Chapter 36 Endocrine Disorders
Critical illness leads to widespread changes in the endocrine system; the extent of the changes depends on the severity and duration of the physiologic insult. These changes are similar in nature irrespective of whether the insult involves surgery, sepsis, trauma, or a major cardiovascular event. In this chapter the endocrine disorders that are commonly encountered in postoperative cardiac surgery patients are described and an approach to their management is provided. The stress response to surgery and cardiopulmonary bypass is discussed in Chapter 2.
HYPERGLYCEMIA
Hospitalized patients with hyperglycemia can be classified as follows1:
Hyperglycemia in nondiabetic patients is a risk factor for adverse outcomes. The stress of critical illness or major surgery increases circulating levels of the counterregulatory hormones glucagon, epinephrine, cortisol, and growth hormone. The net effect is hyperglycemia due to increased hepatic glucose production and decreased peripheral glucose uptake. A number of therapies commonly used in the intensive care unit (ICU) can also precipitate hyperglycemia. These include corticosteroids, β2-adrenoreceptor agonists (epinephrine, isoproterenol, and albuterol), excessive calories in parenteral or enteral nutrition, and dextrose-containing intravenous fluids.
Adverse Effects of Hyperglycemia and Rationale for Treatment
The adverse effects of hyperglycemia are shown in Table 36-1. Treatment with insulin reverses these effects and improves outcome in both diabetic and nondiabetic patients. In a study of diabetic patients with acute myocardial infarction, intensive control of hyperglycemia for at least 3 months, with a target blood glucose level of lower than 180 mg/dl (10 mmol/l), reduced mortality rates for at least 3 years.2 In studies of diabetic patients undergoing cardiac surgery, glucose control with a target of lower than 150 mg/dl (8.3 mmol/l) during the first 2 postoperative days reduced the rates of sternal wound infection and overall mortality.3,4 Intensive insulin therapy with a target glucose level of lower than 110 mg/dl (6.1 mmol/l) in surgical intensive care patients—of whom approximately 60% had undergone cardiac surgery—led to a marked reduction in mortality rates, principally among patients staying in an ICU for more than 5 days.5 In this study, progressively worse outcomes were seen at higher glucose levels. In a medical ICU setting, the same authors reported a reduction in morbidity with tight glucose control and a benefit in mortality rates in patients whose ICU stay was longer than 3 days.6 This later study also reported a much higher rate of hypoglycemia than was seen in the surgical ICU study. In both trials, nutritional targets were met early, with a high proportion of patients receiving parenteral nutrition. This complicates the applicability of these results to centers with less aggressive feeding practices.
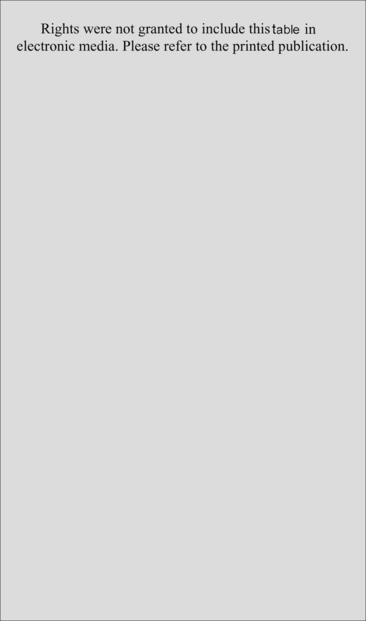
Table 36-1 Adverse Effects of Hyperglycemia
Rights were not granted to include this table in electronic media. Please refer to the printed book.
From Clement S, Braithwaite SS, Magee MF, et al: Management of diabetes and hyperglycemia in hospitals. Diabetes Care 27:553-591, 2004. TNF-α, tumor necrosis factor α; NFκB, nuclear factor kappa B.
Although there is no consensus on a threshold glucose level at which to start insulin in patients in an ICU, tighter glucose control is warranted with increasing severity of illness and anticipated length of ICU stay. Particular care should be taken with tight glucose control regimes to avoid hypoglycemia, especially in patients with variable or unreliable nutritional intakes. However, with appropriate education of staff and frequent bedside monitoring of blood glucose, tight control of blood glucose can be achieved. Except in the setting of myocardial ischemia and reperfusion (see subsequent material), it appears that the benefits arise from better control of hyperglycemia rather than from insulin per se.7,8
Treatment of Hyperglycemia
Oral Hypoglycemic Drugs
Oral hypoglycemic agents are not suitable for the control of hyperglycemia in the ICU. Sulfonylureas have a relatively long and unpredictable duration of action that risks hypoglycemia and hyperglycemia. Metformin reduces cardiovascular risk in outpatients9 but has been associated with potentially fatal lactic acidosis in patients at risk for renal dysfunction and tissue hypoxia,10,11 both of which are very common in the ICU. Thiazolidinediones have a slow onset of action and predispose to systemic edema.
