Emerging Technologies
Suneet Mittal
Jonathan S. Steinberg
Andrew Choi
Aysha Arshad
Atrial fibrillation (AF) is the most common sustained arrhythmia encountered in clinical practice. Over the past decade, catheter ablation has become an important therapeutic option in patients with drug-refractory, symptomatic AF (1). While several approaches to ablation have been promoted, pulmonary vein isolation is critical to most ablation strategies (2,3).
However, despite continual improvements in our understanding of AF as well as improvements in ablation technologies, catheter ablation of AF continues to be limited by suboptimal efficacy and a significant risk of major procedural complications (1). These include pulmonary vein stenosis, phrenic nerve palsy, stroke, cardiac tamponade, and atrioesophageal fistula formation (4, 5, 6, 7). The purpose of this review is to highlight several emerging technologies that may help maximize efficacy and minimize adverse events during catheter ablation of AF. These include advances in realtime imaging, alternative energy sources, and novel catheter designs.

Increasingly, cardiac CT or MRI scans are being obtained prior to catheter ablation of AF to delineate the pulmonary venous and left atrial anatomy. These images are then “merged” with real-time 3-D mapping data sets obtained during the ablation itself. However, this approach has several limitations. First, it is costly to obtain these preprocedural radiographic studies. In the case of cardiac CT scans, there is the additional issue of exposure to radiocontrast agent and radiation. Second, the images are often obtained days or weeks prior to the ablation; in the interim, there can be a change in the patient’s underlying rhythm, which can lead to a discrepancy between the previously acquired and real-time data sets. Third, images cannot be updated during the procedure; volume loading (as is common with open-irrigated ablation catheters) and ablation lesions may dynamically alter the left atrial anatomy and make it necessary to have the ability to intermittently update the data.
Real-time imaging during catheter ablation of AF is clearly desirable to the electrophysiologist. Goals of imaging include facilitation of the transseptal puncture, visualization of the pulmonary venous and left atrial appendage anatomy, visualization of the esophagus, early identification of procedural complications (pulmonary vein stenosis, pericardial effusion, thrombus formation), and identification of the
tissue-electrode interface and assessment of the efficacy of delivered lesions (8). To date, real-time imaging has only been possible using intracardiac echocardiography.
tissue-electrode interface and assessment of the efficacy of delivered lesions (8). To date, real-time imaging has only been possible using intracardiac echocardiography.
Intracardiac Echocardiography
The AcuNav (Acuson, Mountain View, CA) ultrasound catheter is the most commonly used intracardiac echocardiography catheter used during catheter ablation of AF. This phased-array system incorporates a 64-element transducer mounted to the end of a 10F catheter. It is capable of providing 2-D imaging as well as full spectral and color Doppler imaging. The imaging plane starts about 2 mm from the tip and extends out to greater than 10 cm (8,9).
A major limitation to currently available 2-D imaging is the need to constantly adjust the catheter (move up and down; rotate clockwise and counterclockwise) to acquire the desired images. Toward this end, 3-D echocardiography holds great promise. Although initial catheter designs were not practical given the need for large transducer sizes (10), technological advances appear to have overcome this limitation (Fig. 17.1) (11).
Recently, the results of a semiautomated 3-D intracardiac echocardiography system were described. This technique displays a 3-D volume of interest without the need to adjust the 2-D plane of the tip of the intracardiac echocardiography catheter (12). In this system, a standard AcuNav catheter is advanced into the mid-right atrium via a 12F long vascular sheath. The ultrasound catheter is attached to a prototype stepping motor for automatic longitudinal catheter rotation in 2° to 5° increments (Fig. 17.2). Within just a few minutes, 3-D reconstructions of relevant anatomic structures can be obtained (Fig. 17.3). Importantly, the ultrasound catheter does not need to be repositioned. This type of system has the potential of providing continuously updated real-time information on intracardiac geometry.
Infrared Imaging
An alternative approach to real-time imaging involves the use of infrared imaging. Cardio-Optics (Boulder, CO) has developed a 7.5F forward-viewing steerable catheter (SiteSeekir), which is capable of seeing through circulating blood in vivo using infrared light in the 1,600 ± 700-nm region. At this wavelength, which is beyond the optical diameter of the red blood cell, scattering is reduced significantly. In this manner, monochromatic images can be obtained, allowing for real-time imaging of relevant cardiac structures. In fact, the feasibility of this system for identifying right atrial structures, including the coronary sinus ostium and its branches, has been demonstrated (13).
One of the major limitations to the current approach to catheter ablation of AF is the difficulty in visualizing the tissue-electrode interface to assess the efficacy of individual ablative lesions. Toward this end, this infrared system may be useful as a gradual increase in tissue signal intensity “whitening” is observed near the electrode at the endocardial surface during ablation (14). Importantly, no imaging artifact is observed during delivery of radiofrequency energy.

