Profile
Common precipitants
Signs and symptoms
Hemodynamics on presentation
Profoundly hypertensive
Abrupt rise in BP
Rapid onset of dyspnea (“flash pulmonary edema”); systemic edema may be absent; diaphoresis with adequate perfusion typical
Systolic BP > 160 mmHg; sinus tachycardia and hypoxia common
Normal to moderately hypertensive
Progressive fluid accumulation
Gradual or subacute worsening of dyspnea; moderate to severe systemic edema; minimal distress with adequate perfusion
Systolic BP > 100 mmHg but <160 mmHg; tachycardia and hypoxia uncommon
Hypotensive
Deterioration of advanced, chronic disease; excessive diuresis
Mild dyspnea; often with cool, edematous extremities
Systolic BP < 90 mmHg; HR often normal but may be <60 beats per min if on baseline medications with rate control effects
Cardiogenic shock
Myocardial injury, valve dysfunction
Rapid onset of dyspnea with evidence of profound hypoperfusion
Systolic BP < 90 mmHg; tachycardia common (unless on rate control agents)—may be ventricular in origin
Arrhythmogenic
Ventricular or supraventricular dysrhythmia
“Palpitations” and “dizziness”; mild to moderate dyspnea (often secondary feature); systemic edema may be present or absent
Systolic BP variable; HR < 60 or >120 beats per min; hypoxia
Acute coronary syndrome
Acute myocardial ischemia or infarct
Chest pain with dyspnea
Systolic BP > 100 mmHg; HR variable; hypoxia less common
Isolated right heart failure
Right ventricular ischemia or infarct (right coronary or left circumflex); pulmonary hypertension; tricuspid or pulmonary valve dysfunction; pulmonary artery obstruction (embolism)
Dyspnea without rales; systemic edema if subacute or long standing
BP variable; tachycardia and hypoxia often present
Specific Targets of Therapy
The importance of appropriate ED treatment of acute HF cannot be sufficiently underscored. Data from Acute Decompensated Heart Failure National Registry (ADHERE) show that when intravenous (IV) vasoactive medications are started early by ED physicians rather than waiting for the inpatient service, outcomes such as mortality rate (4.3 % vs. 10.9 %), intensive care unit admission rate (4 % vs. 20 %), and total hospital length of stay (3 days vs. 7 days) are dramatically improved [17]. Therefore, knowing which agents to administer and the correct circumstance in which to administer them is critical. An overview of therapeutic targets within the context of clinical profiles. These targets are discussed in greater detail in the following sections with increased emphasis placed on those that are particularly relevant to management of the short-stay HF patient. While each is presented in isolation, there may be some overlap of targets in an individual patient.
Acute Hypertension (Afterload)
As noted, elevated BP (systolic BP > 140 mmHg) is present in more than half of all patients with Acute Heart Failure Syndrome (AHFS), and for those with substantial dyspnea, appropriate, early vasodilatation can lead to substantial improvement in symptoms [18]. A number of agents can produce afterload reduction, yet only a handful have been rigorously tested in the management of AHFS, and head-to-head comparison trials are sorely lacking. Regardless, it has been postulated that in patients with hypertensive AHF, the decision to implement therapy focused primarily on BP control (rather than volume reduction) may be more important than actual agent used [12]. Though such a hypothesis has not been tested in clinical trials, as shown in Studying the Treatment of Acute Hypertension (STAT), the degree of BP reduction has critical bearing on outcomes, with an increase in adverse event rates when the systolic BP declines to less than 120 mmHg [19]. Thus, when managing acute hypertension with any agent, close monitoring and frequent BP measurement is essential.
Nitrovasodilators
Nitrates have long been considered the first-line agents for AHF associated with elevated blood pressure. With varying strengths of recommendation, guidelines endorse the use of nitrate therapy in AHF. The use of nitrates is recommended by the Canadian Cardiovascular Society, the European Society of Cardiology (class IIa), and the American Heart Association (class IIb) [1, 20, 21].
