Sudden cardiac death (SCD) is the leading cause of cardiovascular death and remains an important public health issue. The definition of SCD includes death from natural causes within an hour of a change in cardiovascular status and can occur in patients with or without preexisting cardiovascular disease. Incidence estimates for SCD in the United States vary from 250,000 to 450,000 cases a year, with most estimates in the range of 300,000 to 350,000 per year.1 Although the worldwide incidence of SCD is difficult to estimate, several studies have shown the rate of SCD is approximately 50 to 100 per 100,000 people.2 In the United States, SCD accounts for about half of cardiovascular deaths and 15% to 20% of all deaths.2 In this chapter, we will briefly review the most common etiologies of SCD as well as outline treatment strategies for dealing with patients who present with SCD because these commonly fall under the realm of the interventional cardiologist. A thorough knowledge of emergency resuscitation measures is critical for the interventional cardiologist who is often called to potentially treat patients with SCD, whether this occurs out of or within the hospital (even within the cardiac catheterization laboratory).
Diseases of the coronary arteries account for the majority of SCD (Table 64-1). In the Western world, approximately 80% of SCD is caused by coronary artery abnormalities.3 Coronary artery disease can precipitate SCD by several mechanisms. Acute coronary plaque instability leading to cardiac ischemia or infarction can lead to electrical instability and ventricular fibrillation (VF). Previously infarcted myocardium can precipitate ventricular arrhythmias around areas of scar. Adverse remodeling after a myocardial infarction (MI) can create another substrate for ventricular arrhythmias. Furthermore, stable coronary disease can precipitate SCD. In an autopsy study of 90 cases of SCD attributed to coronary artery disease (CAD), 17 of the 90 cases (19%) had no evidence of prior MI or active coronary plaque.4 While the majority of patients with SCD from CAD will have VF as their initial cardiac rhythm, VF will degrade to asystole in a short period of time. Therefore, even though the initial rhythm documented by emergency medical systems can be asystole, coronary abnormalities may still be the primary etiology of the arrest.
Cardiac artery abnormalities Coronary atherosclerotic disease Congenital abnormalities Coronary artery embolism Coronary arteritis Coronary dissection Coronary spasm Coronary bridge Primary electrophysiologic abnormalities Accessory pathways Congenital QT interval disorders Acquired QT interval disorders Brugada syndrome Idiopathic ventricular fibrillation Neurohormonal/central nervous system Catecholaminergic polymorphic ventricular tachycardia Other catecholamine-dependent arrhythmias | Myocardial diseases Left ventricular hypertrophy (primary or secondary) Congestive heart failure (acute or chronic) Inflammatory diseases Myocarditis Sarcoidosis Infiltrative diseases Myocardial tumors Cardiac valve disease Congenital heart disease Sudden infant death syndrome Miscellaneous Aortic dissection Toxic and metabolic disturbance Obstruction to venous return Cardiac tamponade, pulmonary embolism Commotio cordis |
Nonatherosclerotic coronary abnormalities compose a smaller proportion of the etiology of SCD (see Table 64-1). Congenital abnormalities of the coronary arteries are an important cause of SCD. Anomalous coronary arteries are a common cause of SCD in the young, particularly the left coronary artery arising from the right aortic cusp. SCD is often precipitated by exercise in this patient population. Other rare causes of coronary ischemia or infarction leading to SCD include coronary vasospasm, coronary artery dissection, and coronary artery embolism.3 Myocardial bridges, while a common (and often benign) finding in up to 70% in autopsy studies, have in some cases been reported to cause SCD, particularly in those with hypertrophic cardiomyopathy.5
Nonischemic dilated cardiomyopathies are responsible for 10% to 15% of SCD in the United States.3 Several reports have shown that 30% to 50% of all deaths in chronic heart failure are secondary to SCD.6 Ventricular tachycardia (VT) can occur in chronic heart failure during times of stable hemodynamics (scar-mediated ventricular arrhythmias) or during progressive hemodynamic deterioration that may render the myocardium more susceptible to ventricular arrhythmias. Other fatal arrhythmias, including bradyarrhythmias and pulseless electrical activity (PEA), may occur secondary to worsening pump function and decreased cardiac output. Hypertrophic cardiomyopathy is another common cause of SCD, especially in the younger population. Before the advent of modern treatments for patients with valvular diseases, many died from SCD; however, recently the risk has decreased substantially. Virtually all infiltrative and inflammatory myocardial diseases have been shown to cause SCD.
