As the initial recipient of the venous circulation, the pulmonary vascular bed is uniquely positioned to filter diverse particulates within the venous circulatory system. The etiology of these circulating particles may be thrombotic, as seen in pulmonary thromboembolism, or nonthrombotic, as encountered in a number of uncommon syndromes including embolism from air, amniotic fluid, fat, tumors, septic foci, and other miscellaneous sources. The pathophysiologic sequelae of these emboli are related to both mechanical occlusion of the pulmonary vasculature and inflammatory mediated damage to the pulmonary microvasculature resulting in capillary leak and pulmonary edema. The intent of this chapter is to describe the incidence, pathophysiology, and clinical features of these varied embolic phenomena and to provide a rational approach to the diagnosis and treatment of these disorders.
Pulmonary embolism (PE) is a commonly occurring condition associated with significant morbidity and mortality. Although there is a well-established and decreased incidence of PE with appropriate prophylaxis and a significant improvement in patient outcome associated with the prompt institution of appropriate therapy, prophylaxis and therapy for venous thromboembolic disease continue to be underused, thereby contributing to the morbidity, mortality, and high costs associated with the condition. In the United States, it is estimated that more than 500 000 cases of PE occur each year1 with a mortality rate of greater than 15% in the first 3 months after diagnosis.2 Despite our increased knowledge about the condition and ever-increasing technological sophistication, mortality rates associated with PE remain high and relatively unchanged over the past half century.3 Additionally, autopsy studies continue to demonstrate that the diagnosis of PE is made less than 50% of the time prior to death.4 While specific risk factors have been identified for the occurrence of PE and numerous effective prophylactic regimens have been demonstrated, recent studies show that appropriate prophylactic regimens continue to be significantly underused.5
PE results from the detachment and migration of thrombi fragments, which lodge within and obstruct blood flow to a single or multiple areas of the pulmonary vascular bed (Figure 45-1). Pulmonary emboli generally detach from deep venous thromboses (DVTs) of the proximal lower extremities.6 Less commonly, deep pelvic veins or proximal upper extremity DVTs associated with central venous catheters can be the source of pulmonary emboli.7 PE is, therefore, a part of the continuum of venothromboembolism (VTE). Thrombi, from which pulmonary emboli may arise, occur, in the majority of cases, when one or more components of Virchow’s classic triad is/are present: Venous stasis, hypercoagulability, and/or intimal injury.
A cascade of pathophysiologic mechanisms ensues with the pulmonary embolic event, which can lead to hypoxemia and cardiovascular compromise. The reduction of the cross-sectional area of the pulmonary arterial bed caused by the embolism has been shown to correlate with the degree of physiologic impairment. Pulmonary arterial pressure generally begins to rise with a 25% to 30% occlusion of the pulmonary vascular bed in otherwise normal heart and lungs,8 while greater than 50% obstruction is generally present before a clinically significant elevation occurs. Increased right ventricular afterload can cause right ventricular dilatation, hypokinesis, tricuspid regurgitation, and eventual right heart failure.9 A normal right ventricle can fail with acute increases of the pulmonary arterial pressure to 40 to 50 mm Hg when obstruction of the pulmonary vascular bed approaches 75%. Patients with PE and preexisting cardiopulmonary disease may experience more severe physiologic impairment with lesser degrees of pulmonary vascular obstruction.10 The increase in pulmonary vascular resistance, which occurs with PE, can impede cardiac right ventricular outflow and diminish left ventricular preload to cause an acute decrease in forward cardiac output and, ultimately, hemodynamic collapse. Hypoxic pulmonary vascular vasoconstriction and the release of neurohumeral factors incited by the embolic event are additional factors, contributing to increases in pulmonary vascular resistance and the resultant adverse hemodynamic consequences.11
Hypoxemia results from pulmonary emboli by a number of mechanisms. PE creates a redistribution of pulmonary blood flow to alter ventilation–perfusion characteristics in the lung, thereby decreasing the efficiency of alveolar gas exchange.12 Atelectasis may also occur and contribute to altered ventilation–perfusion relationships and gas exchange impairment.9 A right-to-left shunt may develop with PE by the intrapulmonary passage of blood through unventilated gas exchange units and/or intracardiac shunting of blood through a patent foramen ovale associated with increases in right atrial pressure occurring with PE.11 The inability to correct arterial hypoxemia with supplemental oxygen suggests the existence of intrapulmonary and/or intracardiac right-to-left shunting of blood. Additionally, decreased cardiac output and hypoxemia can lead to a low venous blood oxygen tension, which may amplify gas exchange impairment through lung gas exchange units with poor ventilation–perfusion characteristics.
While arterial hypoxemia and an increased alveolar–arterial oxygen gradient are the most common abnormalities of gas exchange, hyperventilation is common with PE and can cause hypocapnia and respiratory alkalosis. However, PE can increase both anatomic and physiologic dead space, thus impairing carbon dioxide removal. Hypercapnia associated with PE suggests underlying obstructive airway disease or a massive clot burden.13
One or more elements of Virchow’s triad (stasis, hypercoagulability, and intimal injury) are present in the vast majority of patients with VTE.14 General risk factors for VTE include age, immobility, surgical procedures, congestive heart failure, stroke and paralysis, trauma, malignancy, and hypercoagulable states. However, numerous clinical circumstances associated with an increased risk for the development of VTE have been identified (Table 45-1). Most patients with PE have more than one risk factor.14 Various degrees of risk have been defined for individual risk factors, with some patient populations having a greater than 50% incidence of VTE.15 Knowledge of these risk factors and a thorough patient history investigation for these factors are needed in order to develop an efficient and rational approach to the evaluation, diagnosis, and treatment of PE for the individual patient, particularly since presenting clinical signs and symptoms are insensitive and nonspecific for the diagnosis of PE.