As a class, nitrates work by providing an exogenous source of nitric oxide that is then available to bind to soluble guanylate cyclase, thereby producing vascular smooth muscle relaxation [22]. Combined effects on venous capacitance and arterial resistance lead to a decrease in pulmonary capillary wedge pressure (PCWP) [23, 24]. At higher doses (i.e., ≥ 150–250 mcg/min), arteriolar dilation predominates, helping to improve cardiac output through a reduction in afterload [25–27]. This effect may be more pronounced when systemic vascular resistance is severely elevated [28] and may be mediated through a dose-dependent, differential effect on the augmentation index—a measure of the amplified pressure wave that is reflected back to the central circulation from the periphery (i.e., a ratio of central/peripheral pulse pressure) during each cardiac cycle [29]. Nitrate tolerance is a common but poorly understood phenomenon thought to involve O2 free radical formation and nitric oxide (NO) synthase inhibition which can decrease the hemodynamic response to ongoing administration despite up-titration [30, 31].
Nitroglycerin (glyceryl trinitrate) is the most common nitrate used in the United States and is typically given as an initial sublingual tablet or spray (400 mcg/dose) to enable quick absorption and rapid onset of action. For persistent symptoms, transdermal application (1–2 in. of 2 % ointment) or, for more severe cases, IV administration may be required. Because the half-life of nitroglycerin (NTG) is short (<5 min), a continuous infusion (rate, 20–400 mcg/min) may be needed to maintain the effect. Higher doses of IV NTG (or its relative, isosorbide dinitrate [ISDN]) may be particularly useful in patients with profound BP elevations and respiratory distress (i.e., hypertensive cardiogenic pulmonary edema). Intravenous nitrovasodilators, when used in the treatment of AHF in the ED, have been shown to be effective in improving blood pressure and dyspnea in the short term [32]. Repeat IV bolus (every 3–5 min) of both high-dose NTG (2 mg) [33] and ISDN (4 mg) [34, 35] has been associated with a reduction in the need for mechanical ventilation and intensive care unit admission, a lower incidence of cardiac injury (as evidenced by biomarkers), and a shorter total hospital length of stay. In studies to date, substantial doses of NTG (mean [SD] = 6.50 [±3.47 mg]) and ISDN (mean [SD] = 11.4 mg [±6.8 mg]) have been given with a low incidence of hypotension (<4 %) and no report of adverse neurologic, renal, or cardiac events.
While sustained administration of such aggressive therapy may not be appropriate for the OU, most patients who respond do so quickly, often circumventing the need for continued IV nitrate therapy. Results of a topical high-dose nitrate strategy (two sublingual NTG tablets followed by application of ten NitroDerm TTS patches) have been reported with demonstration of a reduced intensive care unit admission rate and greater improvement in cardiac stress in the high-dose nitrate arm [36]. Importantly, this strategy was implemented in a nonmonitored setting (general medical ward), thus enabling possible extrapolation to the OU. Preload-dependent conditions, such as right heart ischemia, pericardial effusion/pericarditis, or restrictive cardiomyopathy, should be considered a contraindication to nitrate therapy (regardless of dose).
Even though the use of nitrates has been shown to be safe and effective in the stabilization phase of AHF, a recent large retrospective cohort study of over 11,000 patients did not show an improvement in short-term or near-term survival. In comparing patient groups receiving nitrates to non-nitrate groups, Edwin et al. found mortality rates of 1.8 % vs. 1.5 % (p = 0.151) at 7 days and 10.55 % vs. 10.1 % at 30 days (p = 0.540) [1].
Sodium nitroprusside (NTP) is another nitric oxide donor that can be used in profoundly hypertensive and dyspneic patients. The administration of NTP results in both preload and afterload reductions even at lower doses and has been shown to be effective for patients with refractory elevations in systemic vascular resistance [37]. However, controlled trials of NTP in AHF are lacking. Because of potential significant and prolonged hypotension as well as reflex tachycardia, invasive arterial monitoring and close supervision are recommended [38]. Furthermore, NTP may increase the risk of coronary steal syndrome and cyanide toxicity. Suffice to say, if used to treat AHF, NTP should be considered a contraindication to short-stay management in an OU setting.
