Embolization and coronary no-reflow are rare but important complications during percutaneous coronary intervention (PCI). Recognition and treatment are important in that they may reduce or prevent myocardial necrosis and serious subsequent complications. Additionally, nonclinical events may occur as a result of this phenomenon, and prevention where possible becomes important. Macroembolization usually is due to macro-particles and/or blood clots that may occur during intervention of patients with acute coronary syndromes. No-reflow may occur due to the embolization of micro-particulate matter as debris from coronary lesions, activation of platelets, and/or release of vasoactive substances that may modulate distal vasculature. No-reflow is defined as the reduction of coronary flow in the absence of a proximal occlusive lesion with resultant myocardial ischemia. In PCI of native coronary vessels in absence of acute coronary syndromes, no-reflow may be due to microvascular spasm and/or platelet microemboli. When PCI is performed in thrombotic lesions, such as in acute coronary syndromes, no-reflow may be due to distal embolization of thrombus. During saphenous vein graft intervention and rotational atherectomy, it is most often related to embolization of degenerated plaque elements including thrombotic and atherosclerotic debris. Any or all of these mechanisms can occur simultaneously.
A number of strategies have been available as adjuncts to reduce embolization, but many have been shown to be ineffective and are no longer available. Many are mentioned here for an historical perspective.
The problem of embolization of plaque material during percutaneous catheter angioplasty and stenting has been recognized since the early development of balloon angioplasty. Shortly after the introduction of balloon angioplasty in an experimental model of angioplasty, effluent debris was collected and analyzed by histologic and polarized light microscopic examinations for gross debris and cholesterol.1 This study is often widely cited as showing no evidence for significant embolization during balloon angioplasty. Microscopically visible atherosclerotic debris was present in 12% of procedures, and thin-layer chromatography showed evidence of cholesterol embolization in 25%. These findings of atherosclerotic debris in between 12% and 25% of treated vessels is completely in line with clinical studies that followed in patients over the next 2 decades.
Both particle size and amount of the embolic material are important. Small amounts of 15-μm particles have been shown to cause patchy ischemia, while increased numbers of particles can cause decrease in flow. When particles are larger, in the 100 to 300 μm range, a relatively few particles can reduce flow.
Clinical studies have found evidence for embolization associated with PCI procedures. In the stent era, embolization has been detected in about 20% of simple native vessel stent implantation procedures based on the occurrence of creatine phosphokinase-MB (CPK-MB) enzyme release only. For example, in the STARS trial, patients with type A lesions who had completely uncomplicated, successful stenting with a single stent were evaluated. In this group, the incidence of CPK-MB release was 20%.2 In the STARS registry, which included patients who had dissections, had suboptimal stent results, or required additional stents, the incidence of CPK-MB release was close to 30%.3 Similarly, in the STRATAS rotational atherectomy trial, almost 30% of patients had CPK-MB release.4 The majority of these enzyme elevations are not associated with clinical events.
Clinically apparent embolization occurs more frequently in saphenous vein graft (SVG) interventions. In-hospital major events occur in almost 15% of these interventions.5,6 In contrast to the enzyme elevations seen with PCI in native vessels, these are clinical events including chest pain, electrocardiographic changes, and/or marker elevation. Thus, suspected embolization occurs in 15% to 20% of PCI cases overall.
One of the more severe manifestations of embolization is the no-reflow phenomenon. This was originally identified in experimental models of myocardial infarction.7 It is the failure of restoration of myocardial flow despite removal of the epicardial coronary obstruction. This is an infrequent event during PCI, complicating between 0.5% and 3% of procedures. It is most typically associated with reperfusion after acute coronary syndromes, particularly acute ST-segment elevation myocardial infarctions and in SVG interventions. It also occurs as a result of rotational atherectomy. It can also occur, more rarely, in native vessels.
