Fig. 11.1
(a, b) (EPSS normal, abnml). End point septal separation (EPSS) is the use of M-mode in the parasternal long cardiac window to evaluate the distance between the anterior leaflet of the mitral valve and the interventricular septum in end diastole. EPSS >1.2 cm is considered abnormal and is associated with poor ejection fraction. IVS interventricular septum, * Anterior mitral valve leaflet, A–A EPSS

Fig. 11.2
(a, b) (FS nml, abnml). Fractional shortening (FS) is the use of M-mode in the parasternal long cardiac window to measure the degree of left ventricle (LV) chamber shortening between diastole and systole. FS is expressed as a percentage by calculating the difference between end-diastolic LV diameter and end-systolic LV diameter. The difference is then divided by the end-diastolic LV diameter and multiplied by 100. Some ultrasound machines possess software that will convert FS% into EF as is recorded in this image. IVS interventricular septum, LV left ventricle, LVPW left ventricle posterior wall, A to A LV end-systolic diameter, B to B left ventricle end-diastolic diameter

Fig. 11.3
(a, b) (nml, plethoric). A longitudinal view of the inferior vena cava (IVC). A plethoric IVC shows limited to no respiratory variation and is associated with AHF

Fig. 11.4
(A lines). A lines. The hyperechoic pleural line with reverberation artifact of the pleura producing equally spaced, repeating hyperechoic horizontal lines referred to as A lines may be seen in normal lung or in pathologic conditions such as chronic obstructive pulmonary disease (COPD) or pneumothorax. A lines are not seen well in acute exacerbations of heart failure as B lines (see Fig 11.5) erase or obscure A lines.

Fig. 11.5
(B lines). B lines are vertical hyperechoic lines arising from the pleural line and extending to a depth of at least 18 cm. Greater than three B lines in a single intercostal space is considered abnormal: Image “a” shows two prominent B lines extending down from the pleural line. Image “b” shows confluent B lines extending down from the pleural line, appearing more as a hyperechoic or bright white sheet than as individual B lines. The confluent B lines (or B lines that merge into one another) are indicative of more significant interstitial fluid than is present when individual B lines can be identified.

Fig. 11.6
The spine can been seen superior or cephalad to the diaphragm (in this image, to the left of the diaphragm is superior/cephalad in the body) due to the presence of significant pleural fluid that provides an excellent acoustic window for viewing deeper structures such as the spine. When aerated lung alone is present and there is no pleural fluid, the spine is not visible superior to the diaphragm because the air within the lung scatters the sound waves, not allowing the sound waves to reach the deeper spine.
Visual estimation of ejection fraction (EF) is widely used and considered comparable to calculated EF when performed by providers experienced in POCUS [10].
Visual estimation of EF by both cardiologists and EPs has been shown to correlate well with quantitative assessments of EF [11, 12]. A recent study demonstrated a sensitivity of 98.7 % and a specificity of 86.2–87.9 % for low EF when two EPs used visual estimation to evaluate EF when compared to a gold standard of cardiologists using the modified Simpson method of EF calculation [12]. Randazzo et al. used a subjective visual estimation technique of LVEF in patients who were scheduled to receive a formal echocardiogram (echo) from the ED. A diverse group of providers that included attendings, residents, and a physician assistant used visual estimation to classify EF as poor, moderate, or normal. The overall agreement in estimation of function between the EPs and cardiologists was good (r = 0.712) [13]. Fractional shortening and the Teichholz method for estimation of EF in M-mode imaging are alternative methods of measuring EF [1].
While detection of reduced EF is extremely helpful, use of reduced EF alone as an echo variable for predicting AHF would result in failure to detect the nearly half of all HF patients with preserved EF [14]. The diagnosis of heart failure with preserved EF, previously referred to as diastolic heart failure, is complex and incompletely agreed upon by experts [15]. The use of tissue and flow Doppler in the diagnosis of heart failure with preserved EF is not an expected EP skill. However, the scope of POCUS is always expanding as providers attain mastery of additional ultrasound exams. One recent publication detailed a focused protocol for diagnosis of heart failure with preserved EF that could be performed in the emergency department [16]. One study found EP-performed echocardiograms to have a high sensitivity (92, 95 % confidence interval, 60–100) but moderate specificity of 69 % (95 % CI, 50–83) in identifying clinically significant diastolic function [17]. Another study found the evaluation could be performed by EPs in under 10 min and yield a sensitivity of 89 % and a specificity of 80 % for diastolic dysfunction [10, 18].