Natriuretic Peptides
Since its approval by the Food and Drug Administration in 2001, nesiritide, a recombinant form of brain natriuretic peptide (BNP), has been widely studied as an alternative to existing vasodilator therapy with early trials suggesting benefit with its use [39–42]. However, Acute Study of Clinical Effectiveness of Nesiritide in Decompensated Heart Failure (ASCEND-HF), a large (n = 7141) definitive trial of nesiritide, was completed in 2010 finding a statistically significant but clinically irrelevant difference in dyspnea with the use of nesiritide, without any benefit on hard end points such as mortality and readmission. Additionally, an increased risk of asymptomatic hypotension with the use of nesiritide was seen (21.4 % vs. placebo 12.4 %) [43], though no other signals of potential harm were noted. Offering no specific advantage over existing therapy, nesiritide has fallen out of favor and is rarely used in the management of AHF today.
Other natriuretic peptide compounds, including ularitide (a synthetic analog of urodilatin, an atrial-NP derivative) and cenderitide (a chimer of c-type and d-type NP) [44], have been developed and are currently subject to preliminary investigation. Phase I and II studies of ularitide have shown favorable hemodynamic effects in reducing dyspnea without inducing renal dysfunction in AHF patients [45]. Ularitide is currently being tested in the phase III TRUE-AHF clinical study [46]. Cenderitide is being evaluated as a continuous subcutaneous infusion for use after the stabilization and in-hospital phase for systolic blood pressure reduction [45]. Further research is needed to determine what role, if any, these drugs will have in the ER and OU management of AHF.
Angiotensin-Converting Enzyme Inhibitors
Angiotensin-converting enzyme (ACE) inhibitors have been used in the setting of acute HF with hypertension. As a class, they are effective antihypertensives and provide antagonism of the renin–angiotensin–aldosterone system, making them ideal agents for HF treatment. Abundant data show substantial benefit from the use of oral ACE inhibitors in chronic HF (i.e., disease regression, symptom improvement, decreased mortality) [47–49]. Based on this, the American College of Cardiology/American Heart Association guidelines recommend ACE inhibitor therapy, absent contraindications, for all patients with symptomatic left ventricular dysfunction (< 40 % ejection fraction) [21]. Data abounds showing substantial benefits in disease regression, symptom improvement, and decreased mortality [50]. The timing of initiation of oral ACE inhibitor therapy has been recommended to be within the first 24–48 h after hemodynamic stabilization. There is limited data on the safety of ACE inhibitors in the early phase of therapy (first 12–24 h) [32, 51]. Major concerns include sustained hypotension due to their relatively longer half-lives, renal dysfunction, and hyperkalemia. Therefore, absent further safety data, ACE inhibitors should be used with caution during the stabilization phase of AHF care.
Calcium Channel Blockers
Due to their negative inotropic effects, beta-blocking agents and nondihydropyridine calcium channel blockers (CCBs) are considered contraindicated in the initial management of AHF [52]. It has also been recommended to avoid rapid-onset dihydropyridine CCBs, such as sublingual nifedipine, as they produce unpredictable effects on peripheral resistance and have been correlated with an increased risk of coronary and cerebral hypoperfusion [50, 53]. However, fourth-generation short-acting IV dihydropyridine CCBs (i.e., nicardipine and clevidipine) have shown promise [54]. As shown in the PRONTO trial, clevidipine works to rapidly and safely reduce blood pressure and dyspnea in patients with hypertensive AHF. Compared with standard of care (n = 53), more patients in the clevidipine group (n = 51) reached target blood pressure range within 30 min (71 % vs. 37 %, p = 0.002); dyspnea improvement within 45 min (−37 vs. 28 on a visual analog scale, p = 0.02) was also greater in the clevidipine group [55] suggesting a potential future role for this class of agents in AHF.