In approximately 5% of SCD cases, there are no identifiable cardiac abnormalities on autopsy7; however, in a younger population, this percentage may be higher.8 Among these, inherited channelopathies and other primary electrophysiologic abnormalities have been implicated in SCD. These include long and short QT syndromes, Wolf-Parkinson-White syndrome, Brugada syndrome, catecholaminergic polymorphic VT, and idiopathic VF.9 Many of these syndromes are familial, and appropriate family member screening is essential in preventing SCD.
In the general population without known cardiovascular disease, the risk factors for SCD parallel those of CAD including age, cigarette smoking, hypertension, hyperlipidemia, diabetes, obesity, sedentary lifestyle, and family history of premature CAD. A family history of SCD infers a 1.5-fold increase in SCD. However, in patients with diagnosed CAD, these risk factors typically no longer hold prognostic significance for SCD.10 Instead, the left ventricular (LV) ejection fraction seems to be the most powerful predictor of first-episode SCD and is currently used to risk stratify patients for primary prevention implantable cardiac defibrillator (ICD) therapy.11 Vigorous exercise has been identified as a risk for SCD; however, the overall risk is very low.12 Patients with prior MI, frequent premature ventricular contractions, and in particular nonsustained VT have a higher risk of SCD.
The initial role of healthcare providers and the general population is to recognize and diagnose cardiac arrest, followed by the initiation of basic life support (BLS). The initial evaluation needs to be very brief, and the BLS guidelines from the American Heart Association (AHA) recommend that the initial evaluation of an unconscious man should only include checking for breathing. A pulse check is no longer recommended for the general population.13 After recognition of cardiac arrest, emergency medical systems need to be activated immediately, followed by initiation of cardiopulmonary resuscitation (CPR). High-quality minimally interrupted CPR appears to improve time to return of spontaneous circulation (ROSC) and neurologic recovery in both out-of-hospital and in-hospital cardiac arrest.14-16 Pulse checks and rhythm evaluations should only occur during preset intervals (every 2 minutes) and should only delay CPR for a maximum of 10 seconds.
The concept of cardiocerebral resuscitation, where rescue breathing does not interrupt CPR, is based on the finding that high-quality CPR with minimal interruptions improves outcomes in cardiac arrest patients. A meta-analysis of 3 randomized trials comparing compression-only CPR with standard CPR found a statistically significant 22% improvement in survival (Fig. 64-1; risk ratio 1.22; 95% confidence interval [CI], 1.01-1.47).17 However, a secondary analysis of nonrandomized cohort studies found no benefit for compression-only CPR.17 It must be noted that this method of CPR is not recommended for children or suspected noncardiac causes of cardiac arrest. While the 2010 AHA guidelines state that compression-only CPR can be used when initial responders are reluctant to perform mouth-to-mouth ventilations, the recommended approach is still to perform 30 compressions followed by 2 rescue breaths.13 Because the oxygen level in the pulmonary vasculature is likely adequate during the first minutes of cardiac resuscitation, initial rescue breaths are no longer recommended. After the placement of an advanced airway, asynchronous breaths should be performed at a rate of 8 to 10 breaths per minute with no interruption in CPR. Excessive ventilation can be detrimental as animal models have shown that overventilation can lead to reduced success of defibrillation and decreased survival.18
Figure 64-1
Meta-analysis of 3 randomized trials comparing survival to hospital discharge between compression-only cardiopulmonary resuscitation (CPR) and routine basic life support. (Reprinted from Hupfl M, Selig HF, Nagele P. Chest-compression-only versus standard cardiopulmonary resuscitation: a meta-analysi. Lancet. 2010;376:1552-1557. Copyright © 2010, with permission from Elsevier.)