Environmental |
Long-haul air travel |
Obesity |
Cigarette smoking |
Hypertension |
Immobility |
Natural |
Increasing age |
Women’s health |
Oral contraceptives, including progesterone-only and especially third-generation pills |
Pregnancy |
Hormone replacement therapy |
Medical illness |
Previous PE or DVT |
Cancer |
Congestive heart failure |
Chronic obstructive pulmonary disease |
Diabetes mellitus |
Inflammatory bowel disease |
Antipsychotic drug use |
Chronic indwelling central venous catheter |
Permanent pacemaker |
Internal cardiac defibrillator |
Stroke with limb paresis |
Nursing-home confinement or current or repeated hospital admission |
Varicose veins |
Surgical |
Trauma |
Orthopaedic surgery, especially total hip replacement, total knee replacement, hip fracture surgery, knee arthroscopy |
General surgery, especially for cancer |
Gynaecological and urological surgery, especially for cancer |
Neurosurgery, especially craniotomy for brain tumour |
Thrombophilia |
Factor V Leiden mutation |
Prothrombin gene mutation |
Hyperhomocysteinaemia (including mutation in methylenetetrahydrofolate reductase) |
Antiphospholipid antibody syndrome |
Deficiency of antithrombin III, protein C, or protein S |
High concentrations of factor VIII or XI |
Increased lipoprotein (a) |
Nonthrombotic |
Air |
Foreign particles (e.g., hair, talc, as a consequence of intravenous drug misuse) |
Amniotic fluid |
Bone fragments, bone marrow |
Fat |
Cement |
Symptoms and physical examination findings have been consistently shown to be insensitive and nonspecific for the diagnosis of VTE.16,17,18,19 Classic clinical findings of DVT include pain and swelling of the affected extremity, warmth and superficial venous dilatation, a thrombosed venous cord, and pain upon passive dorsiflexion of the foot (Homan’s sign). Patients with PE frequently present with dyspnea, tachypnea, tachycardia, and/or chest pain.20 Cough and hemoptysis may also be present. Lightheadedness, syncope, and hypotension can occur and may indicate a greater clot burden. Patients with massive PE may present with shock, refractory hypoxemia, or cardiopulmonary arrest.21 Patients with underlying cardiopulmonary disease can develop more severe pathophysiologic consequences of PE with less clot burden.22
The initial laboratory evaluation has also been shown to be insensitive and nonspecific for the diagnosis of PE.23 Most patients with PE have abnormal, but nonspecific, chest radiograph findings.24 Atelectasis, pleural effusions, pulmonary infiltrates, and/or hemidiaphragm elevation have been commonly found on chest radiographs in patients presenting with PE, but these findings are also observed with numerous other conditions. Hampton’s hump and Westermark’s sign are considered to be suggestive of PE, though they are present infrequently. A normal chest radiograph in a patient with dyspnea and hypoxemia without bronchospasm or evidence of anatomic cardiac shunt suggests a high likelihood of PE.25
Arterial blood gas analysis, as well, cannot confirm or exclude the diagnosis of PE. As discussed, arterial blood gas abnormalities commonly found in the setting of PE include arterial hypoxemia, increased alveolar–arterial oxygen gradient, hypocapnea, and respiratory alkalosis. Hypercapnia and respiratory acidosis are signs of underlying cardiopulmonary disease or a massive PE. A normal arterial oxygen pressure and, less commonly, a normal alveolar–arterial oxygen gradient may be present, particularly in patients who are young with otherwise normal lungs.26 While arterial blood gas analysis has been shown to be neither sensitive nor specific for the diagnosis of PE, correlation has been demonstrated between the extent of the clot burden and the degree of the alveolar–arterial oxygen gradient.23
Electrocardiogram (ECG) abnormalities are seen in up to 80% to 90% of cases of PE; however, a wide variety of abnormalities can be observed, and these findings are not sensitive or specific for the diagnosis of PE.27 A major limitation of studies on ECG abnormalities and PE is that most studies have been done on patients after the diagnosis of PE has been confirmed. Tachycardia and incomplete right bundle branch block have been found more often in confirmed than suspected cases.28 S1Q3T3 is a nonspecific and infrequent finding that may suggest right heart strain from a massive or submassive PE. ECG analysis may be most useful to identify or exclude diagnoses other than PE.
D-dimer is a degradation product present in the circulation, which is produced by endogenous fibrinolysis of cross-linked fibrin. A number of different methods have been developed as assays for D-dimer. Enzyme-linked immunosorbent assay (ELISA), enzyme-linked fluorescent assay (ELFA), and newer generation latex agglutination assays have been shown to have a high sensitivity for PE, and when used in conjunction with a clinical scoring system, these have been demonstrated in some studies to have sufficient negative predicative value to rule out the diagnosis of PE in the emergency department setting.29 The specificity of these assays, however, is poor, as D-dimer levels have been found to be elevated with many conditions.30 Additionally, D-dimer assays have been found to be less accurate in hospitalized patients.31 Therefore, D-dimer assays are not useful in confirming the diagnosis of PE or in ruling out the diagnosis of PE for hospitalized patients.
As reviewed above, PE can present with a variety of symptoms, signs, and initial diagnostic studies, though it is unusual to be able to confirm or exclude the diagnosis of PE based on these findings. Initial evaluation based on these data, therefore, is generally most useful in assigning a probability of PE in a particular patient. Additionally, specific quantitation of this probability cannot be determined because of differences in patient populations and discrepancies in study results. However, a qualitative assessment of the probability of PE can usually be determined on the basis of the initial presentation data, which can then be used to guide the subsequent approach to further diagnostic evaluation and therapy. The initial presenting data should be used to determine a qualitative pretest probability for further diagnostic testing as well as to consider when evaluating the potential risks and benefits of therapeutic options.
Ventilation–perfusion (V/Q) scanning has been used frequently as a diagnostic study for the evaluation of acute PE and, under the appropriate clinical circumstances, may yield results that the clinician can use to confirm or exclude the diagnosis of PE. However, V/Q scanning is nondiagnostic for PE in the majority of cases.32,33 The collaborative Prospective Investigation of Pulmonary Embolism Diagnosis (PIOPED) helped shape consensus on the interpretation of V/Q scans. Important information determined from this investigation included the finding that the degree of pretest clinical suspicion is crucial in determining the probability of PE when V/Q scanning is used. It was found that a low probability V/Q scan was associated with PE in 40% of patients with a high clinical suspicion of PE, and that a high probability scan was associated with only a 56% incidence of PE in patients with a low clinical suspicion of PE.34 However, a normal or near normal perfusion scan was found to be associated with a low incidence of PE in all clinical risk groups studied, and a low probability V/Q scan for patients with a low clinical probability was associated with a very low incidence of PE (4%).34 Additionally, a high probability scan in patients with a high clinical suspicion was associated with a 96% incidence of PE.34
Helical or spiral computed axial tomography (helical or spiral CT) requiring the administration of a contrast agent has been developed to evaluate for the presence of PE. Some studies have reported high sensitivity (>95%) and specificity (>95%) for acute PE,35 though more recent and larger studies have reported less sensitivity for this study, suggesting that a negative CT may not be useful to rule out PE.36,37,38,39 Subsegmental pulmonary artery emboli are less readily appreciated by spiral CT, though subsegmental pulmonary emboli are found in a minority of patients presenting with acute PE and their clinical significance is uncertain. Improvements in spiral CT technique for the evaluation of PE are ongoing and include thinner sectioning and three-dimensional reformation, which may improve the sensitivity and specificity of this diagnostic study. Spiral CT has the additional advantage of being able to evaluate for the presence of other potential cardiopulmonary conditions including tumors, lymphadenopathy, emphysema, pleural, and/or pericardial disease. Spiral CT is an increasingly used study to evaluate for the diagnosis of acute PE. While a high specificity has been demonstrated with spiral CT, sufficient sensitivity has not yet been well enough established to exclude the diagnosis of acute PE independent of a low pretest probability. Spiral CT of the thorax with intravenous contrast should, therefore, be considered a confirmatory, rather than screening, test for acute PE.
Magnetic resonance imaging (MRI) and magnetic resonance angiography (MRA) are newer methods of evaluating for acute PE. MRI and MRA have not been studied as extensively as other methods to diagnose PE, though some studies have reported a high sensitivity and specificity for the diagnosis of PE.40 MRI and MRA techniques continue to evolve, and these diagnostic methods show a number of potential advantages over more traditionally employed methods of testing for PE. MRI/MRA is noninvasive, uses a noniodinated intravenous contrast agent, and can demonstrate both perfusion and ventilation characteristics of the lungs. Also, MRI venography has been found to be highly sensitive (97%) and specific (98%) for the detection of DVT, and it can be used to demonstrate pelvic deep vein thrombosis, which cannot be detected by ultrasound.41,42
Echocardiography is a potentially rapidly available method that is insensitive for the diagnosis of acute PE, though it may add information useful in the setting where the diagnosis is suspected. Transthoracic echocardiography (TTE) may rarely allow for direct visualization of emboli in transit to the pulmonary vascular bed or large emboli located in the main, right, or proximal left pulmonary arteries.11 Additionally, TTE may show indirect evidence of acute PE through the demonstration of right ventricular dysfunction in association with right ventricular pressure overload.11 TTE in the setting of acute PE may demonstrate right ventricular dilatation and/or hypokinesis, paradoxical interventricular motion, tricuspid regurgitation, and pulmonary hypertension. Regional right ventricular dysfunction with apex sparing, severe free wall hypokinesis is an echocardiographic finding considered specific for PE.43 In a normotensive patient with PE, TTE may reveal right ventricular hypokinesis, which may be an independent risk factor for early death. Some studies have suggested that normotensive patients who have acute PE and demonstrate evidence of right ventricular strain and dysfunction by echocardiography might benefit from the administration of thrombolytic therapy.11
Transesophageal echocardiography (TEE) expands the limited acoustic window, which limits TTE. Studies have reported a 60% to 80% sensitivity and 95% to 100% specificity for acute PE, and a 90% to 95% sensitivity and 100% specificity for central PE using TEE.11,44 TEE, similar to TTE, can also demonstrate indirect evidence of acute PE suggested by right ventricular abnormalities, though these echocardiographic findings must be interpreted cautiously, as numerous cardiopulmonary conditions may be associated with abnormalities of right ventricular anatomy and function. While TEE has been shown to offer advantages over TTE in the diagnosis of acute PE, the use of TEE is more limited because it requires a higher level of training to perform and interpret the study and also requires conscious sedation of a patient who may have hemodynamic or respiratory compromise.