Investigational Therapy
Relaxin is a peptide hormone released in pregnancy that helps regulate hemodynamic function and renovascular blood flow. Specific effects of relaxin include production of nitric oxide, vascular endothelial growth factor, and matrix metalloproteinases as well as inhibition of endothelin and angiotensin II. Such effects result in a number of vascular changes (especially systemic and renal vasodilation) that may be beneficial in acute hypertensive HF [56].
The RELAX-AHF trial, a multicenter international randomized control trial of 1161 patients with AHF, was published in 2013 and suggests the drug serelaxin improved dyspnea in the visual analog scale through day five and reduced average length of stay. However, the proportion of patients with significant improvement in dyspnea measured by the Likert scale during the first 24 h was not improved. While 60-day readmission was also not significantly improved, all-cause mortality and cardiovascular death at 180 days were significantly decreased [57, 58]. Based on the latter, a large-scale trial powered for mortality and worsening in-hospital HF has been initiated, results of which will determine the future role of serelaxin in the management of AHF.
Other drugs including TRV-027, a novel beta-arrestin biased ligand of the angiotensin II type 1 receptor, are currently being studied for use in AHF as well.
Excess Volume (Preload)
Volume overload is another common feature in patients presenting with AHF, and the relief of congestion through removal of excess fluid is an important goal of therapy [59]. The 2013 American College of Cardiology Foundation/American Heart Association Heart Failure Guidelines recommends diuretic therapy without delay in the emergency department in patients with significant fluid overload [21]. Despite a lack of prospective, randomized trials, diuretics have remained the mainstay of therapy for decades and are used in the vast majority (∼90 %) of patients with acute HF symptoms. Several alternatives have been recently investigated, but none has been found to be superior in terms of safety or efficacy. Consequently, diuretics remain the de facto “standard” of care for AHF in those with (and often without) hypervolemia.
Loop Diuretics
Intravenous loop diuretics (furosemide, bumetanide, torsemide, and ethacrynic acid) work by inhibiting the Na+–K+–2Cl− cotransport channel in epithelial cells which line the thick ascending limb of the loop of Henle [60] and are the most common class of medication used in the treatment of AHFS. They work to produce an osmotic diuresis and have an onset of action of approximately 30 min postadministration with a peak effect at 2–4 h. Furosemide is the agent used most frequently in the United States, and with typical dosing (40–80 mg IV every 8–12 h), in-hospital fluid losses can approach 4 L [61]. Furosemide has been noted to have hemodynamic effects as well, with nonsustained vasodilation occurring 5–15 min after administration [62]. Latent vasoconstriction has also been reported and appears to be related, at least in part, to activation of neurohormonal factors [63]. Despite this potential disadvantage, loop diuretics do effectively reduce filling pressures and induce symptomatic improvement [64], making them widely accepted for acute HF treatment.
The optimal approach to diuretic dosing and administration has been a source of ongoing controversy. Higher cumulative doses of furosemide have been associated with an increased risk of in-hospital death in one study [11], and in a Cochrane Collaborative review, continuous infusion was found to be more effective than repeat bolusing, particularly for those patients with refractory edema or congestion [65]. The best evidence to date, however, has come from the recently completed diuretic optimization strategies evaluation (DOSE) study, which prospectively compared approaches to IV furosemide administration [66, 67]. Using a 2 × 2 factorial design, patients (n = 308) were randomized to receive high (2.5 times daily oral) vs. low (daily oral) dosing and intermittent bolus (every 12 h) vs. continuous infusion for a period of at least 48 h. No statistical difference in global symptom relief or absolute change in renal function at 72 h was found for either level of comparison (i.e., low vs. high dose and intermittent bolus vs. continuous infusion) [67]. However, there was a signal of greater improvement with use of a high-dose strategy in several secondary end points including dyspnea relief, weight loss and net volume loss, proportion free from signs of congestion, and reduction in biomarkers of myocardial stress (i.e., NT-proBNP) suggesting some clinical benefit with a high-dose diuretic approach.