Automated chest compression devices have been developed as an alternative to manual chest compressions during cardiac arrest (Fig. 64-2). These devices can include circumferential compression around the chest wall or a piston placed at the sternum to perform compressions in the anteroposterior plane. Several of these devices contain both radiolucent as well as radiopaque components, allowing them to be used within the cardiac catheterization laboratory while performing fluoroscopic procedures. Typically, the views required are angulated views (either cranial or caudal with additional left anterior oblique or right anterior oblique angulation). A meta-analysis of randomized trials found that devices using piston-delivered CPR had the same rate of ROSC compared to conventional CPR, whereas devices using load-distributing band CPR did have a better rate of ROSC.19 A subsequent large randomized trial found no improvement in survival using a piston-based mechanical CPR device over traditional CPR.20
Another alternative method of CPR, called active compression-decompression CPR (ACD-CPR) uses a suction device to convert the passive recoil of a compressed chest wall into active expansion, improving venous return (see Fig. 64-2). A Cochrane review of randomized data including approximately 5000 patients found no benefit with ACD-CPR when compared to standard CPR.21 As such, the guidelines state there is insufficient evidence to support the use of ACD-CPR.22 Interposed abdominal compression CPR (IAC-CPR) involves the use of a second rescuer performing mid-abdominal compression during the upstroke of chest compression. A meta-analysis found that in 34 of 38 studies, including animal and mechanical model studies, there was an improvement in ROSC with IAC-CPR compared to regular CPR.23 There were 4 prospective studies included, and 3 of them found significant improvements in ROSC with the use of IAC-CPR, with 1 of these studies showing improved survival. These 3 studies included in-hospital arrest, while the fourth study that did not find a difference was in out-of-hospital arrests.23 Given these initial data, the guidelines state that IAC-CPR can be considered by trained practitioners during an in-hospital arrest.22
Inspiratory impedance threshold devices (ITDs) allow positive-pressure ventilation but prevent airflow during chest relaxation, thus increasing negative intrathoracic pressures during chest decompression while performing CPR. This increase in negative intrathoracic pressures improves preload and myocardial perfusion. A meta-analysis of randomized trials examining the role of ITD found that the addition of ITD to standard CPR or device-assisted CPR resulted in improved rates of ROSC and early survival; however, longer term survival was not improved.24 Therefore, current guidelines state there is insufficient evidence to support or refute the use of ITD.22
Survival of patients with cardiac arrest relies on high-quality CPR as well as early defibrillation. An initial chest thump, also called a precordial thump, may be attempted to quickly restore electrical stability. The technique involves 1 or 2 blows at the junction of the middle and lower third of the sternum from 8 to 10 inches away. It should not be used for tachycardias without a loss of consciousness. The initial experience of the chest thump was mixed, with some studies showing successful conversion of VT/VF using the technique. However, a subsequent trial found that in 103 patients treated with a precordial thump as the initial strategy, a rhythm change was noted in 17. Only 5 of the cases had ROSC, whereas 10 others had a deterioration in the cardiac rhythm.25 Given these conflicting data, the precordial thump is not considered an alternative to conventional treatments such as expedited defibrillation.
Many researchers have adopted the 3-phase model for resuscitation after cardiac arrest.26 The initial phase, or electric phase, occurs during the first 4 to 5 minutes after arrest, and immediate defibrillation is essential in the survival of these patients. The second phase, the hemodynamic phase, usually consists of minutes 4 to 10 after cardiac arrest. During this phase, some have theorized that it might be better to improve oxygen delivery with high-quality CPR followed by defibrillation. While this theory is supported in animal models,26 randomized trials employing a strategy of 2 to 3 minutes of CPR prior to defibrillation have yielded inconsistent results. One trial of 200 cardiac arrest patients found an improvement in survival in a CPR-first strategy when the response time of the ambulance was greater than 5 minutes,27 whereas 2 other studies found no improvement in survival.28,29 Notably, current guidelines state there are insufficient data to support the use of 2 to 3 minutes of CPR prior to defibrillation if immediately available.30 The third phase, the metabolic phase, occurs after 10 minutes of cardiac arrest. During this phase, early CPR and defibrillation are less effective, and outcomes are very poor. In these cases, if ROSC is obtained quickly, postresuscitative treatment is essential in improving outcomes.
While defibrillation is essential for ROSC and early defibrillation improves outcomes, interruptions of high-quality CPR must be kept as minimal as possible. Studies have shown that interruptions of CPR for defibrillation can worsen outcomes.31 Current guidelines recommend CPR be initiated while the defibrillator is being set up and interruptions kept to a minimal. CPR should stop for a rhythm check, followed by defibrillation if necessary, and immediate resumption of CPR prior to another rhythm check. Only a single shock for VT or VF from either an automated external defibrillator (AED) or monophasic or biphasic defibrillator30 should be used before resuming CPR. Biphasic defibrillators are preferred because of the ability to perform defibrillation with lower energy levels (200 J compared to 360 J with a monophasic defibrillator).
