment group. This finding correlated with the peak T 3 levels in the treatment group. The authors concluded that T 3 therapy corrects T 3 deficiencies and implied that it improves myocardial oxygen consumption and possibly enhances cardiac function reserve in infants after CPB.
In a randomized, double-blind, placebo-controlled trial, Bettendorf et al. evaluated 40 children after cardiac surgery with CPB. The treatment group was given a daily infusion of T 3 (2 mg/kg on postoperative day 1 and 1 mg/kg on subsequent days) for 12 days. Both groups were found to have low postoperative plasma concentration of thyrotropin, T 4 , free T 4 and T 3 , and elevated levels of reverse T 3 . Treatment with T 3 raised T 3 plasma concentration without affecting postoperative recovery of thyroid function. Furthermore, patients in the treatment group demonstrated increased cardiac index (as assessed by Doppler echocardiography) and had a lower mean therapeutic intervention score than patients treated with placebo. The improvement in cardiac function was more pronounced in patients who had longer operations and duration of CPB. No adverse effects with respect to heart rhythm or rate were associated with thyroid repletion.
Subsequently, Chowdhury et al. evaluated postoperative serum total T 3 levels in 75 children after cardiac surgery. Patients in their cohort who had T 3 below a prespecified level (<40 ng/dL and <60 ng/dL in neonates) and were mechanically ventilated (n = 28) were randomized to either continuous T 3 infusion or placebo. The continuous T 3 infusion dose (0.05 to 0.15 mcg/kg/h) was titrated to maintain serum levels between 80 and 200 ng/dL. Overall, no differences were found between treatment and control group in inotropic score, mechanical ventilation, or hospital stay. However, in the small subgroup of nine neonates (five treated, four controls) the overall postoperative management requirements (as analyzed by Therapeutic Intervention Scoring System [TISS] score) and inotropic score were lower in the T 3 -treated group. Furthermore, in the T 3 -treated group of neonates, mixed venous oxygen saturation increased by 17% between 18 and 24 postoperative hours versus only 2% in the nontreated group, albeit this difference did not reach statistical significance. No adverse effects were noted with T 3 treatment on heart rate, blood pressure, or arrhythmias.
Mackie et al. randomized 42 neonates after aortic arch reconstruction (Norwood operation or biventricular repair of interrupted aortic arch and ventricular septal defect) to receive either a continuous infusion of T 3 (0.05 mcg/kg/h) or placebo for 72 hours. Primary outcome was a composite clinical outcome score (based on time to negative fluid balance, sternal closure, and extubation) and cardiac index at 48 hours. No differences were found in cardiac index, time to sternal closure, and extubation, but T 3 -treated neonates more rapidly achieved negative fluid balance, obtaining a favorable composite clinical outcome score compared to controls. No serious adverse effects were attributed to T 3 therapy.
Recently the results of Triiodothyronine Supplementation in Infants and Children Undergoing Cardiopulmonary Bypass (TRICC) trial were reported. This was a multicenter, randomized, double-blind, placebo-controlled trial that evaluated 193 children less than 2 years of age after cardiac surgery. There was no difference in the primary outcome (time to extubation) between treatment (T 3 0.4/kg bolus immediately before CPB and on the release of the aortic clamp followed by 0.2/kg bolus at 3, 6, and 9 hours after cross-clamp release) and placebo group. Overall, no difference in time to extubation and inotropic scores between T 3 -treated and placebo group was found. Again, treatment with T 3 was considered safe with no difference in adverse events between groups. In a prespecified secondary analysis, response to thyroid treatment was found to be age specific. In particular, patients less than 5 months of age demonstrated a significant shortening in the time to achieve extubation (primary outcome), whereas older patients had a small but statistically significant delay. Furthermore, the younger cohort showed reduced inotropic requirements and improvement in cardiac function as evaluated by echocardiography.
Thyroid replacement therapy in the CICU setting usually takes place in the form of intravenous T 3 therapy. Regimens that have been used include continuous infusion at 0.05 to 0.15 mcg/kg/h to maintain serum levels within 80 to 200 ng/dL or bolus therapy with 0.4 mg/kg at initiation and termination of CPB and followed by 0.2 mg/kg every 3 hours for another three doses (hour 3, 6, and 9 after completion of CPB). The half-life of intravenous T 3 is approximately 7 hours in children but considerably longer (up to 16 hours) in infants. Oral T 3 administered at a dose of 0.5 mcg/kg every 12 hours has been shown to prevent the decline in T 3 levels that typically occurs after pediatric cardiac surgery. The oral form is inexpensive and more widely available, but it remains unclear if normalization of T 3 levels affects clinical outcomes. Furthermore, concerns relating to absorption in cases of impaired splanchnic perfusion due to low cardiac output or use of vasoactive medications will need to be addressed in future studies.