Some patients are found to be less responsive to even high-dose loop diuretics, and they have shown to have poorer outcomes and increased mortality [68]. Though such resistance is more common in those on long-term therapy, on occasion, it may be seen in diuretic-naïve patients with profound volume depletion and decreased renal perfusion. In such patients, an enhanced effect may be achieved through combining loop and thiazide diuretics [69, 70]. Adding a thiazide leads to what has been called “sequential nephron blockade” based on the portion of the nephron where the two classes of drugs exert their effect. In patients undergoing continuous furosemide infusion and intact renal function (GFR > 75 mL/min), adding a thiazide potentiates the diuretic effect [7] but requires close monitoring for hypokalemia as both loop and thiazide diuretics are known to decrease hemoconcentration of potassium. The ongoing Combination of Loop with Thiazide-type Diuretics in Patients with Decompensated Heart Failure (CLOTRIC) is the first large-scale trial to evaluate the safety and efficacy of adding thiazide to loop diuretic therapy [71].
Vasopressin Antagonists (Vaptans)
Arginine vasopressin, also known as antidiuretic hormone, triggers manufacture and cell membrane insertion of aquaporin-2 molecules in renal collecting ducts and thus serves as a potent stimulus for free water reuptake by the kidneys. Vasopressin release is upregulated in HF, and its appearance contributes greatly to dysregulated fluid accumulation. While V1 receptors primarily regulate the effect of vasopressin in the vasculature (where it produces vasoconstriction), V2 receptors function in the kidney. Antagonists of vasopressin (conivaptan [a dual V1/V2 receptor antagonist], tolvaptan [a V2 ≫ V1 receptor antagonist], and lixivaptan [a V2 ≫ V1 receptor antagonist]) block this pathway, resulting in increased excretion of low-solute fluid, enabling reversal of hyponatremia (a known risk factor in acute HF) without adversely affecting glomerular filtration rate or renal blood flow [72]. This class of medications, therefore, has broad theoretical appeal for use in AHFS, offering a pharmacological approach to volume reduction that lacks the drawbacks of loop diuretics. Despite such potential, utility of the “vaptans” in Efficacy of Vasopressin Antagonism in Heart Failure Outcome Study With Tolvaptan (EVEREST), a two-part investigation that enrolled over 4,000 patients with acute HF, was less than ideal offering a statistically significant (though clinically marginal) improvement in dyspnea, edema, serum sodium, and renal function without any long-term effect on mortality or HF-related morbidity [73, 74]. More recent studies have found tolvaptan use to be an independent and powerful predictor of improving sodium levels in hyponatremic AHF, an independent predictor of survival, but did not show improvement in outcome [75]. Consequently, there is no indication for use of vasopressin antagonism in AHF at present.
Ultrafiltration
Mechanical fluid removal using ultrafiltration is an alternative to pharmacological diuresis and a viable option for the management of AHF with volume overload, particularly in those with diuretic resistance or the cardiorenal syndrome. Ultrafiltration is an efficient yet costly (∼$19,500 for device acquisition and $950 per filter, with 1–2 filters required per treatment) mechanism which uses venovenous hemoconcentration to extract up to 500 mL of isotonic fluid per hour. While early trials such as Ultrafiltration Versus Intravenous Diuretics for Patients Hospitalized for Acute Decompensated Heart Failure (UNLOAD) suggested a reduction in readmissions with the use of ultrafiltration [76], the recently completed Cardiorenal Rescue Study in Acute Decompensated Heart Failure (CARRESS-HF) found no beneficial effects on fluid removal or renal function and no difference in mortality or rehospitalization through 60 days with ultrafiltration compared to a stepped diuretic approach [77]. As a result, the role of ultrafiltration is limited in the management of AHF.