Given the importance of time to defibrillation as a predictor of outcomes, the use of AEDs provides promise for significantly improving outcomes in victims of SCD. The technology of AEDs has improved, and recent models can weigh as little as 2 to 4 kg and cost under $1000. While initial data regarding the use of public access AEDs were uncertain, larger trials have since shown impressive rates of survival when AEDs are used in airports, airplanes, casinos, and police vehicles.3 A randomized trial studying placement of AEDs in general community sites demonstrated a significant improvement in survival to hospital discharge when a trained first responder used an AED as opposed to CPR alone (23% with AED use, 14% with CPR alone).32 These results have led to the increased use of AEDs deployed in high-risk areas. Despite this deployment, public access AEDs are only used in a small proportion of out-of-hospital cardiac arrests,33 although with increasing awareness, their use should grow.
After initial resuscitative measures are under way, the next step in management is to obtain stable ROSC and stable hemodynamics. Intravenous (IV) or intraosseous (IO) access should be obtained. If the initial rhythm is VT or VF, CPR should continue in 2-minute cycles with rhythm and pulse checks afterward with subsequent defibrillation for recurrent VT/VF. Although there is minimal randomized evidence to support its use, epinephrine (1 mg IV/IO) is recommended every 3 to 5 minutes. Several recent observational studies have questioned the utility of epinephrine for out-of-hospital cardiac arrest34,35; however, these studies are by definition confounded by indication (ie, patients receiving epinephrine are inherently sicker than those who do not receive it). Higher dose epinephrine is not recommended because trials have failed to show improvements in survival compared with standard-dose epinephrine.36,37 Vasopressin (40 mg IV/IO) can be given in place of a dose of epinephrine.38 While a randomized trial showed superiority with this strategy over epinephrine alone,39 a subsequent meta-analysis of several randomized trials found no benefit.40 Another randomized trial that took place after the meta-analysis found that a combination of vasopressin, epinephrine, and steroids (methylprednisolone 40 mg IV once) improved survival compared to epinephrine and placebo (21% survival with favorable neurologic outcome in the combination group vs 8% in the epinephrine plus placebo group).41 The conflicting data regarding the use of vasopressors underscore the importance of high-quality CPR and defibrillation to optimize outcomes for cardiac arrest patients.
Antiarrhythmic therapy can be effective in the treatment of refractory VT/VF. Amiodarone (300 mg initial bolus followed by 150 mg bolus as clinically indicated) is the preferred antiarrhythmic.38 The ARREST trial showed an improvement in survival to hospital admission with amiodarone use compared with placebo (44% vs 34%), at the cost of an increase in bradycardia and hypotension requiring treatment.42 The ALIVE trial found amiodarone to be superior to lidocaine (1-1.5 mg/kg IV followed by 0.5-0.75 mg/kg every 5-10 minutes).43 Magnesium sulfate (2 g IV) can be used to treat torsade de pointes44; however, it is not recommended for routine use in VT/VF.
When the initial rhythm is asystole or PEA, no defibrillation is advised, and high quality CPR should be initiated with rhythm and pulse checks every 2 minutes. In this situation, the most critical role of the physician is to reverse any underlying cause of the arrest. Much like in VT/VF, evidence supporting the use of epinephrine and vasopressin during PEA or asystole is lacking; however, guidelines recommend the use of epinephrine (1 mg IV/IO every 3-5 minutes) with vasopressin (40 mg IV/IO) as an alternative to one of the epinephrine doses.38 Atropine is no longer recommended for PEA or asystole, as recent trials have shown no improvement in outcomes and a potential signal of harm.45 While transcutaneous pacing can be temporarily effective in patients with bradycardia, it is usually not effective and therefore not recommended in treating patients with asystole who typically require transvenous pacing.46
Despite many advances in the treatment of heart disease, the outcomes after cardiac arrest remain poor. A changing cardiac arrest population with older age and less VF may offset improvements in bystander CPR rates and shorter times to defibrillation.47 The etiology of the cardiac arrest is linked to subsequent survival, with very few patients with asystole surviving to hospital admission or discharge and as few as 11% of patients with PEA surviving to discharge.48 On the contrary, reports of survival with VF are better than 25%.47
Initial emergency medical care systems involved training firefighters in the treatment of cardiac arrest patients, including the use of defibrillators. Initial reports from Miami, Florida, and Seattle, Washington, during the 1970s reported initial out-of-hospital cardiac arrest survival rates of 14% and 11%, respectively. Adding emergency medical technicians as trained first responders increased these rates of survival to close to 30% in the late 1980s. More recently, overall survival rates have decreased across the United States, likely due to the addition of emergency medical systems in rural areas and increased population density in urban areas (ie, a greater denominator of patients noted to undergo emergency resuscitation measures).49 Significant regional variability exists, with 1 study reporting survival to discharge after all cardiac arrests ranging between 3.0% and 16.3% with survival rates of VF arrests between 0% and 39%.50 This large variability underlies the importance of community efforts to optimize the treatment of SCD.