A few studies have employed echo to evaluate the acute impact of HF treatment on structural and functional changes. Some studies found changes in invasive hemodynamics correlated with changes in echo parameters during treatment of HF, specifically change in right atrial (RA) pressure with change in inferior vena cava diameter (IVCd) and change in pulmonary capillary wedge pressure (PCWP) with change in IVCd and IVC collapsibility [19]. Several early studies of HF demonstrated acute reduction in LV size with concomitant decrease in left-sided filling pressures [20–24] although others did not confirm this finding [25, 26].
Ultrasound of the Inferior Vena Cava
If bedside assessment of the inferior vena cava (IVC) functions as a rapid noninvasive means for clinicians to determine a patient’s right atrial pressures, it may be a tool to help differentiate decompensated from compensated HF in the absence of other conditions that raise RA pressure [27].
Evaluation of the IVC is routinely performed with the patient supine or minimally inclined [28]. A subxiphoid or right lateral view of the IVC approximately 2–4 cm proximal to the entrance of the IVC into the RA is assessed for changes in diameter as the patient breathes.
IVCd is thought to be easy to measure in patients with HF and has low interobserver variation [22]. Studies in patients without HF have shown a moderate to high degree of inter-rater reliability for IVCd measurement [29, 30]. One issue that arises when discussing IVC imaging is the significant heterogeneity in how IVC measurements are made. One study evaluated the inter-rater reliability of several methods of IVCd measurement and found the best inter-rater reliability using the anterior midaxillary longitudinal approach with the liver as an acoustic window as compared to both longitudinal and transverse subxiphoid IVC measurements [31].
The IVC collapsibility index (CI) is calculated by determining the difference between the maximum (IVCdmax) and minimum IVC diameter (IVCdmin) via the following formula CI = (IVCdmax-IVCdmin)/IVCdmax. At the extremes, a low collapsibility index indicates that there is very little change in the IVC diameter with respirations, while a high CI occurs when there is significant respirophasic change in the IVC diameter [32].
The IVC diameter changes in response to both intrathoracic and intra-abdominal pressure variations and right atrial (RA) volume. Generally, in the spontaneously breathing patient, the negative intrathoracic pressure produced by inspiration increases venous return to the right atrium, producing a transient decrease in IVC diameter that reverses as the positive intrathoracic pressure associated with expiration limits venous return to the heart.
In patients with left heart failure or other obstructive conditions (cardiac tamponade, massive pulmonary embolism, right heart failure, tension pneumothorax), poor forward movement of blood leads to rising RA pressures. With elevation of RA pressures, the diameter of the IVC increases and respirophasic changes in the IVC diameter become limited. At the extreme, heart failure results in a plethoric IVC that is dilated and has minimal to no change in diameter with respirations.
Consequently, ultrasound imaging of the IVC may offer noninvasive information regarding RA pressure [33–41]. Kircher et al. reported that CI >50 % was indicative of right atrial (RA) pressures <10 mmHg, whereas CI <50 % indicated RA pressures >10 mmHg [33]. In another study, CI <20 % during passive respiration and CI <40 % during forceful inhalation were both predictive of RA pressures >10 mmHg as measured during right heart catheterization. The IVC has been shown in a number of studies to also correlate to PCWP as well [38].
Besli found that mean IVC diameter is significantly greater in patients with systolic HF than those without HF. Additionally, IVCd was significantly greater in patients with decompensated systolic HF when compared to those with compensated HF. Likewise, the percentage of patients with no IVC diameter variability with respirations was 36 % in patients with decompensated HF versus 5 % in those with compensated HF [42]. Thus IVC imaging may help differentiate between patients with decompensated and compensated HF [42].
IVC imaging has also been employed to help elucidate the cause of dyspnea in patients presenting to the ED. Miller et al. evaluated patients with undifferentiated dyspnea using IVC measurements [43]. They used the caval index which is similar to the CI and is expressed as a percentage via the formula (IVCe − IVCi)/IVCe × 100 % where IVCe and IVCi are the diameters of the IVC in expiration and inspiration, respectively. His group found that a caval index of less than 33 % yielded a sensitivity of 80 % and a specificity of 81 % for the detection of AHF in patients with dyspnea. Furthermore, extremely low caval indices of less than 15 % carried a high likelihood ratio (LR) of greater than ten that the patient’s dyspnea was due to AHF.