Pulmonary angiography continues to be considered the gold standard for the diagnosis of PE; however, it is being used less frequently because the diagnosis of PE is being confirmed or excluded to the satisfaction of treating clinicians by less invasive and resource intensive studies (Figure 45-2). An alternative perspective is that pulmonary angiography has historically been underused in the diagnosis of PE and that it continues to be a useful method in the evaluation of acute PE, particularly for those patients who have a high clinical probability of PE and for whom other methods of evaluation have not sufficiently confirmed the diagnosis. Pulmonary angiography has a significant complication rate, which should be considered when deciding about the use of the study. It requires the administration of an iodinated intravenous contrast agent and, therefore, can induce renal failure. PIOPED reported a 0.3% rate of renal failure requiring dialysis associated with pulmonary angiography. It also reported cardiopulmonary compromise requiring intubation or cardiopulmonary resuscitation in 0.4% of the patients undergoing the procedure and an incidence of 0.2% of groin hematoma requiring blood transfusion.45 The death rate associated with the procedure reported by PIOPED was 0.5%. Severely ill patients, such as those in intensive care units, have been shown to have a higher incidence of complications associated with the procedure. Additionally, while pulmonary angiography has been considered the gold standard for the diagnosis of PE, significant interobserver disagreement may occur with the interpretation of the study. Pulmonary angiography remains a potentially useful study for the evaluation of PE despite these issues because of the high diagnostic accuracy of the procedure for PE as well as the ability of the procedure to facilitate the inclusion of hemodynamic data and/or catheter-directed therapy. Once again, however, pulmonary angiography should be considered a confirmatory study, the use of which is based on the pretest probability of the condition as well as the inherent risks and potential benefits of the procedure.
PE may be insufficiently confirmed or excluded on the basis of the available studies previously reviewed. In these instances, it may be useful to proceed with studies to evaluate for the presence of proximal DVT. Proximal DVT is the source for PE and, if DVT is found, can serve as a basis for the institution of therapy with anticoagulation, which is also therapeutic for acute PE. A number of methods with a high sensitivity and/or specificity are available for the diagnosis of proximal DVT including: Doppler ultrasound, impedance plethysmography, contrast venography, MRI, and CT. In cases where proximal DVT has been demonstrated and PE remains a high clinical suspicion without the ability to confirm or exclude the diagnosis of PE, anticoagulant therapy may be administered to treat both conditions. However, under these circumstances, the absence of a definitive diagnosis or exclusion of PE may interfere with the ability to make more informed decisions about future diagnostic strategies and therapy for the affected patient.
The objective of therapy for acute PE is to prevent thrombus extension, as well as early and late DVT and PE. Treatment of uncomplicated PE consists of anticoagulation and/or inferior vena caval filter placement. Therapeutic anticoagulation has generally been achieved in the setting of acute PE with the use of unfractionated heparin (UFH) or low molecular weight heparin (LMWH) preparations. These agents achieve a prompt antithrombotic effect by accelerating the action of antithrombin III to prevent propagation of thrombus.10 These compounds do not dissolve thromboemboli; rather, they allow endogenous fibrinolytic mechanisms to proceed unopposed. Because a significant improvement in outcome is associated with quickly achieving a therapeutic level of anticoagulation for acute PE,46 therapeutic anticoagulation should be instituted promptly and aggressively with the diagnosis or strong suspicion of acute PE. However the potential benefit of therapy should be weighed against the risk of complications.
UFH is administered as an intravenous bolus to attempt to achieve immediate anticoagulation. Therapeutic anticoagulation is subsequently maintained by the administration of UFH as a continuous intravenous infusion titrated to achieve an activated partial thromboplastin time of 2 to 3 times the upper normal limit (60–80 s). Algorithms using weight-based nomograms have been shown to achieve and maintain therapeutic anticoagulation better than nonstandardized methods.47 Adverse reactions associated with UFH anticoagulation include bleeding and heparin-induced thrombocytopenia (HIT), which may be associated with thrombosis. Major episodes of bleeding occur in approximately 2% of patients treated with UFH.48 Risk factors for the development of UFH associated bleeding complications include advanced age, recent surgery, trauma, concurrent use of aspirin, and thrombolytic therapy. HIT is a potential complication, which occurs by the action of heparin-dependent IgG antibodies that activate platelets through their Fc receptors.49 HIT usually develops 5 or more days after the administration of heparin and occurs in approximately 5% of patients treated with UFH.50
LMWH preparations are being more commonly used as the agent of choice to achieve therapeutic anticoagulation for patients with acute PE. These agents have been demonstrated to have comparable safety and efficacy to UFH for the treatment of acute PE, and they may offer some advantages over therapy with UFH.51,52,53,54 LMWHs have a longer half-life, greater bioavailability, and a more predictable dose response than UFH.55 Additionally, LMWHs are subcutaneously administered based on weight and generally do not require laboratory monitoring. In contrast to hepatically cleared UFH, LMWHs are renally cleared and thus require dose adjustments or alternative anticoagulant use for patients with significant renal insufficiency. HIT can be seen with LMWH as well as UFH; however, the incidence of HIT has been found to be less for LMWH.56
Newer agents have been developed to achieve therapeutic anticoagulation. Fondaparinux, a synthetic pentasaccharide and selective inhibitor of activated clotting factor X, has been shown to be safe and effective for the treatment of acute PE57 and is approved by the U.S. Food and Drug Administration (FDA) for this purpose. Fondaparinux is administered as a once daily subcutaneous injection and does not require laboratory monitoring. It is also renally cleared and must be dose adjusted in patients with renal insufficiency. Argatroban and lepirudin are direct thrombin inhibitors approved by the FDA for the treatment of VTE complicated by HIT. Argatroban is hepatically metabolized and is contraindicated in patients with significant hepatic dysfunction, while lepirudin is cleared by renal mechanisms and requires dosage modifications for renal insufficiency. The oral agent ximelagatran and hirudin, which is derived from leech saliva, are direct thrombin inhibitors, which are showing promise in ongoing studies as agents to achieve therapeutic anticoagulation.
Definitive anticoagulation requires the depletion of factor II (thrombin) and takes approximately 5 days to achieve.10 Treatment with UFH or LMWH is, therefore, generally recommended for a minimum of 5 days. Oral vitamin K antagonists, such as warfarin, are usually instituted for continuing outpatient therapy of VTE. Oral therapy with warfarin can be instituted once therapeutic levels of anticoagulation are achieved with UFH or LMWH. Warfarin therapy is then overlapped for a period of 5 days of heparin therapy and until a therapeutic level of anticoagulation is achieved with warfarin, as determined by measurement of the prothrombin international normalized ratio. The target international normalized ratio for the majority of patients being treated for PE is between 2.0 and 3.0. Variation in anticoagulant effect with warfarin therapy can occur due to interactions of the agent with many foods and drugs as well as due to genetic variations in warfarin metabolism.58 The duration of anticoagulation depends on the continuing risk of recurrent VTE versus the risk of complications, generally bleeding, with continued anticoagulant therapy.