The clinical sequelae of the no-reflow phenomenon can be both acutely and chronically severe. Patients have, in the most profound situations, hemodynamic collapse or a severe ischemic syndrome, depending on the size of the affected vessel territory. Both prevention and treatment have been challenging during the development of catheter intervention.8
The short-term and long-term consequences of particulate embolization during PCI have been studied extensively. Acute complications include the no-reflow phenomenon, which is slow or no flow in treated vessels, causing prolonged chest pain, and can produce myocardial cell necrosis.
In the Primary Angioplasty and Myocardial Infarction (PAMI) trial, no-reflow occurred during primary coronary intervention for ST-segment elevation acute infarction in 1.3% of over 1000 patients. Compared with patients who did not have no-reflow during PCI, those with the no-reflow phenomenon had a significantly higher hospital and 6-month mortality. Hospital mortality was 2% without versus 13% with no-reflow (P = .04), and at 6 months, it was 3% without versus 31% with no-reflow (P = .0001); this illustrates the striking clinical importance of this phenomenon.9 In another study, data were prospectively collected from over 4200 consecutive patients undergoing PCI to identify those with no-reflow. No-reflow was identified in 3.2% of patients. Patients with no-reflow were more likely to have acute myocardial infarction, unstable angina, and cardiogenic shock or have undergone SVG intervention. No-reflow in this group was also highly predictive of postprocedural myocardial infarction (17.7% vs 3.5% in patients without no-reflow). No-reflow was similarly a strong predictor of in-hospital mortality. Administration of calcium-blocking drugs or nitroprusside was not associated with improved in-hospital outcomes in these patients, although antegrade flow rates improved in patients treated with nitroprusside in this group. Mortality was 7.4% with versus 2% without no-reflow (P <.001; odds ratio [OR], 3.6).10 Late consequences of these events have been characterized in numerous studies, suggesting a relationship between in-hospital CPK elevations, reflective of embolization, and increased late mortality as long as 3 years after the index interventional procedure.11
A variety of mechanisms for the clinical effects of embolization during PCI have been elucidated (Table 62-1). Most simply, mechanical disruption of the plaque results in fragmentation of plaque elements.12 A variety of plaque elements can be identified, including plaque fragments, cholesterol crystals, foam cells, and amorphous debris.13
|
In a study of the aspirated particles from 23 SVG procedures retrieved using an extraction system, a mean particle size of 168 μm was noted. The range was large, from a minimal of 6 to a maximum of 815 μm in the minor access, and 7 to 3427 μm in the major access of these particles. Particles were composed of cholesterol clefts, lipid-rich macrophages, fragments of fibrous cap, necrotic lesion core, and fibrin.
Many of the causes of embolization of microscopic debris into the small vessels of the distal microcirculation are well described. This is evidenced by studies of rotational ablation.14,15 Debris of varying sizes of particulate has variable effects on microcirculatory plugging. Rotational atherectomy creates particulate debris with a mean particle size of less than 6 μm. These smaller particles pass through the capillary circulation in the same manner as red blood cells. Larger particulate, which comprises about 20% of the rotational atherectomy debris, can be trapped in the microcirculation and contributes to slow flow and CPK elevations.
Hori et al16 studied the effective particle size and number in a coronary arterial microsphere embolization perfused canine model. Small numbers of 15-μm particle emboli caused patchy myocardial ischemia and a paradoxical regional increase in blood flow from the effect of reactive increased flow to nonaffected adjacent areas. Increasing numbers of emboli caused impaired flow reserve and decreased resting flow. Larger particles (100-300 μm) caused a dramatic reduction in the number of emboli needed to cause decreased resting flow.
Angiographic thrombus is a powerful predictor of PCI complications. In the clinical setting of acute coronary syndromes, macroembolization of clot is well known. Gross distal embolization can be observed even when no thrombus is identified. Many interventionalists have had the experience of watching a fragment of clot, or thrombus, visibly embolize downstream during injections in the middle of PCI procedures.