Another ED-based study of undifferentiated dyspnea had very similar results. A low IVC variability (15 % or less) in patients with dyspnea predicted a causative etiology of AHF with 92 % sensitivity and 84 % specificity. The static measure of absolute IVC diameter did not differentiate dyspnea due to AHF from other causes [27]. In both of these studies, as IVC diameter variability decreased, the likelihood increased that a patient’s dyspnea was due to AHF [27, 43].
Evaluation of the IVC may be prognostic as well as diagnostic. Pellicori et al. suggest that increasing IVCd is associated with worse prognosis in patients with HF regardless of their LVEF [44]. Patients in the highest tertile of IVC diameter had approximately a 40 % risk of an adverse event within the first year and patients with HF in the lowest tertile of IVC diameter had a similar outcome to subjects without HF [44].
US exam of IVCd and collapsibility has been used in diagnosis and therapy of chronic HF with the assumption that IVCd reflects RA pressure [33, 45–47]. Yavasi et al. [48] found that the mean IVC-CI increased significantly with treatment of HF. After the therapy, there was no significant difference between the IVC-CI of the HF patients and controls. The study suggested that treatment response could be better monitored via serial measurements of IVC-CI than serial NT-proBNP levels as there was no significant change in BNP after the therapy.
The IVC-CI shows promise as a diagnostic aid in identifying decompensated heart failure as the cause of dyspnea in patients presenting to the ED and monitoring treatment of ADHF [27, 43, 48]. Standardization of the exact part of the IVC chosen for measurement and patient position at the time of measurement would help produce results that are more easily compared and grouped for analysis.
Lung Ultrasound
Air scatters sound waves. Consequently, air-containing structures such as the lungs were not considered to be amenable to evaluation with ultrasound. Lung ultrasound (LUS) has gained broader application due to a greater understanding of the artifacts generated by the interaction of the US and lung structures and content [28].
Lung ultrasound can be done with the standard cardiac probe or any other low-frequency probe, along the intercostal spaces. Additionally, a high frequency probe can also be used to visualize the pleura but will not provide the necessary depth to evaluate for certain diagnostic artifacts such as B lines. Bilateral hemithoraces are scanned along the anterior chest upper and lower halves as well as laterally superior to the diaphragm [49].
In ultrasound images, the pleural line is represented as a hyperechoic line just deep to the soft tissue of the thoracic wall. In normal conditions, sliding of the parietal and visceral pleura against one another can be seen with respirations.
A lines are produced by a reverberation artifact and appear as multiple, relatively evenly spaced horizontal lines that are parallel to the pleural line [50].
When interstitial fluid is present, the increased alveolar edema produces characteristic artifacts known as B lines that consist of hyperechoic vertical lines which arise from the visceral-parietal lung interface, extend to a depth of at least 18 cm, move with respiration, and erase A lines. Isolated B lines can be found in normal lung, especially in the more dependent regions. On the contrary, three or more B lines per intercostal space are considered abnormal and consistent with the diagnosis of alveolar interstitial syndrome (AIS). AIS can be focal and unilateral, as in pneumonia or pulmonary contusion, or diffuse and bilateral as in pulmonary edema or acute respiratory distress syndrome (ARDS) [41, 49].
LUS easily detects pleural effusion, a pathologic finding frequently present in ADHF. A pleural effusion appears as an anechoic area in the dependent area of the thorax, delimited inferiorly by the diaphragmatic dome and superiorly by the aerated lung [41, 50, 51].
LUS may be used to distinguish between AHF and other noncardiac causes of dyspnea, particularly the COPD exacerbation [28, 41, 52–54].
Evaluation for both B lines and pleural fluid facilitates that differentiation. B lines are strongly associated with other indices of congestion (i.e., radiographic, measurement of extravascular lung water by the dilution technique, PCWP, echocardiographic, and intrathoracic impedance monitoring) [55–58]. Consequently, lung ultrasound can provide direct insight into the pulmonary interstitium [58–62].
A meta-analysis of seven studies, the majority conducted in the ED or ICU, showed that, in patients with acute dyspnea, lung ultrasound for B lines has a sensitivity of 94.1 % and specificity of 92.4 % for the diagnosis of acute cardiogenic pulmonary edema [53]. Another study in the ED population showed a sensitivity of 86 % and specificity of 98 % for lung US in diagnosis of diffuse AIS [60].