In some patients with acute PE, inferior vena cava (IVC) filters may be indicated. IVC filters are used where anticoagulation is contraindicated, in patients with recurrent PE despite therapeutic levels of anticoagulation, and in those patients undergoing surgical embolectomy. While IVC filters do nothing to decrease thrombus formation and have actually been associated with increased rates of DVT,59 they reduce the incidence of clinically significant embolization of thrombi to the pulmonary vasculature and decrease mortality associated with VTE.60 Retrievable IVC filters have been developed and may be useful in patients with a reversible, short-term increased risk of acute PE.61
The use of thrombolytic therapy has been shown to achieve greater initial resolution of thrombus and lower residual pulmonary vascular resistance than therapy with UFH for the treatment of acute PE. However, because of the high rate of bleeding complications associated with thrombolytic therapy, it has not been conclusively demonstrated to offer an advantage over conventional anticoagulation for patients with acute PE and stable hemodynamics.62 However, evidence suggests that thrombolytic therapy for the treatment of massive PE causing hypotension, which otherwise treated may have a mortality rate as high as 30%,63 may result in improved outcomes compared to therapy with heparin.64,65 Additionally, there is ongoing debate as to whether patients with acute PE and stable hemodynamics but with echocardiographic evidence of cardiac right ventricular strain, might benefit from the administration of thrombolytic therapy.66 FDA approved regimens exist for the use of alteplase, streptokinase, and urokinase for the therapy of acute PE. Catheter-directed methods of thrombolytic administration are being developed to attempt to improve efficacy and decrease complication rates, but data are insufficient to make recommendations about the specific role of these methods in the management of acute PE.
Pulmonary embolectomy can be used to relieve pulmonary vascular obstruction caused by acute PE and is considered for patients with massive PE when thrombolytic therapy is contraindicated or ineffective. High morbidity and mortality rates have historically been associated with the procedure; however, procedural techniques have undergone refinement with reports of improved outcomes. A 2002 publication of a study conducted at Brigham and Women’s Hospital on 29 patients presenting with acute PE treated with surgical embolectomy reported an 89% 1-month survival.67 Catheter thrombectomy and/or clot fragmentation are other potential alternatives to attempt to relieve cardiac right ventricular failure and cardiogenic shock associated with massive PE.68,69,70,71 Many patients presenting with massive PE have contraindications to the administration of fibrinolytics, and few hospitals are able to have constant availability of personnel and resources to perform surgical embolectomy. Catheter-directed methods may, therefore, become a feasible approach in the management of acute, massive PE, though additional studies are needed to define their safety and efficacy.
Appropriate prophylaxis has been well established to decrease the incidence of VTE in a wide variety of patients and clinical settings; however, VTE prophylaxis remains grossly underused. Mechanical and/or pharmacologic methods are available to reduce patient’s risk for PE. A thorough investigation for risk factors should be conducted to determine the specific prophylactic measures employed. Mechanical prophylactic devices include graduated compression stockings, intermittent pneumatic stockings, and IVC filter placement. Pharmacologic agents used to reduce the risk of VTE include subcutaneous UFH, LMWH, warfarin, and fondaparinux. UFH at a dosage of 5000 units subcutaneously every 8 hours has been shown to be an effective means to decrease the incidence of VTE in patients at low to moderate risk for VTE.72 LMH is comparable to UFH in efficacy and complications for VTE prophylaxis73 and has been shown to be more effective than UFH in specific high-risk patient populations, including hip and knee replacement patient and multitrauma patient populations.73,74 Very high-risk patients may benefit from combined mechanical and pharmacologic prophylactic interventions. Comprehensive consensus guidelines exist to guide VTE prophylaxis for specific patient populations.75 Computer-assisted VTE prophylaxis protocols have been shown to improve rates of appropriate prophylaxis administration and reduce the risk of VTE76 and are coming into common hospital usage.
Venous air embolism (VAE) as a cause of mortality was initially described in animals by the Italian naturalist Redi in 1667.77 It was reported in humans by Morgagni in 1769 based on postmortem findings,78 and subsequently by Beauchesne (1818) and Magendie (1821), both reports in association with neck tumor excision.79 VAE occurs in a variety of circumstances, including diagnostic and therapeutic medical and surgical interventions and their complications. These circumstances include but are not limited to neurosurgical procedures, particularly in the sitting position80,81,82; intracardiac pacemaker placement,83,84 orthopedic and general surgical procedures85; obstetrical/gynecologic cases86,87,88,89,90,91; trauma92,93; diving/decompression accidents94,95; positive pressure ventilation96; percutaneous lung biopsy97,98,99; penetrating lung injury100,101; vascular cannulation102,103,104,105,106,107,108,109,110,111; hemodialysis112,113; mechanical ventilation96,114,115; epidural catheter insertion116; and contrast injection117,118,119 (Table 45-2).
Surgical | Medical |
---|---|
Neurosurgery | Cardiology |
Craniotomies | Pericardiocentesis |
Laminectomies | Pacemaker placement |
Pin holder placement | Cardiac catheterization |
Shunt placement | Nephrology |
Cardiac surgery | Hemodialysis |
Vein harvesting | Critical care medicine |
General surgery | Placement/maintenance/removal of central venous/PA catheters |
Thoracic trauma | Mechanical ventilation |
Head and neck trauma | Diving/decompression accidents |
Craniofacial surgery | Radiology |
Transplantation | Contrast injection |
Pneumoperitoneum from ruptured viscus | Arthrography |
Obstetrics/Gynecology | Percutaneous lung biopsy |
Caesarian section | Gastroenterology |
Hysteroscopy | Air entry into veins during upper/lower endoscopy |
Laparoscopy | Air entry into veins during ERCP |
Orogenital sex during pregnancy | |
Epidural catheter insertion | |
Orthopedics | |
Pulse lavage of fractures | |
Arthroscopy | |
Total hip arthroplasty | |
Spine surgery in prone position | |
Dentistry | |
Root canal procedures |
Distinction is made between venous, or pulmonary air embolism, and arterial air embolism, as their pathologic and clinical manifestations, as well as therapeutic interventions, may differ.120 In VAE, air enters the venous circulation and passes into the right heart and lungs. The deleterious effects in this circumstance are generally exerted in the pulmonary121 and cardiovascular systems. It is possible that the filtering capacity of the lungs may be exceeded, allowing air to pass into the arterial system,122,123,124,125 and causing an arterial air embolus, even in the absence of an intracardiac defect.126,127 Arterial air embolism may also be the result of air that enters the venous circulation and passes through a patent foramen ovale, which may occur in up to 30% of the population based on autopsy studies.128,129,130,131 This phenomenon is referred to as a paradoxical embolism, and more commonly has manifestations affecting the heart and central nervous system. Either venous or arterial embolism may result in death.