Liberation of vasoactive humoral substances such as serotonin has also been implicated in the genesis of slow flow and no-reflow. Serotonin levels have been measured in the distal coronary artery in patients after angioplasty or stenting.17 Balloon angioplasty caused an increase in coronary sinus serotonin from basal values of 3 ng/mL to almost 30 ng/mL after balloon angioplasty and from 3.5 ng/mL to 114 ng/mL after stenting. This increase in liberation of serotonin was dramatically blunted by the administration of the serotonin antagonist ketanserin. Coronary cross-sectional area distal to the site of dilatation significantly decreased after angioplasty and stenting from 4.33 to 3.3 mm2 after balloon angioplasty, and from 4.27 down to 2.86 mm2 after stenting, consistent with distal vessel modulation. Pretreatment with ketanserin diminished this decrease in distal coronary luminal area.
One candidate protein that may cause coronary no-reflow is tissue factor. This is abundant in atherosclerotic plaque. In 1 study, coronary blood was drawn from 6 patients undergoing coronary intervention with a distal protection device, and the particulate material that was recovered showed tissue factor activity. To examine the role of tissue factor in no-reflow, blood was drawn via a catheter from coronary vessels in 13 patients during no-reflow and after restoration of flow. Mean tissue factor antigen levels were elevated during no-reflow compared with levels after flow restoration (194 vs 73 pg/mL, P = .02). Tissue factor induced no-reflow in a porcine model after selective intracoronary injection of atherosclerotic material or purified tissue factor. These data suggest that tissue factor is released from dissected coronary plaque and is 1 of the factors causing the no-reflow phenomenon.18
Indirect evidence for the role of vasoactive mediators is seen in the attenuation of rotational ablation flow effects by the use of a vasoactive “cocktail.”19
The problem of embolization during PCI became particularly apparent during SVG intervention.20 Both the volume of SVG atheroma and its friable nature make it prone to embolization during intervention (Fig. 62-1).
As many as half of patients with saphenous vein bypass graft (SVBG) have angiographic evidence of atherosclerosis 5 years after surgery. A large proportion of grafts are severely diseased by 7 to 10 years after surgery. In most PCI trials, the mean age for SVGs undergoing PCI is between 8 and 9 years after the original bypass surgery. In addition to the problems presented by the nature of the graft atheroma itself, patients are obviously a decade older than when their initial bypass surgery was done and represent a group with high frequency of congestive heart failure, prior myocardial infarction, depressed left ventricular function, and other comorbid conditions.
During PCI for SVGs, distal embolization is common. Patients who suffer distal embolization during these procedures have significantly poorer outcomes than patients who do not. The proportion of patients with major adverse in-hospital events after embolization approaches three-quarters, whereas patients without clinical evidence of embolization during their intervention have major adverse event rates in the hospital of less than 20%.21
Early attempts to improve the outcome of intervention in SVGs included the use of plaque removal or debulking using directional coronary atherectomy (DCA). In the CAVEAT-2 study (Coronary Angioplasty Versus Excision Atherectomy Trial), patients were randomized to receive DCA versus plain balloon angioplasty (percutaneous transluminal coronary angioplasty [PTCA]). Although angiographic success rate was improved with DCA (from 79% with PTCA to 89.2% with DCA) and 6-month restenosis rates were similar (45.6% for DCA and 50.5% for PTCA), distal embolization occurred more frequently with DCA than PTCA (13.4% vs 5.1%; P = .012). The risk of thrombus embolization was much higher when visible thrombus was recognized (39% vs 14%). The 1-year outcomes were worse in patients who developed distal embolization. Figure 62-2 shows a simple thrombus aspiration catheter.
Figure 62-2
A. Simple thrombus aspiration catheter. A syringe is attached to the lumen for aspiration. The lumen has the capacity to remove a significant amount of thrombus. B. Schematic illustrating the aspiration of thrombus into the catheter lumen. The system is compatible with a 6-Fr guide catheter. See the “Aspiration Thrombectomy” section of the chapter for more information. (Image used with permission from Vascular Solutions, Inc./Teleflex.)