The ideal energy source for catheter ablation of AF would have the following characteristics:
The ability to rapidly deliver uniform transmural lesions,
The freedom from dependence on tissue-electrode contact, and
A good safety profile (15).
Although radiofrequency energy is the most commonly used energy source used during catheter ablation of AF, as an energy source it fails to meet any of these desired attributes. Radiofrequency energy is effective only when the ablation catheter is in direct contact with the region of interest; even when delivered, it is difficult to ensure transmurality. In fact, in patients with a recurrence of AF following ablation, resumption of left atrial pulmonary vein conduction is almost always demonstrated (16,17). Delivery of radiofrequency energy in the vicinity of the pulmonary veins is associated with increased intimal thickness, thrombus formation, endocardial contraction, and proliferation of the elastic laminae, which can lead to development of pulmonary vein stenosis or lead to a thromboembolic event (18). Finally, collateral damage to the phrenic nerve and esophagus are feared complications of radiofrequency energy. Clearly, alternative energy sources would be welcome.
Cryoablation
The application of cryothermal energy during ablation results in the formation of an “ice ball” or section of frozen tissue. The main stages of cryothermy include a freethaw cycle, followed by hemorrhage and inflammation, and finally replacement fibrosis (19, 20, 21). In contrast to radiofrequency energy, cryothermal lesions are typically hemispherical in shape, have a sharply defined interface with normal tissue, and retain a well-preserved architecture. These lesions are typically not associated with endothelial injury and therefore minimize the risk of thrombus formation (Fig. 17.4). Additional benefits of cryoablation include the following: stability of the catheter during ablation, safety when ablating in the vicinity of cardiac and vascular structures, and decreased pain perception during energy delivery (22, 23, 24).
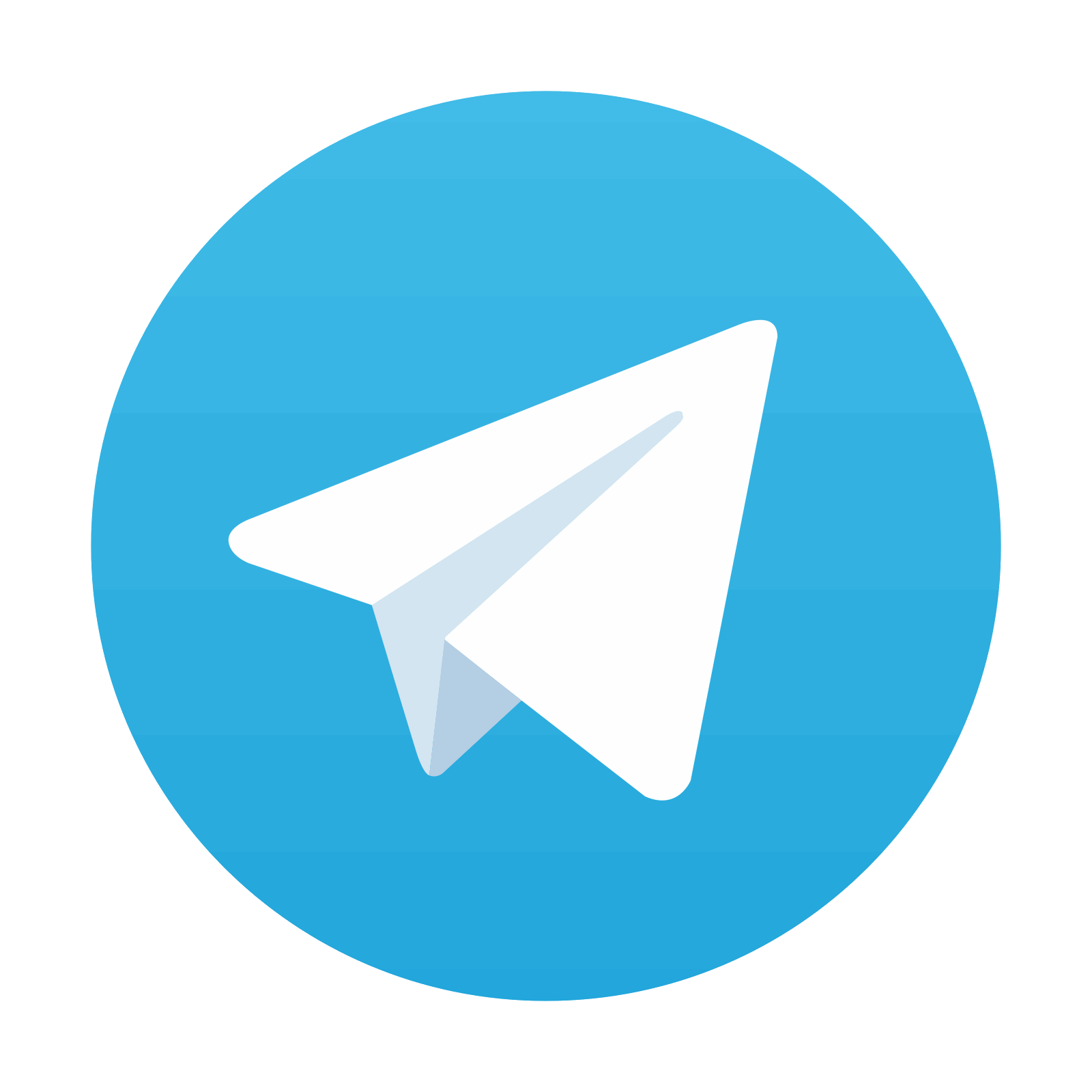
Stay updated, free articles. Join our Telegram channel

Full access? Get Clinical Tree
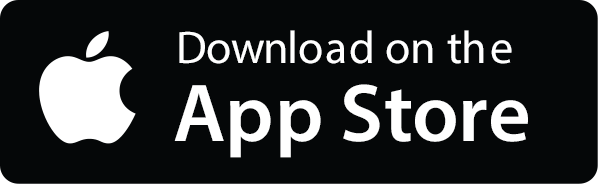
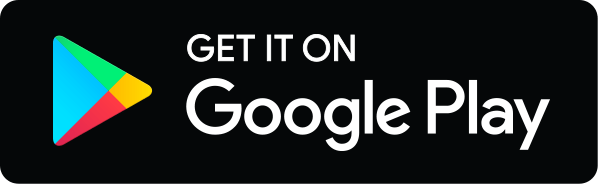