Diminished Cardiac Function
Cardiac output can be acutely reduced for a number of reasons, but ischemia, valvular dysfunction, and arrhythmia are among the most common. Each of these has inherent therapy that warrants a discussion that is beyond the scope of this chapter. It is important to remember, however, that when treating HF related to such causes, the primary intervention should be directed toward the inciting factor (i.e., reperfusion for ischemia, surgery for critical valve dysfunction, or rate control for atrial fibrillation) rather than the end manifestation.
On occasion (<5 % of the time), reduced cardiac output with hypoperfusion will result from a simple, subacute progression of underlying, advanced HF, and intervention to improve pump function (i.e., inotropes) may be needed. In general, such patients are poor candidates for short-stay management of AHF, but some, especially those with end-stage disease, may benefit from a brief “tune-up” with medications that augment cardiac function. For those without evidence of pulmonary congestion, a small bolus of isotonic normal saline (250–500 cc) may be attempted first, as these individuals frequently suffer from intravascular depletion as a result of chronic overdiuresis. It is important to remember that while inotropic agents can effectively transiently improve cardiac function, they should be used cautiously, especially in patients with coronary artery disease, as they increase myocardial oxygen demand and enhance the potential for arrhythmia development [38, 78–80].
The most commonly used inotropes are norepinephrine, dobutamine, and milrinone. Norepinephrine is an alpha- and beta-adrenergic agonist that combines cardiac chronotropic and ionotropic response (and, consequently, cardiac output) with peripheral vasoconstriction [81]. Dobutamine acts through β1– and β2-adrenergic receptor stimulation to increase inotropy and chronotropy [82]. Vascular effects include vasodilatation at low doses and vasoconstriction at higher doses. Patients with a history of beta-blocker usage at baseline may require increased dosing to achieve therapeutic effect [83]. Milrinone is a type III phosphodiesterase inhibitor (PDEI) which also improves hemodynamic function (i.e., stroke volume and cardiac output) but does so by preventing intracellular breakdown of cyclic adenosine monophosphate (cAMP) [84]. Though this activity is independent of adrenergic receptor stimulation, it produces similar net effects on the heart (i.e., inotropy, chronotropy, and lusitropy) [85]. In the peripheral circulation, however, vasodilatory effects predominate resulting in significant preload and afterload reduction. This latter response may cause a worsening of hypotension, particularly in patients with intravascular volume depletion [86]. Concurrent administration of dobutamine and milrinone (or an alternative PDEI such as amrinone or enoximone) yields an additive effect on cardiac function and may be a useful approach for those on chronic beta-blocker therapy [87, 88].
Positive inotropic effects can also be accomplished by targeting the myocardial contractile apparatus itself. Traditionally, this has been achieved through the use of cardiac glycosides (i.e., digoxin) which produce their desired effect by inhibition of Na+–K+ ATPase. Mediated through an increase in intracellular sodium, this works to establish a gradient that promotes intracellular calcium ion accumulation, which subsequently enhances myocyte contractility, resulting in an incremental improvement in cardiac output. Digoxin was commonly used for management of AHF two to three decades ago but has since fallen out of favor [89]. Digoxin, however, is one of the few medications which, when used in the ambulatory setting, has actually been shown to reduce rehospitalization for HF [90], and there is resurgent interest potential utility for patients with acute symptoms [91].
