4 Electrocardiography
Leads
Twelve leads are routinely used to record the body surface ECG: three bipolar limb leads labeled I, II, and III; three augmented limb leads labeled aVR, aVL, and aVF; and six unipolar chest leads labeled V1 through V6 (Fig. 4-1). In the bipolar limb leads, the negative pole for each of the leads is different, whereas in the unipolar chest leads, the negative pole is constant and created by the three limb leads. This is referred to as Wilson’s central terminal. The positive chest lead is, in effect, an exploring lead that can be placed anywhere. In children, the routine ECG often includes leads placed on the right side of the chest in positions referred to as V3R and V4R. Similar right-sided chest leads are often used in adults to diagnose right ventricular infarction, and one or more leads placed on the back are sometimes used to diagnose posterior wall infarction.
Electrocardiographic Waveform
The ECG waveform consists of a P wave, a PR interval, the QRS complex, an ST segment, and T and U waves. The relationship of these waveform components to the underlying action potentials of the various cardiac tissues is shown in Figure 4-2A, as is an example of a normal 12-lead ECG in Figure 4-2B. The P wave reflects depolarization of the atria, the QRS complex reflects depolarization of the ventricles, and the ST segment and T wave reflect repolarization of the ventricles. The U wave occurs after the T wave and is thought to be an electromechanical event coupled to ventricular relaxation.
P Wave
Impulses arising from an ectopic atrial focus are associated with P waves whose shape depends on the location of the focus. If the abnormal focus is in close proximity to the sinus node, the sequence of atrial activation will be normal or nearly normal, and the P wave will resemble the normal sinus P wave. The more distant the ectopic focus is from the sinus node, the more abnormal will be the sequence of atrial activation and the P-wave configuration. For instance, impulses originating in the inferior portion of the atrium or within the AV node will depolarize the atria in a retrograde, superiorly oriented direction and will be associated with the P waves that are inverted in leads II, III, and aVF and upright in lead aVR (Fig. 4-3).
QRS Complex
The QRS complex is altered in both shape and duration by abnormalities in the sequence of ventricular activation. These include the bundle branch blocks (Fig. 4-4A), the fascicular blocks, ventricular preexcitation (Fig. 4-4B), nonspecific intraventricular conduction disturbances, and ectopic ventricular beats (Fig. 4-4C). The increase in QRS duration may range from a few milliseconds, as in the case of fascicular blocks, to more than 40 milliseconds, as with bundle branch blocks. The fascicular blocks reflect conduction slowing in one fascicle of the left bundle and are characterized by a shift in electrical axis and subtle changes in the initial portion of the QRS complex. The bundle branch blocks are caused by conduction slowing or block in the right or left bundle branch, usually caused by fibrosis, calcification, or congenital abnormalities involving the conducting system. They are associated with more pronounced abnormalities in the sequence of ventricular activation than are the fascicular blocks and thus with more significant changes in the QRS configuration. Intraventricular conduction abnormalities may also occur without a change in QRS configuration and reflect slow conduction without a change in the sequence of activation. Such slowing may be caused by cardioactive drugs, an increase in extracellular potassium concentration, and diffuse fibrosis or scarring as may occur in patients with severe cardiomyopathies.