Summarizing, children after cardiac surgery typically develop a nonthyroidal illness (sick euthyroid) syndrome, which can occasionally be clinically important. It is still unclear if this response is adaptive or maladaptive, contributing to severity of illness and organ dysfunction. Given the unclear benefit of the T 3 supplementation therapy in the aforementioned studies, one can argue that severe illness causes severe NTIS and not vice versa. Although T 3 treatment in this setting appears safe, its clinical efficacy has not been proven in the entire cohort of children after cardiac surgery. Subgroups such as neonates and young infants are more likely to be benefited, but the evidence is not yet conclusive. Therefore data are not currently sufficient to support the routine use of T 3 supplementation in children after cardiac surgery.
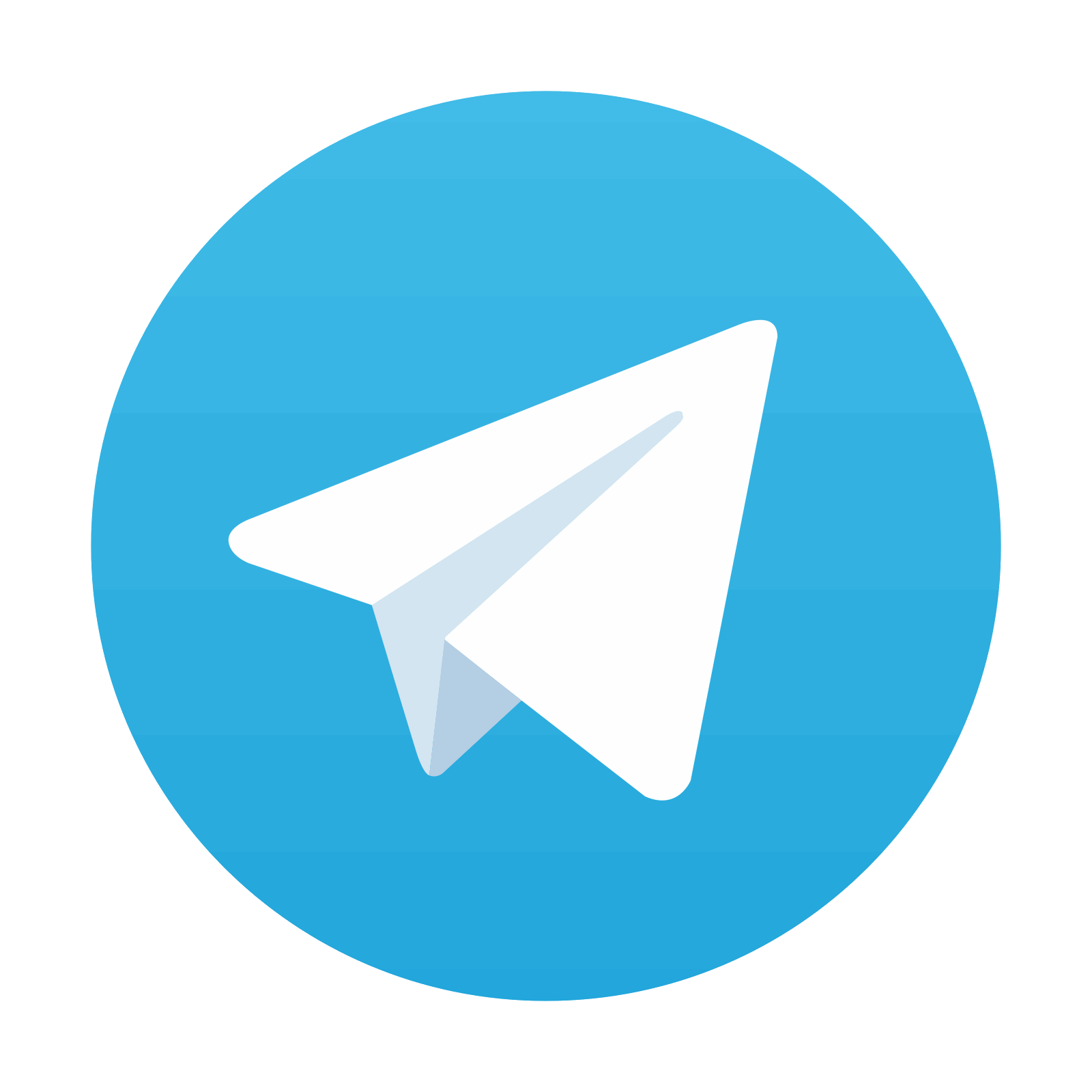
Stay updated, free articles. Join our Telegram channel

Full access? Get Clinical Tree
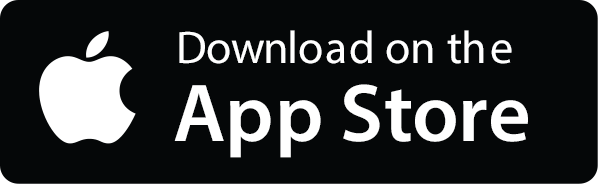
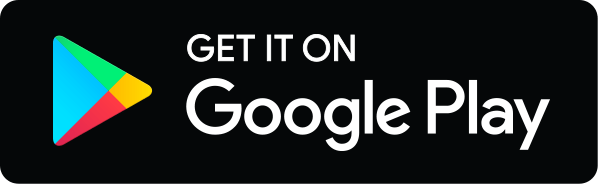