The incidence of VAE in multiple studies varies depending on the patient population observed and the method of detection employed. The highest incidence of VAE occurs in neurosurgical patients undergoing surgery in the sitting, or Fowler’s, position. This approach was previously used more commonly,81 but is used now primarily in high midline, posterior fossa procedures.82 The incidence of VAE in retrospective and prospective studies of neurosurgical patients has ranged from 2% to 58% (Table 45-3)80; however, with the introduction of TEE, the incidence has been reported to be as high as 76%.132 Many of these VAE are small, but may herald the advent of a larger and clinically more significant event.133
Authors | Year | Type of Study | No. of Cases | Incidence (%) | Method of Detection |
---|---|---|---|---|---|
Bithal et al.495 | 2004 | Prospective | 334 | 28 | Capnography |
Young et al.496 | 1986 | Retrospective | 255 | 30 | Capnography or Doppler |
Papadopoulos et al.132 | 1994 | Prospective | 62 | 76 | TOE |
Michenfelder et al.497 | 1972 | Prospective | 69 | 32 | Doppler |
Slbin et al.498 | 1976 | Retrospective | 180 | 25 | Doppler |
Voorhies et al.499 | 1983 | Prospective | 81 | 50 | Doppler |
Standefer et al.500 | 1984 | Retrospective | 488 | 7 | Doppler |
Matjasko et al.501 | 1985 | Prospective and retrospective | 554 | 23 | Doppler |
Black et al.502 | 1988 | Retrospective | 333 | 45 | Doppler |
The pathophysiology of VAE includes mechanical effects resulting from obstruction as well as inflammatory mediated events resulting from the release of thromboxane and free radicals with subsequent endothelial damage and increased capillary permeability.83,134,135,136,137,138 Air can enter the venous system at a communication between a gas (usually the atmosphere) and the open venous system where the ingress of gas is favored by a pressure gradient.106 The air can either be injected under pressure, or it may move along a gradient of atmospheric to subatmospheric pressure. The former situation is seen in the setting of an injection of air via a syringe or pressurized infusion device. The latter mechanism occurs in the setting of surgery or other invasive procedures, where veins above the level of the heart are exposed to the atmosphere. This second mechanism may be a particular problem in the presence of increased negative intrathoracic pressure, such as in patients with obstructive lung disease. Once air enters the pulmonary circulation, it causes an increase in pulmonary pressure because of obstruction and reflex pulmonary vasoconstriction.139 This increase in pulmonary vasoconstriction leads to an increase in right ventricular end-diastolic pressure and right atrial pressure. Additionally, this vascular obstruction causes an increase in dead space leading to hypercapnia and a decrease in end-tidal carbon dioxide (ETCO2).140,141
The adverse effects of VAE cannot be attributed solely to vascular obstruction by air bubbles and vasoconstriction. In experimental animals, a complex interaction of air and blood occurs in the right heart as air and blood are whipped together, creating a network of air bubbles, fibrin stands, erythrocytes, fat globules, and platelet aggregates.142,143 This fibrin mesh is then ejected into the terminal branches of the pulmonary arteries. The role of the neutrophil in VAE-induced lung injury has been investigated by numerous authors.83,135,137,142,143,144,145,146,147,148 Neutrophils are sequestered in the lungs during VAE, and in the experiments by Albertine et al. were shown to clump around air bubbles and attach to the endothelium of the pulmonary arterioles, distorting the microvascular architecture, and producing endothelial cell gaps.137 This disruption of the endothelium led to increased lung lymph flow and protein flux. The cascade of events initiated by the release of thromboxane and leukotrienes causes increased alveolar–capillary permeability with resultant edema and a dose-dependent inactivation of surfactant.83 All of these factors may lead to an increase in intrapulmonary shunting, decreased arterial oxygen tension, and decreased pulmonary compliance.149
A classic study of VAE done by Durant et al.150 in 1947 demonstrated that mortality during VAE was associated with the amount of air embolized, the speed at which it entered the body and the body position at the time it occurred. A fatal dose is considered to be 300 to 500 mL of air at a rate of 100 mL/s, which can be achieved with a 14-gauge needle and a pressure gradient of only 5 cm H2O between air and venous blood.106,107 In the critically ill and unstable patient, smaller volumes of air may also be fatal.151 A grading system has been developed for VAE in the neurosurgical patient population based on emboli size, monitoring device detection, and physiologic changes occurring in the operating theatre. A large embolus (>50 mL) may require abandonment of the surgical procedure if any concern exists for recurrence of the VAE.82
The clinical manifestations of VAE may be nonspecific and require vigilance with a high index of suspicion to promptly identify the occurrence of VAE. The symptoms associated with VAE may mimic a variety of other disorders. Faintness, chest pain, lightheadedness, and a feeling of impending doom have been reported106,152; and in one series, 100% of patients had the onset of sudden dyspnea.153 A classic “gasp” reflex caused by acute hypoxemia106,107 has been described. Physical examination findings are also nonspecific. The typical mill wheel murmur is a splashing auscultatory sound heard over the precordium because of the presence of gas in the cardiac chambers and great vessels, although it is a late finding.119,150 More commonly, a harsh systolic murmur or normal heart sounds are heard.154 Chest examination may reveal rales or wheezing, and abnormal mental status or neurologic findings are relatively common. Kashuk et al.153 reported a 42% incidence of central nervous system manifestations in a series of 24 cases of air embolism with abnormalities ranging from altered mental status to coma. Tachypnea, tachycardia, and hypotension may also occur, heralding the onset of further hemodynamic compromise.120 Central nervous system manifestations may develop secondary to hypoxemia and hypoperfusion as a result of hemodynamic compromise or as a result of cerebral arterial embolism via intracardiac or intrapulmonary shunts.
Similar to the clinical findings associated with VAE, routine laboratory analysis in this disorder may be nonspecific. Arterial blood gas analysis may show hypoxemia of varying severity as well as hypercapnia.155 Review of electrocardiographic data may demonstrate sinus tachycardia, right heart strain, and myocardial ischemia.156,157,158 Chest radiography is most commonly normal, but may exhibit a variety of additional findings such as pulmonary edema, characteristic bell or cone shaped air collections in the main pulmonary artery (Figure 45-3), enlarged pulmonary arteries, focal pulmonary edema, atelectasis, intracardiac air, and air in the hepatic venous circulation.159 CT of the chest may be of diagnostic value by demonstrating air in the vasculature and cardiac chambers,118 and has also been implicated as a cause of VAE during the administration of intravenous contrast. Knowledge of the most frequent anatomic locations for air emboli to collect may help the radiologist in differentiating between air embolism and other phenomena117,118,160 (Figure 45-4). Central nervous system imaging may be performed if cerebral air embolism as a complication of VAE is suspected. However, CT of the brain is rarely diagnostic in this setting, as the CT must be obtained immediately after the event because of the rapid resorption of air from cerebral arterioles.161 A restricted diffusion pattern on MRI may be seen in cerebral air embolism, but further evaluation of this MRI pattern as characteristic for cerebral air embolism is required.83,162
FIGURE 45-3.
Anteroposterior chest radiograph. Main pulmonary artery demonstrates characteristic bell, or cone-shaped, air collections. Additionally, focal oligemia is suggested in the left upper lobe.
Reproduced, with permission, from Kizer KW, Goodman PC. Radiographic manifestations of venous air embolism. Radiology. 1982;144:35-39.

FIGURE 45-4.
Diagrams show frequent locations of air emboli (depicted as large black dots) observed on axial CT scans in (A) vessels and (B) cardiac chambers after insertion of an intravenous cannula or injection of contrast agent. The numbers indicate the frequency of air emboli at the corresponding locations in 677 CT examinations with contrast material enhancement. In six patients, air embolism was found in the subclavian or brachiocephalic vein, which are not shown on the figure.
AA, ascending aorta; DA, descending aorta; LA, left atrium; LPA, left pulmonary artery; LV, left ventricle; MPA, main pulmonary artery; RA, right atrium; RPA, right pulmonary artery; RV, right ventricle; SVC, superior vena cava.
Reproduced, with permission, from Groell R, Schaffler GJ, Rienmueller R, et al. Vascular air embolism: location, frequency, and cause on electron-beam CT studies of the chest. Radiology. 1997;202:459-462.

Echocardiography, both transthoracic (TTE) and transesophageal (TEE), is well documented as a valuable tool for monitoring and detecting VAE.108,163 Precordial Doppler remains an essential noninvasive first-line tool for monitoring patients during high-risk surgical procedures because of its availability and high sensitivity.164,165,166 Additionally, echo detects VAE well before the earliest evidence of physiologic changes.167 Proper probe positioning and verification of accurate positioning is crucial to its efficacy, and left parasternal positioning is as sensitive as right parasternal.168,169 However, TEE is the most sensitive method of detection of VAE and is considered the gold standard.106,107,127,151 Its use is limited primarily by cost and the advanced level of training required to attain operator expertise in study performance and interpretation.119,170 In institutions where TEE is used, the procedure allows additional information to be obtained including an assessment for the presence of a patient foramen ovale when the probe is placed prior to beginning the surgical procedure.