Insulin
Subcutaneous insulin cannot be titrated rapidly, and absorption is unreliable in critically ill patients because of poor peripheral perfusion or edema. Bolus intravenous insulin has a half-time of about 5 minutes and is therefore not suitable for stable blood glucose control. Thus, in the ICU, a continuous intravenous infusion of short-acting insulin should be used to treat hyperglycemia. In patients with type 1 diabetes, insulin therapy should not be discontinued during the postoperative period. In other patients, an insulin infusion should be commenced before the upper limit of the target blood glucose range is reached, typically about 180 mg/dl (10 mmol/l).
Numerous algorithms have been published to guide intravenous insulin dosing. The infusion rate should be determined by the blood glucose level, the rate of change of the blood glucose level, and some measure of insulin sensitivity. The last variable differs from patient to patient, and changes in the same patient at various times during an acute illness.3,5,12 An example of an insulin-infusion protocol that is based on the principles just outlined and that has been validated in the ICU environment is the Yale Protocol.12 A modified version of this protocol is provided in Table 36-1a.
Patients who are well enough to be transferred to the ward and who are eating regular meals can be switched from intravenous to subcutaneous bolus insulin. This may be given as three doses of short-acting insulin (before each meal) plus a dose of intermediate-acting insulin at night. The total daily dosage should be equivalent to the preceding day’s total intravenous dose, with 25% given as intermediate-acting insulin at night and 25% given in each of the three short-acting doses prior to meals. The insulin infusion should overlap with the first subcutaneous dose by 2 hours.1
Hypoglycemia
Symptoms of hypoglycemia may be masked in ICU patients, especially if they are sedated. Neurologic damage can occur when the blood glucose falls to less than 20 mg/dl (1.1 mmol/l). Levels lower than 50 mg/dl (2.8 mmol/l) should be treated with 50 ml of 50% dextrose (via a central vein), temporary cessation of the insulin infusion, and blood glucose testing every 15 minutes until return to stable normoglycemia. Factors likely to require a change in infusion rate should be anticipated. Insulin requirements are reduced by: (1) decreased nutrition delivery or absorption; (2) reduced infusion rates of β2-adrenoreceptor agonists; (3) the introduction of renal replacement therapy with dextrose-free fluid; (4) hepatic failure.6,13 Insulin requirements are increased with parenteral nutrition compared to an equivalent caloric amount of enteral nutrition.
Metabolic Support in Myocardial Ischemia using High-Dose Glucose-Insulin-Potassium Infusions
Myocardial Substrate Metabolism
As part of the stress response to surgery or myocardial ischemia, insulin activity is reduced and circulating levels of catecholamines, growth hormone, glucagon, and cortisol are increased (see Chapter 2). This results in increased myocardial utilization of free fatty acids and reduced utilization of glucose—despite hyperglycemia. In the absence of oxygen, the myocardium ceases to oxidize lactate and may become a net lactate producer (as the result of anaerobic glycolysis).14,15 These changes are potentially harmful to the ischemic myocardium. Increased reliance on free fatty acid metabolism leads to increased oxygen requirements, which may exacerbate ischemia. Ischemia-induced inhibition of β-oxidation leads to the accumulation of the toxic intermediates of fatty acid metabolism such as acyl CoA, which is associated with intracellular calcium overload and membrane instability. During postischemic reperfusion, myocardial fatty acid oxidation is poorly coupled with contractile function so that increased oxygen consumption is not associated with increased myocardial work. The net result is an increase in myocardial oxygen requirements, reduced contractility, and arrhythmias.
Insulin, given in doses sufficient to suppress circulating free fatty acid levels and optimize myocardial glucose uptake (≥ 0.1 units/kg/hr),16 protects ischemic myocardium and reduces reperfusion injury. Reduced mitochondrial fatty acid uptake and increased glucose uptake (with increased adenosine triphosphate [ATP] production resulting from glycolysis) improve membrane function and thereby reduce the risk for arrhythmias. Increased glucose oxidation and anaplerosis (replenishment of citric acid cycle substrates) reduce myocardial oxygen consumption and improve coupling between substrate oxidation and contractile function.