Other devices, such the transluminal extraction catheter (TEC), were developed for plaque and thrombus removal.22,23 No-reflow was seen in 19% of patients with thrombus and 5% of patients without thrombus. Late complete vessel occlusion was seen in over one-third of patients with thrombus. The excimer laser has also been evaluated as a method of plaque removal. In an initial series of 495 patients, embolization occurred in only 3.3% of patients and Q-wave infarction in only 2.4% of patients. However, in some laser studies, thrombus-containing lesions were associated with increased rates of embolization and poor clinical outcomes.
Rheolytic thrombectomy, a technique that uses high-velocity saline jets directed retrograde into the open mouth of a Bernoulli device, draws thrombus into the catheter tip for maceration and evacuation. In the Vegas II trial, rheolytic thrombectomy in native coronary vessels and SVGs containing thrombus was compared to urokinase infusion. Over half the patients treated had vein graft as opposed to native vessel thrombus-containing lesions. Rheolytic thrombectomy resulted in a significant decrease in major events at 30 days. Little subsequent study has been conducted of the efficacy of this form of thrombectomy therapy for the treatment of degenerated SVG lesions that are predominantly atherosclerotic rather than thrombotic.24
Another atherectomy (X-Sizer; EV3, Plymouth, MN) device using a cutting device with suction has been evaluated. No difference in acute events or outcomes at 1 year were noted.25,26
A recent meta-analysis of over 9000 patients in 16 trials found that ablative devices failed to reduce complications associated with embolization. Periprocedural myocardial infarctions occurred in 4.4% of patients tested with devices versus 2.5% without (OR, 1.83; 95% confidence interval [CI], 1.43-2.34).27
The use of conventional bare metal stents had improved the results of catheter therapy for obstructive SVGs but did not demonstrate any reductions in restenosis rate. In addition, the potential for liberation of particulate degree with embolization seems increased by the use of stenting compared to conventional plain balloon angioplasty. One of the first concepts tested to diminish the potential for embolization using stenting was evaluation of membrane-covered metal stents compared with bare metal stents (Fig. 62-3). A polytetrafluoroethylene (PTFE) membrane–covered balloon-expandable stent was compared with conventional stents in a randomized trial.28 The concept that the PTFE membrane might trap graft atheroma behind the stent and prevent its liberation was assessed. Over 200 patients were randomly assigned to receive either a conventional metal stent or a membrane-covered stent. Neither restenosis nor acute procedural events were diminished by the use of a membrane-covered stent.
Figure 62-3
Angiographic frames from a saphenous vein graft percutaneous coronary intervention (PCI) in which a polytetrafluoroethylene (PTFE) covered stent was used. A. There is a proximal graft lesion, noted by the circle. The inset shows the covered stent being deployed in the lesion. High pressure is required to fully expand the combination of graft membrane and stent material. In this case, 18 atmospheres was necessary for adequate stent expansion. B. The silhouette of the fully expanded stent in the proximal vessel.