Lung US also detects and quantifies pleural effusion with a higher sensitivity and specificity than plain radiographs. Finding a pleural effusion in a patient with multiple and bilateral B lines can significantly increase the probability of a cardiogenic cause of dyspnea [41].
A multicenter prospective trial was conducted in seven EDs to evaluate the diagnostic accuracy of different approaches to evaluation of the dyspneic patient [54]. Specifically, they hypothesized that adding LUS to routine clinical assessment and chest radiography would increase EPs’ ability to distinguish ADHF from non-cardiogenic dyspnea. It was a robust study that included over 1000 patients who were evaluated and scanned by 62 EPs in both community and academic hospitals with each EP enrolling a median of 42 patients. Each patient was scanned in six thoracic zones (three per hemithorax): the second intercostal space at the midclavicular line, the fourth intercostal space at the anterior axillary line, and the fifth intercostal space at the posterior axillary line. LUS was considered positive for diffuse interstitial syndrome if two or more zones bilaterally showed the presence of at least three B lines. The LUS-implemented approach had a significantly higher accuracy (sensitivity 97 %, specificity 97.4 %) in differentiating ADHF from noncardiac causes of acute dyspnea than the initial clinical workup (sensitivity 85.3 %, specificity 90 %) and also greatly outperformed chest radiography alone (sensitivity 69.5 %, specificity 82.1 %) and natriuretic peptides (sensitivity 85 %, specificity 61.7 %, n = 486). One in five patients was reclassified into the correct diagnosis after LUS was performed (net reclassification index of LUS compared with standard workup = 19.1 %).
The Pivetta study is among several that show that LUS can be implemented by nonexperts and, consequently, that the diagnostic results achieved in these studies can be generalized to other settings [52, 54, 63]. It has been shown that LUS can easily be learned through short didactic sessions [63]. Even in the hands of novice sonographers, LUS can be a reliable tool to predict cardiogenic dyspnea [52, 63]. Furthermore, these studies demonstrate a high interobserver agreement between inexperienced and expert sonographers when interpreting LUS images. One of the studies also suggests that an abbreviated two-zone scan of the lateral and inferior lung zones bilaterally has similar sensitivity and specificity to the eight-zone scan detailed by Volpicelli and generally accepted as the standard in LUS [52, 60].
Missed or delayed diagnosis of ADHF in the ED is associated with prolonged hospital stay, higher rates of ICU admission, higher mortality, and increased costs [64–66]. In patients with heart disease, lung US may provide information about prognosis. Frassi et al. [67] reported that B lines were associated with a twofold increase in the rate of death, myocardial infarction, or HF hospitalization (HR 1.9, 95 % CI 1.1–3.4) at follow-up. In an outpatient study of HF patients, a higher number of B lines on LUS identified patients with a fourfold risk of death or HF hospitalizations and a greater than threefold risk of urgent HF visits, HF hospitalizations, or death from any cause over 6 months, independent of risk factors such as age, sex, NYHA class, and clinical congestion score [68]. Furthermore, this study found that only one in five patients had crackles on exam despite being in the group with the greatest number of B lines. This finding supports the use of LUS as a sensitive predictor of subclinical pulmonary congestion in HF. In both studies, lung US predicted relevant clinical outcomes, including death and incident cardiovascular events [67, 68].
Finally, because of the ability of LUS to show rapid extravascular water variation [69], it is a precious aid in assessing the extent of pulmonary congestion and may be used not only in the diagnosis of ADHF but also in monitoring the response to diuretic treatment. Lung US is able to capture changes in congestion status after intravenous diuretic therapy in decompensated HF [70]. Additionally, lung imaging enables a more direct assessment of extravascular lung water than indirect measures. Coiro et al. [71] evaluated the prognostic significance of quantification of B lines in patients discharged after acute HF compared with other classical congestion markers. The major finding of this study is that residual pulmonary congestion, as easily assessed by lung ultrasound (quantified as greater than 30 B lines), at discharge is an independent predictor of both short-term mortality and hospitalization for worsening HF.
Multisystem Ultrasound
The prior studies looked at each organ individually and reported various specificities in differentiating ADHF from other causes of dyspnea [1, 72–74].