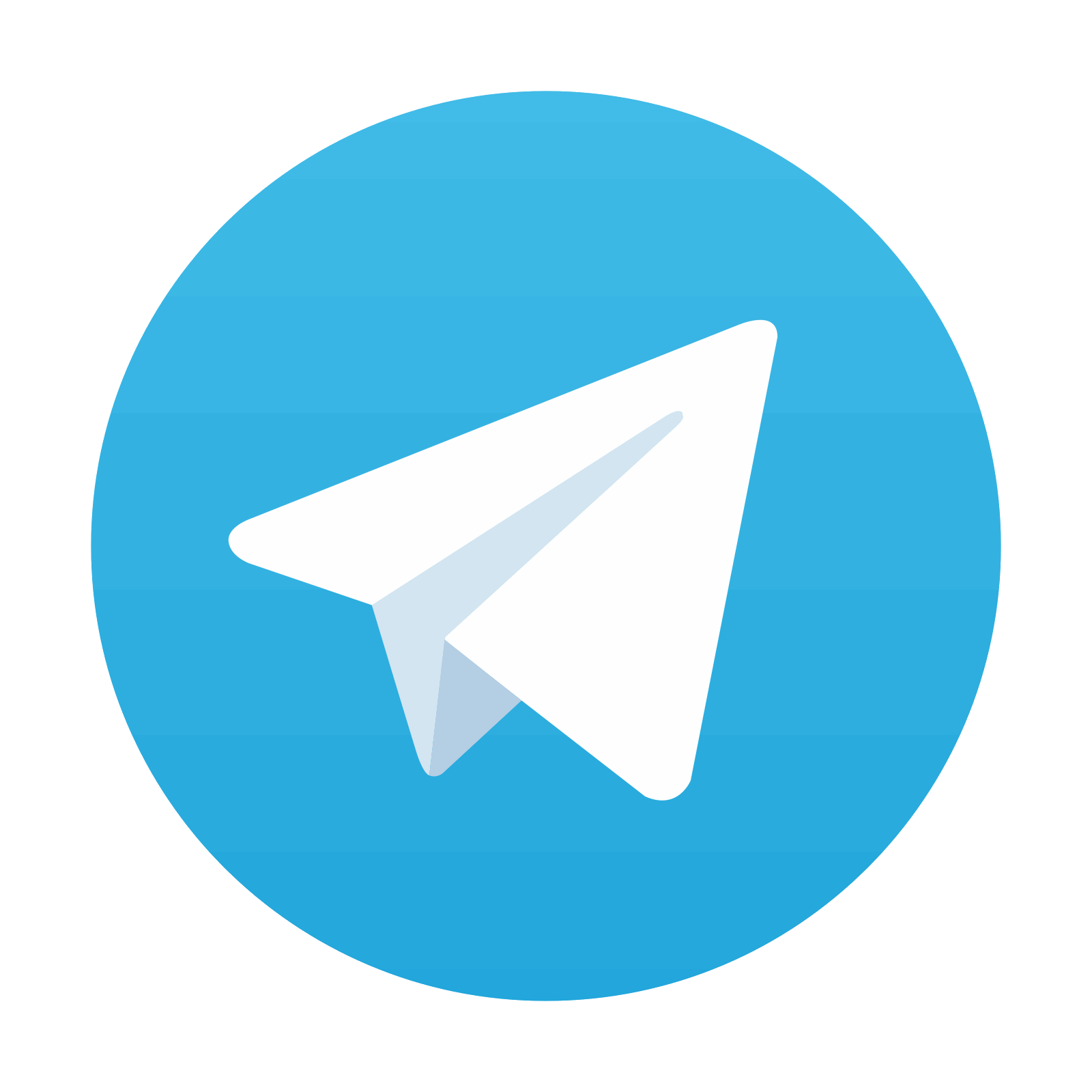
Stay updated, free articles. Join our Telegram channel

Full access? Get Clinical Tree
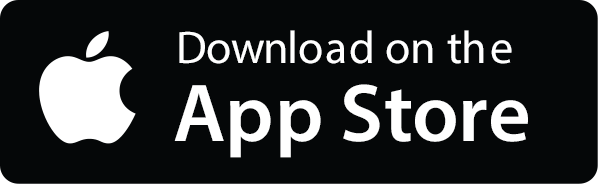
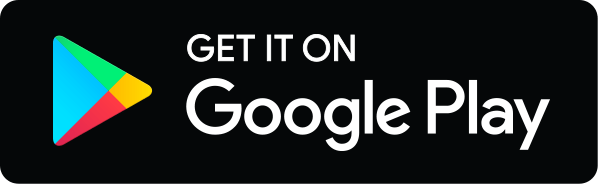