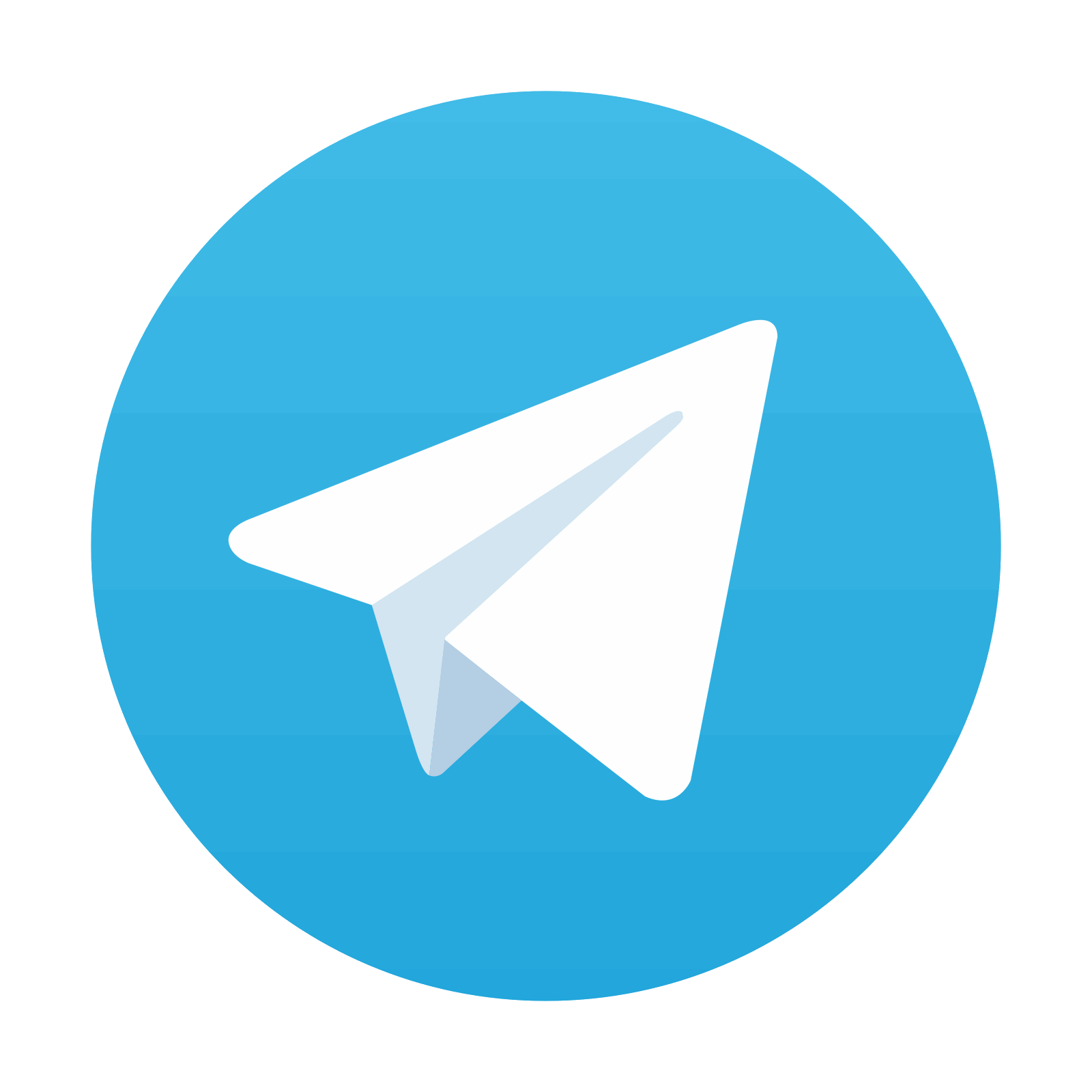
Stay updated, free articles. Join our Telegram channel

Full access? Get Clinical Tree
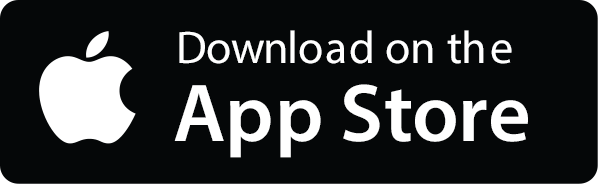
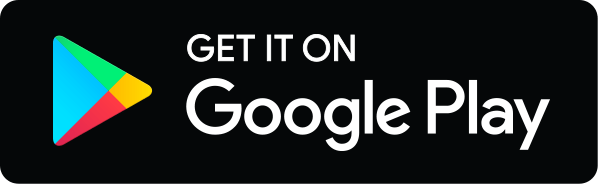