The primary efforts to improve outcomes in cardiac arrest include easy access to emergency medical systems (eg, through calling 9-1-1), training community members to perform bystander CPR, AED placement in public areas, dispatcher-assisted CPR, trained first responder defibrillation, and improvements in advanced cardiac life support (ACLS) from healthcare workers.51 These changes have improved outcomes in many emergency systems like those in Seattle, Washington, where overall survival rates are nearly double those of the nation.50 The emergency medical system there has made several changes, including equipping fire fighters with AEDs for rapid response to patients with cardiac arrest. In addition, there is a multilevel review process evaluating the response to cardiac arrests.52
Partly based on this paradigm and others like it, the AHA has subsequently released a “chain of survival” designed to help improve outcomes in all communities. This chain includes immediate recognition of cardiac arrest and activation of the emergency response system, early CPR with emphasis on chest compressions, rapid defibrillation, effective ACLS, and an integrated post-cardiac arrest care. In addition, a thorough review process to evaluate the response of emergency medical systems is vital to improving outcomes in out-of-hospital cardiac arrests.52
Upon arrival to the hospital, the initial management for victims of SCD concentrates on stabilization of the patient and determining the cause of the arrest. Prompt electrocardiography is recommended in order to diagnose the postreperfusion rhythm and also to determine whether the primary etiology of SCD is related to acute MI. Depending on the arrest time, the initial electrocardiogram (ECG) may represent severe acidosis with attendant hyperkalemia, and thus serial ECGs are often indicated after reversal of these metabolic abnormalities. Adequate ventilation must be maintained to ensure oxygen saturations greater than 94%, with mild permissible hypercapnia to prevent the physiologic cerebral vasoconstriction in response to hypocapnia.53 Hemodynamics need to be optimized with mean arterial pressures (MAP) of at least 65 mmHg, preferably even 80 to 100 mmHg, in order to maintain cerebral perfusion and reverse any ischemic consequences of the cardiac arrest. An adequate volume status should be maintained with goal central venous pressure of 8 to 10 mmHg.54 In addition to hemodynamic support, stabilization of the cardiac rhythm is important, and patients with recurrent VT/VF should be treated with antiarrhythmic therapies (eg, amiodarone) as well as consideration for emergent coronary angiography (see below).
Routine laboratory tests can elucidate reversible causes of cardiac arrest, including electrolyte abnormalities. Prompt echocardiography can be very useful in order to determine the etiology of cardiac arrest, although global left ventricular dysfunction may be a common finding, particularly for patients with longer times to ROSC. Empiric use of transvenous pacemakers for those with atrioventricular or intraventricular conduction abnormalities have not improved outcomes and are only indicated for severe symptomatic heart block. Empiric use of fibrinolytics in cardiac arrest victims does not appear to improve survival.55 However, use of fibrinolytics when pulmonary embolism is the presumed cause may be beneficial and is recommended.