Other forms of monitoring and detection during surgical procedures include capnography, pulmonary artery catheters, air aspiration via a central venous catheter, pulse oximetry, arterial blood gas analysis, and mass spectrometry. VAE results in a decrease in ETCO2, and is always significant when detected by this method.82 Pulmonary artery catheter monitoring is invasive, but has similar sensitivity to capnography82 and can demonstrate an increase in pulmonary arterial pressure with VAE when employed as a monitoring device. The pressure gradient measured between the left and right atria may also help in assessing the potential for paradoxical air embolism. Pulmonary artery catheters are of no use in aspirating air if a VAE is detected,166 but an appropriately placed central venous catheter may aspirate air and confirm VAE.107,140,171 Mass spectrometry to measure end-tidal nitrogen may be used to diagnose VAE, but is not available at most institutions.82
Treatment of VAE begins with methods to prevent and detect its occurrence, followed by supportive care and standard resuscitation. Measures to prevent the occurrence of VAE should be undertaken in any circumstance where the risk for VAE exists. These measures include the previously reviewed monitoring during surgical procedures at risk for VAE. Additionally, volume loading to reduce negative venous pressure at the wound level should be employed preemptively, with some authors recommending colloid over crystalloid because of a more prolonged increase in vascular volume.82 If VAE is suspected to have occurred, the surgical field should be irrigated so that no further entrainment of air can occur, and the surgical site should be lowered below the level of the heart if possible.119 Neck tourniquets may be used to increase cerebral venous pressure either during periods of high risk for VAE or during episodes of recorded VAE. However, care should be taken to ensure that the pressure generated by the tourniquet is in the venous and not arterial range, and that the potential complications of increased cerebral edema or carotid plaque disruption are considered. If a central venous catheter is appropriately located at the superior vena cava-right atrial junction, air may be aspirated via the catheter, with multiorifice catheters being more effective than single lumen catheters in aspirating air.82,172,173 Even with appropriate positioning of the catheter, studies have shown that only approximately 50% of the air will be successfully aspirated.107,149 In addition to prevention, detection, and aspiration of air, supportive therapies such as volume expansion, vasopressors to support organ perfusion, and 100% FiO2 to facilitate the washout of nitrogen from the air bubble should be employed. Nitrous oxide should be avoided as an anesthetic agent, but if being used, should be stopped if VAE is suspected. The deleterious effects of nitrous oxide are due to the differential solubilities of nitrous oxide and nitrogen, which allow nitrous oxide to replace nitrogen in the blood, potentially increasing the gas bubble volume and rendering lethal what might have otherwise been an innocuous air bubble.140,174,175 The patient should be moved to the left lateral decubitus position to prevent air lock at the pulmonary outflow tract.176 Closed chest cardiac massage has been used successfully in the resuscitation of patients with VAE,177 and was found to improve survival to the same degree as left lateral decubitus positioning and air aspiration in the canine model.178 Left lateral decubitus positioning reduced mortality in one series of 93 cases of air embolism from 88% to 33%.79 If evidence for cerebral air embolism exists, arrangements should be made promptly for hyperbaric oxygen therapy in an attempt to decrease the size of the air emboli and allow passage into smaller vessels perfusing smaller areas of the brain.179,180 Expeditious transport should be arranged, and ground transport for short trips is preferred due to the potential for worsening of a patient’s condition in the setting of bubble expansion with a decrease in atmospheric pressure as a result of an increase in altitude. If long-distance transport is required, aircraft should be pressurized to 1.0 ata. If helicopter transport is used, maintaining an altitude of 300 to 500 feet, volume loading, and 100% FiO2 are all recommended.181
Amniotic fluid embolism (AFE) is a catastrophic complication of pregnancy, which classically presents with hypoxemia, cardiopulmonary collapse, and disseminated intravascular coagulation during labor or in the immediate postpartum period. Initially described by Meyer182 in 1926 and further clarified in the early 1940s by Steiner and Lushbaugh,183 AFE has generated intense interest and has been widely reported and reviewed in the obstetric, anesthesia, pulmonary, and critical care literature over the ensuing years. More than 400 cases have been reported in the literature, and yet the incidence, mortality, and diagnostic criteria are still controversial.
The reported incidence of AFE has varied, with many authors citing ranges from 1 in 8000 to 1 in 80 000.183,184,185,186,187,188,189 Albeit rare, AFE is a significant disorder because of the high associated maternal mortality and morbidity. An early review of 272 cases reported in the English literature cited a maternal mortality rate of 86%.190 Later data from the U.S. National Registry demonstrated a maternal mortality of 61%, but included significant additional maternal morbidity with only 15% of patients surviving neurologically intact.185 In a population-based study of 1 094 248 deliveries from California, a frequency of 1 in 20 646 deliveries was reported with a maternal mortality of 26%.184 However, a subsequent case–fatality rate in a large Canadian cohort of patients was only 13%.186 The United Kingdom Amniotic Fluid Embolism Registry reported an incidence of 1 in 120 000 cases and a maternal mortality of 37%.
AFE is a significant cause of maternal mortality in the United States, United Kingdom, and Australia and has accounted for approximately 7.5% to 10% of all maternal deaths in various long-term analyses.185,191,192,193,194,195 Fifty percent of AFE-related mortality occurs acutely within the first hour of presentation, requiring a proactive and informed response to the acute presentation.86,196,197,198 Fetal mortality is also high, approaching 40% in some studies.185,199 There is no consensus on the occurrence of AFE in subsequent pregnancies owing to its significant maternal morbidity and mortality, but several cases of uncomplicated pregnancies after AFE are reported in the literature.194,200,201,202,203,204,205 It is apparent that in spite of years of AFE case compilations and established AFE registries, the incidence and mortality of AFE have not been clearly defined.