Glucose-Insulin-Potassium Infusions in Cardiac Surgery
High-dose infusions of glucose-insulin-potassium (GIK) have been reported to improve outcomes in patients with unstable angina undergoing urgent coronary artery bypass graft (CABG) surgery17 and in patients with refractory left ventricular failure following CABG surgery.18 The maximum benefit from perioperative high-dose GIK infusion is likely to be obtained if the infusion is commenced prior to the induction of anesthesia, continued for at least 12 hours postoperatively (to cover the period of reperfusion), and combined with tight glycemic control.19,20
Whether high-dose GIK improves outcome in patients with acute myocardial infarction, in addition to the benefit received from reperfusion therapy, is uncertain. Early small trials reported a benefit,21,22 but a more recent large, randomized trial in patients with ST-elevation myocardial infarction reported no benefit.23
One method of administering high-dose GIK is shown in Table 36-2. The principal risks in this technique are hypoglycemia and hyperkalemia, the latter after discontinuation of the infusions and in the presence of renal dysfunction. It is essential that the insulin and potassium supplements be stopped first, then the patient slowly weaned from the dextrose infusion (see Table 36-2).
Table 36-2 Protocol for High-Dose Glucose-Insulin-Potassium Infusion
Initiation of Infusion |
Infusion 1 |
50 units soluble insulin in 50 ml 0.9% saline at a fixed infusion rate of 0.1 units/kg/hr |
Infusion 2 |
500 ml 50% dextrose with 25 mmol KCl, 25 mmol KH2PO4, 20 mmol MgSO4 |
Infusion is commenced at 0.5 ml/kg/hr and adjusted to maintain the blood glucose at 100 to 150 mg/dl (5.6 to 8.3 mmol/l) |
Cessation of Infusions |
Infusion 1 |
Stop insulin infusion |
Infusion 2 |
Replace infusion of 50% dextrose + electrolytes with plain 50% dextrose |
Run at same rate as previous infusion |
Commence 5% dextrose at 1 ml/kg/hr |
Measure blood glucose every 1/2 hr and potassium hourly |
If blood glucose >250 mg/dl (13.9 mmol/l) reduce 50% dextrose by a half |
If blood glucose 200 to 250 mg/dl (11.1 to 13.9 mmol/l) reduce 50% dextrose by a quarter |
Stop 50% dextrose when infusion rate <5 ml/hr |
Continue to check blood glucose and serum potassium hourly for at least 6 hr |
Continue 5% dextrose (1 ml/kg/hr) for at least 6 hr |
DIABETES MELLITUS
About 30% of patients undergoing CABG surgery in the United States have diabetes.24 Diabetic patients undergoing cardiac surgery are more likely to have preexisting hypertension, renal insufficiency, previous myocardial infarction, congestive heart failure, or triple-vessel or left main coronary artery disease. Furthermore, they have higher hospital mortality rates and higher rates of postoperative complications than nondiabetic patients.25 Diabetic patients also have a severalfold higher risk for mortality following acute myocardial infarction as well as an increased incidence of congestive heart failure, reinfarction, and recurrent ischemia.2,11,26 Although the rate of death due to coronary heart disease has declined in the overall population over the past few decades, this decline has been less marked among diabetics.27 Furthermore, the incidences of insulin resistance and type 2 diabetes are increasing in North America.
Type 1 diabetes results from immunologic destruction of pancreatic β cells at a young age. Patients with type 1 diabetes are insulin deficient and require treatment with insulin to avoid ketoacidosis. Type 2 diabetes results from a combination of insulin resistance (due to obesity, physical inactivity, and genetic susceptibility) and an age-related decline in insulin secretion. Insulin resistance is often present for many years before type 2 diabetes becomes apparent. Increased insulin secretion compensates for insulin resistance at first, but as insulin secretion declines, hyperglycemia occurs, initially after meals or during periods of stress and later when fasting. Hyperglycemia can usually be controlled by diet initially but subsequently it is common for patients to require treatment with oral hypoglycemic drugs and eventually with insulin. Insulin resistance is associated with dyslipidemia, hypertension, and a prothrombotic tendency, each of which is an independent risk factor for coronary heart disease.11 Of patients with diabetes mellitus, 90% have type 2.

Full access? Get Clinical Tree
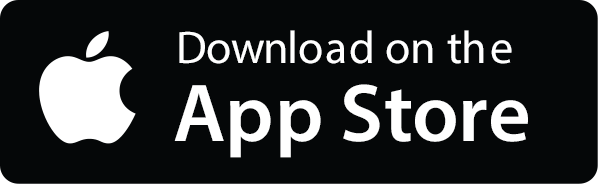
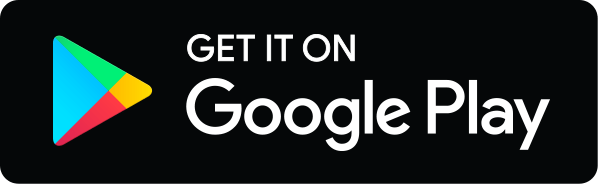