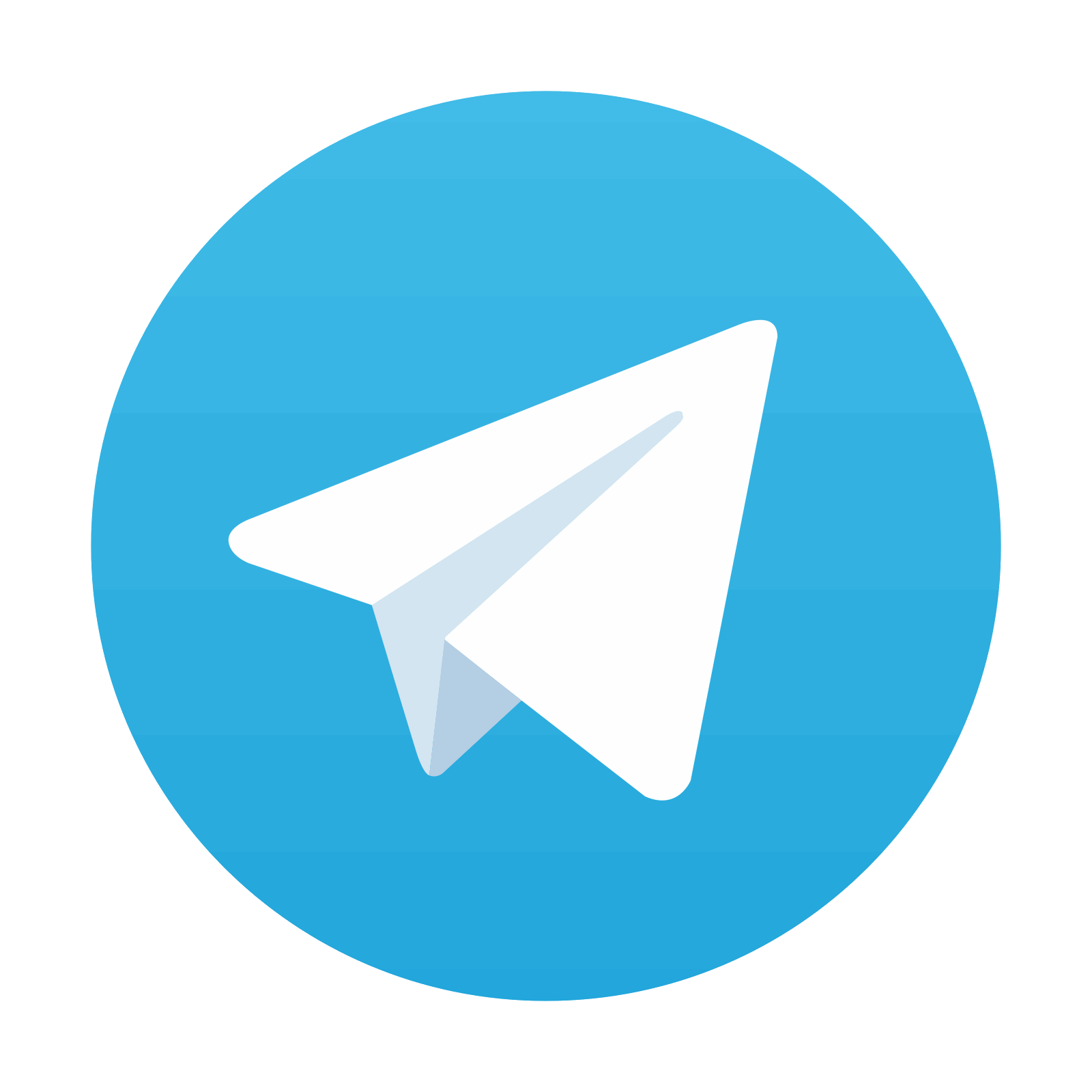
Stay updated, free articles. Join our Telegram channel

Full access? Get Clinical Tree
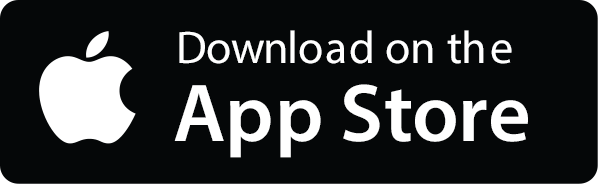
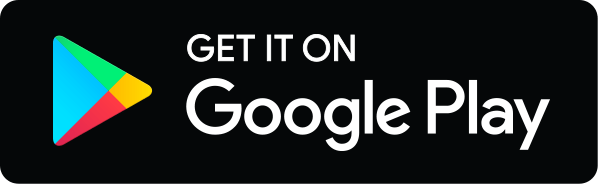