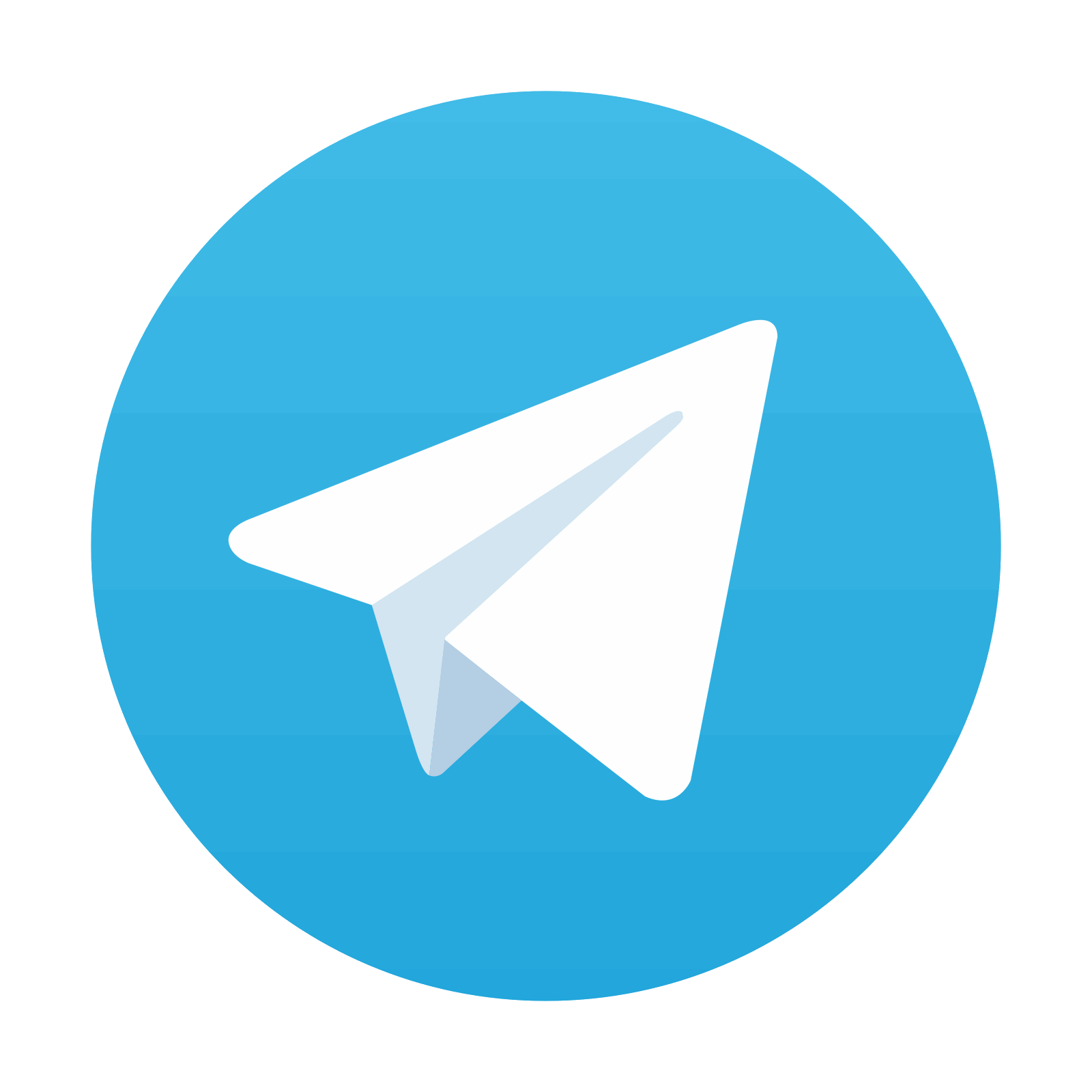
Stay updated, free articles. Join our Telegram channel

Full access? Get Clinical Tree
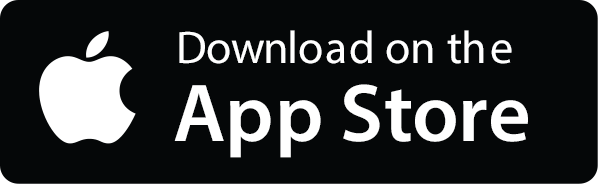
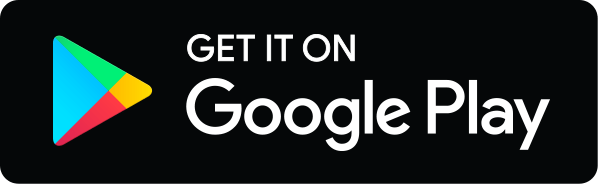