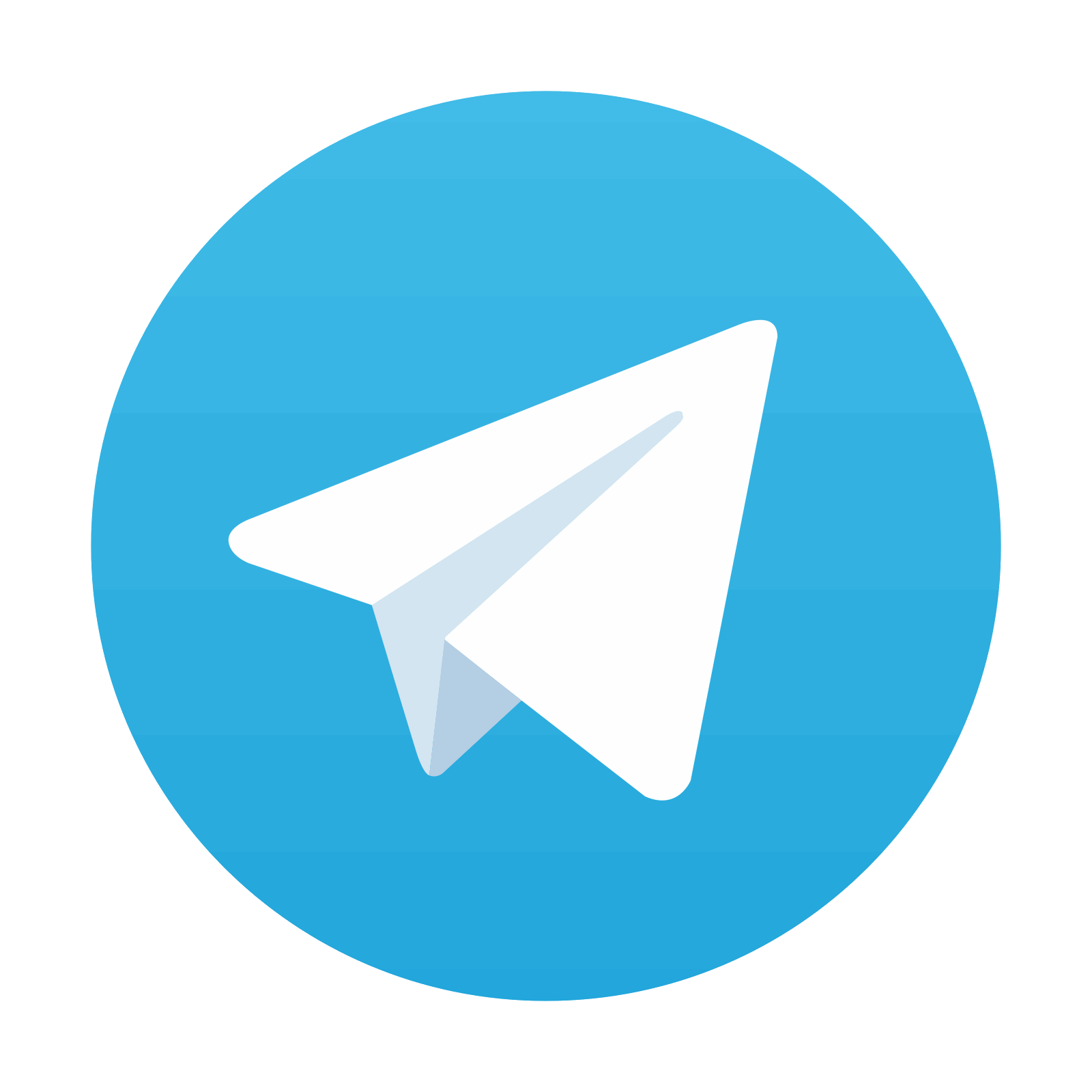
Stay updated, free articles. Join our Telegram channel

Full access? Get Clinical Tree
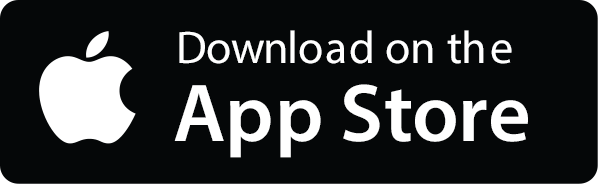
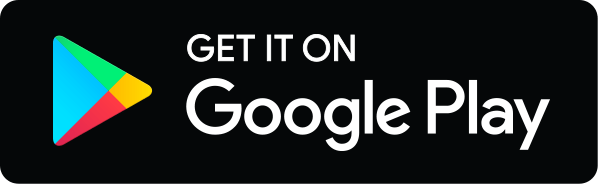