Multiple factors contribute to the difficulty in determining the true incidence and mortality of AFE. These factors include inaccuracies in reporting causes of maternal deaths,191,206,207 reliance upon multiple case reviews and small patient series, variations in diagnostic criteria and study design among case series and registries,184,185 and publication bias owing to selective reporting of severe cases.186 Additionally, AFE may occur with disparate clinical presentations and is difficult to specifically diagnose in subclinical or nonfatal cases.207,208,209 The more recent findings of lower reported maternal mortalities than those seen in earlier case reports and case series may reflect both advancements in intensive care medicine and supportive therapies for patients treated for AFE over the last few decades as well as recognition that subclinical or milder cases of the disorder exist.184,185,193,210
The pathophysiology of AFE remains poorly understood because of its low incidence and difficulties in duplicating the event in animal studies. Amniotic fluid increases from 50 to 1000 mL at term and contains multiple vasoactive materials, as well as various elements of fetal origin including: fetal squamous cells (squames), lanugo hairs, vernix caseosa, gut-derived mucin, and occasionally, bile containing meconium.190,211 While the pathophysiology of AFE is not clearly delineated, it is presumed that amniotic fluid containing fetal debris enters the maternal circulation via uterine venous sinuses within the endometrium or endocervix.184 The constituents of the amniotic fluid then travel to the pulmonary vascular bed and cause acute right heart failure followed by left heart failure, an assumption based primarily on data derived from animal models. There are no case reports in humans where central hemodynamic monitoring was in place prior to the onset of AFE to document this presumed hemodynamic response,212 and there are only rare instances of normative hemodynamic data documented in the gravid female.213 The currently available animal and human hemodynamic data suggest a biphasic pathophysiology for AFE,214 with initial right heart failure secondary to pulmonary vasoconstriction and vascular occlusion, followed by left heart failure because of inadequate filling and coronary ischemia in the setting of hypoxemia.197,214 In animal studies with documented central hemodynamic data, severe acute right heart failure was not prominent, but the animals had previously undergone intubation and general anesthesia, which may have blunted their response to the acute insult of amniotic fluid introduction into the pulmonary circulation.199 However, it does appear that the most severe cardiopulmonary response occurred in the groups with meconium stained amniotic fluid, which supports observations in both animals199 and humans. Another criticism of many animal studies is that heterologous versus autologous amniotic fluid was infused, which would not mimic true AFE.215 The infusion of autologous amniotic fluid in two primate studies failed to demonstrate the dramatic clinical syndrome of AFE seen in other animal studies.216,217 Overall, results of available animal studies are conflicting and difficult to compare because of differing experimental designs and interspecies variation in clinical response to experimental AFE.218
Originally, the cascade of events causing AFE was felt to be caused by mechanical occlusion of the pulmonary vasculature in the setting of tumultuous labor with a large volume of amniotic fluid infused under pressure. However, the contribution of tumultuous labor and large volume infusion cannot explain the documented occurrence of AFE early in pregnancy, when the volume of amniotic fluid is small. The presumption of tumultuous labor intuitively also leads to a concern for an increased risk of AFE with uterine stimulants in the induction or augmentation of labor. In Morgan’s early series190 and the later National Registry data,185 the authors dispute that tumultuous labor and uterine hyperstimulation as a result of labor induction are associated with AFE. Instead, Clark proposes that the uterine hypertonicity seen in AFE is a result of the cardiopulmonary compromise associated with the disorder, rather than the cause of the insult. Wagner strongly criticized the statistical methods used by these authors as well as the validity of their assertions, and called for greater scientific scrutiny of maternal deaths and the association of AFE with uterine stimulants for induction or augmentation of labor.219 A recent large population-based cohort186 did show an association of AFE with the use of uterine stimulants, leaving the question of uterine stimulants as a predisposing factor in the development of AFE controversial. Multiple attempts have been made to determine other risk factors for AFE. The best data at this point suggest the strongest associations for the following risk factors: advanced maternal age, meconium stained amniotic fluid, placental abruption, placenta previa, fetal death, and multiparity.184,186,220,221,222
Similarities in clinical characteristics of AFE to anaphylaxis and sepsis have led Clark and others to propose an “anaphylactoid” syndrome of pregnancy, with small volumes of amniotic fluid precipitating a vasoactive procoagulant cascade leading to pulmonary edema, vascular thrombosis in situ and coagulopathy183,184,185,206,223 (Figure 45-5). An anaphylactoid theory is supported by data from the National Registry, which demonstrated that AFE was more common in association with male fetuses, and in patients who reported a history of atopy. It is important to recognize, however, that other symptoms associated with anaphylaxis were not present to the same degree in AFE, notably bronchospasm and urticaria.224 Bronchospasm was seen in only 15% of the National Registry patients, and in 1% of the patients in Morgan’s early analysis, but is seen in approximately 40% of patients with anaphylaxis.225 The association of male fetuses and AFE noted by Clark (67%) was also not identified in a large population–based study of AFE, where 49% of the fetuses were female.184
The diagnosis of AFE is largely clinical, with a classic presentation of hypoxemia, hemodynamic instability, altered mental status, and disseminated intravascular coagulation occurring during active labor and delivery or in the immediate postpartum period. AFE has additionally been reported as a consequence of abdominal trauma, amniocentesis, and first and second trimester abortions.120,196. The presenting features of AFE in the National Registry were seizure or seizure-like activity (30%), dyspnea (27%), fetal bradycardia (17%), and hypotension (13%). However, during the course of the disease, 100% of patients had hypotension and fetal distress, with 93% experiencing pulmonary edema or acute respiratory distress syndrome (ARDS) and 87% having cardiopulmonary arrest, 37% of those within 5 minutes of symptom onset. Coagulopathy was associated with AFE in 40% of patients.185 In contrast to the National Registry data, 51% of patients in Morgan’s series presented with respiratory distress. Dyspnea may be more difficult to define in the pregnant patient, as up to 60% of pregnant women may develop the dyspnea of pregnancy during the first trimester, even before the mechanical effects of the gravid uterus on the diaphragm are apparent.226 However, the presentation of AFE, particularly in fatal cases, is generally dramatic with sudden rather than gradual onset of symptoms, including dyspnea. This rapid onset of symptoms seen in most patients with AFE prompted Benson to include sudden onset of symptoms as a key feature in his proposed criteria for diagnosis of AFE.209
The finding of fetal squamous cells in the maternal circulation was previously felt to be pathognomic for the condition, but the significance of this finding has been refuted because of the high false-positive rate of squamous cells seen in the maternal circulation of patients without AFE, and because of the difficulty in differentiating between maternal and fetal squamous cells.206,227,228,229,230,231 A key observation may be that the squamous cells seen in patients with AFE, unlike those in patients without AFE, are coated with leukocytes in many cases; this finding may suggest a maternal reaction to a foreign (fetal) antigen. Additionally, the squames in patients with documented AFE may also be seen pathologically in association with other fetal debris.207,227
Serologic studies have more recently been proposed to assist in the diagnosis of AFE, including serum tryptase levels to assess for mast cell degranulation,232,233 and a monoclonal antibody (TKH2) that recognizes the sialyl Tn structure characteristically found in meconium and amniotic fluid.234,235 Serum tryptase may be useful in both antemortem and postmortem diagnosis of AFE given its more prolonged serum half-life,233,236 although some dispute the postmortem significance of an elevated tryptase level.237 Other investigators have found associated low levels of complement in patients with AFE,238,239 elevated zinc coproporphyrin levels,239,240 and increases in tissue factor241 in amniotic fluid versus in maternal serum. None of these tests has been validated in a series of AFE patients, and all have limited practical utility at present. Currently, the finding of fetal debris in the pulmonary circulation at autopsy with a compatible clinical scenario defines the diagnosis of AFE, although routine hematoxylin and eosin staining may be insufficient to demonstrate fetal elements and special stains may be required.211,242
Clinical findings suggest the diagnosis in nonfatal cases, with laboratory and imaging studies providing supportive evidence. The normal arterial blood gas in the pregnant patient demonstrates a mild chronic respiratory alkalosis with compensatory metabolic acidosis.226 In the pregnant patient with respiratory distress and shock, the arterial blood gas may show development of acute respiratory alkalosis and acute metabolic acidosis in addition to the chronic acid base changes associated with pregnancy. In those patients with AFE who develop coagulopathy, numerous coagulation abnormalities may be seen. These include thrombocytopenia, increased fibrinopeptide A, increased D-dimer, decreased antithrombin III, decreased fibrinogen, increased fibrin degradation products, and increased prothrombin and partial thromboplastin times.
The majority of imaging studies documented in patients with AFE are those that can be done at the bedside. Chest radiographs obtained in the patient with AFE are nonspecific. The radiograph may be normal or may demonstrate bilateral pulmonary infiltrates consistent with either cardiogenic or noncardiogenic pulmonary edema.86,243,244 Ventilation–perfusion scans may show multiple perfusion defects, which is a nonspecific finding and can also be seen in pulmonary thromboembolism, a disorder for which pregnant patients are also at high risk. Because of the dramatic presentation of AFE in many cases and the associated hemodynamic instability, extensive radiographic imaging to include CT with PE protocol is usually impractical acutely. There is no data on characteristic CT findings in AFE or CT methods to distinguish AFE from PE. TTE and TEE, however, can be done at the bedside and may be useful in the short-term diagnosis of AFE.245,246 Two case reports used TEE acutely in the abrupt decompensation of a patient in labor—one within 10 minutes of decompensation after elective caesarean section,247 and one within 30 minutes of caesarean section for fetal distress.245 In both cases, acute right heart failure with normal left ventricular ejection fraction was seen on TEE (Figure 45-6). Neither case demonstrated evidence of acute pulmonary artery thromboembolism, either by Doppler examination of the pulmonary arteries in the first case245 or by direct surgical exploration of the pulmonary arteries during cardiopulmonary bypass in the second case.247 Autopsy findings in the first case revealed pulmonary edema with extensive microvascular plugging of pulmonary capillaries by fetal squamous epithelial cells, and the second patient had an uneventful recovery. These reports are intriguing, as they may provide evidence for the right heart failure postulated as the initial phase of hemodynamic response to AFE in humans, and could have therapeutic implications as well. In most instances, however, the echocardiogram will demonstrate left ventricular failure unless done immediately during the acute decompensation. The electrocardiographic findings are nonspecific in AFE, but may include evidence of ischemia or right heart strain.
FIGURE 45-6.
(A) Transesophageal echocardiogram view taken with 10 minutes of acute amniotic fluid embolus, demonstrating dilated right ventricle (RV) and small left ventricle (LV). (B) Transesophageal echocardiogram reflecting a return to normal size and function of the right and left ventricles after intervention including cardiopulmonary bypass.
Reproduced, with permission, from Stanten RD, Iverson LIG, Daugharty TM, Lovett SM, Terry C, Blumenstock E. Amniotic fluid embolism causing catastrophic pulmonary vasoconstriction: diagnosis by transesophageal echocardiogram and treatment by cardiopulmonary bypass. Obstetr Gynecol. 2003;102:496-498.

The astute intensivist must possess an understanding of the differences in physiology of the pregnant patient in order to optimally support the patient with AFE. The cardiac output and plasma volume are markedly increased in the pregnant patient at term, in combination with a relative decrease in colloid oncotic pressure, which may predispose to the development of pulmonary edema. These cardiovascular physiologic changes, coupled with hormonal changes in pregnancy, also promote increased mucosal edema. This increase in mucosal edema mandates downsizing both nasogastric and endotracheal tubes and increases the risk for a difficult airway.248 Minute ventilation increases as pregnancy advances owing to changes in metabolic rate, changes in respiratory mechanics, and increases in serum progesterone. Progesterone has a direct respiratory stimulant effect on the respiratory center, and increases in minute ventilation as pregnancy progresses correlate with increasing maternal serum progesterone concentrations.226,249 Functional residual capacity decreases as pregnancy advances as a result of decreases in expiratory reserve volume and residual volume. The combination of decreased functional residual capacity and increased minute ventilation leads to an overall decrease in maternal oxygen reserve. Progesterone also causes smooth muscle relaxation and decreases lower esophageal sphincter tone, which is further decreased by stomach displacement as pregnancy advances, contributing to aspiration risk. The glomerular filtration rate and creatinine clearance are also increased in pregnancy, and renal compromise may be present and should be considered at lower levels of BUN/Cr.
The treatment of AFE is largely supportive, with the goal in fulminant cases of providing cardiopulmonary support, including Advanced Cardiac Life Support; reversing hypoxemia with intubation and mechanical ventilation; reversing shock with volume resuscitation, pressor agents, and inotropic therapy as needed; using blood products as indicated to treat hemorrhage and reverse coagulopathy; and treating with anticonvulsants and airway management to control seizures. A multidisciplinary approach to the treatment of AFE, with close collaboration between the intensivist, obstetrician, and hematologist or neurologist as indicated, is imperative in ensuring an optimal outcome (Figure 45-7).
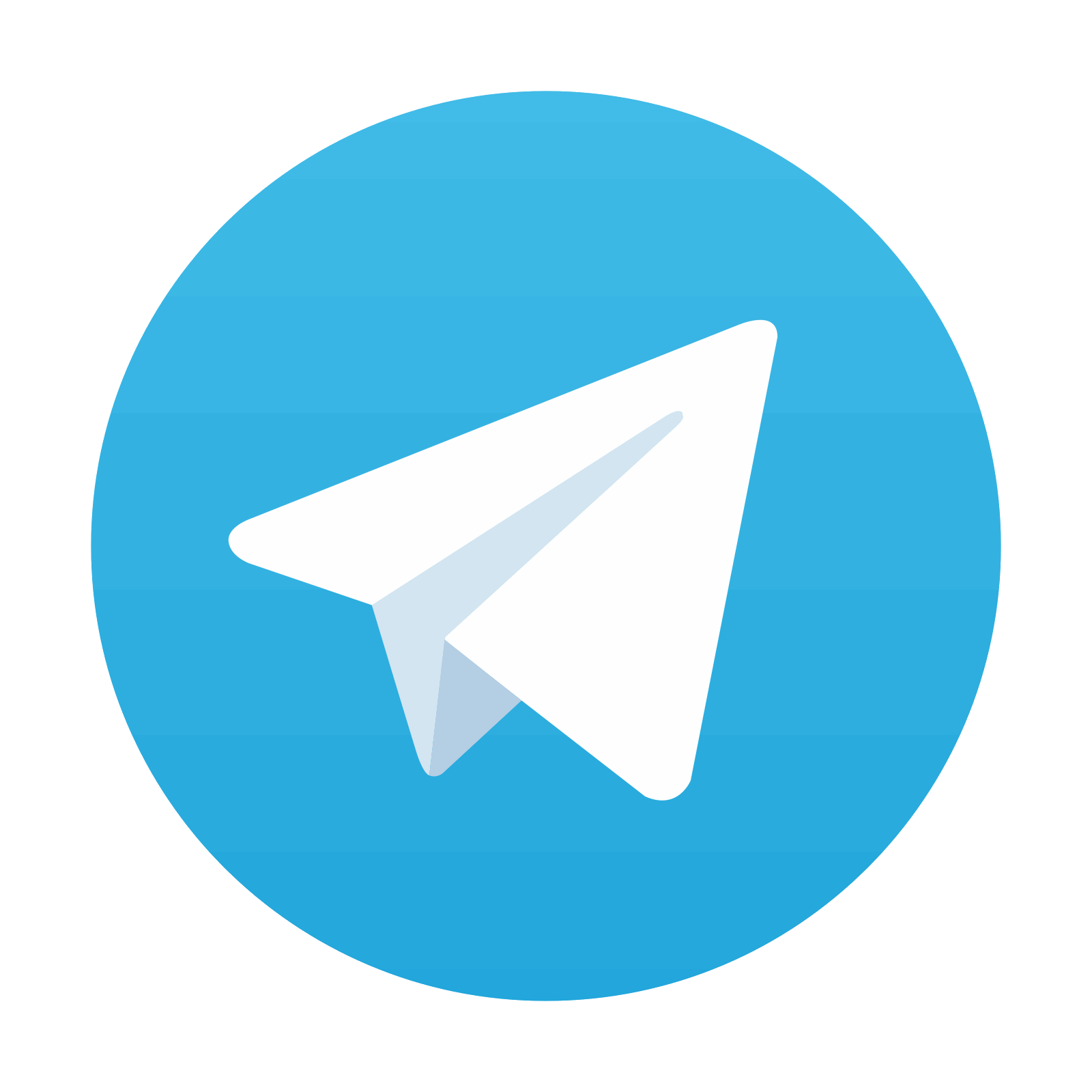
Stay updated, free articles. Join our Telegram channel

Full access? Get Clinical Tree
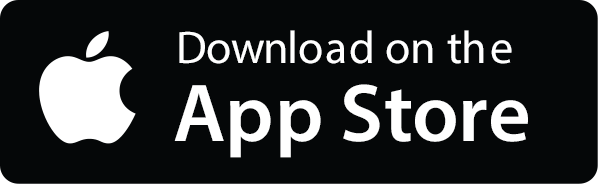
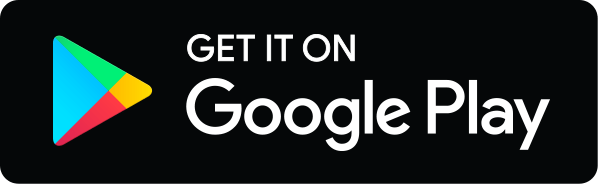