Electrocardiographic Exercise Testing
Zain Ul Abideen Asad
Chittur A. Sivaram
INTRODUCTION
Diagnosis of myocardial ischemia remains an important clinical challenge in patients presenting with chest pain in the ambulatory clinic. After the initial evaluation, stress testing is frequently employed in such patients to confirm the presence of ischemic heart disease. An array of tests, such as electrocardiographic (ECG) exercise testing, stress echocardiography, and myocardial perfusion imaging (MPI), are currently available to the clinician for this purpose. Although the cost, diagnostic accuracy, and complexities differ between various stress tests, unmasking of a mismatch between myocardial oxygen (O2) supply and myocardial O2 demand during stress remains the foundational principle for all the tests. Despite the growth of competing diagnostic tests, ECG exercise testing continues to have a robust, guideline-recommended role in the diagnosis of myocardial ischemia in carefully selected patients with suspected ischemic heart disease.1
INDICATIONS
ECG exercise testing is useful in patients with suspected ischemic heart disease for the confirmation of diagnosis as well as obtaining prognostic information. Diagnosis of myocardial ischemia is based on the appearance of significant ST segment deviation (depression or elevation) seen either during or immediately after termination of exercise. In order to maximize the diagnostic accuracy of ECG exercise testing, patients with intermediate pretest probability (15%-65%) for coronary artery disease and normal resting ECG are the most appropriate candidates for this test. Current guidelines also recommend exercise ECG stress test as the initial diagnostic test rather than other modalities of stress tests in patients with suspected ischemic heart disease and normal resting ECG.1
PHYSIOLOGIC CONSIDERATIONS
A basic understanding of exercise-related changes in cardiovascular physiology is essential for safe performance of exercise stress test, accurate interpretation of test results, and analysis of the implications of abnormalities observed.
Functional Capacity
During exercise, augmentation of cardiac output occurs, driven by an increase in heart rate (HR) and an increase in venous return and stroke volume through the Frank-Starling mechanism. In addition, increased O2 utilization by the exercising muscles results in an increase in the peripheral arteriovenous O2 difference. The O2 uptake value (VO2) is obtained by multiplying cardiac output by the arteriovenous O2 difference and serves as a reliable measure of the energy requirement of the body for a given amount of physical activity. The resting O2 requirement of 3.5 mL O2/kg body weight/minute is termed 1 metabolic equivalent (1 MET). Maximal O2 uptake (VO2 max) obtained during peak exercise is influenced by the patient’s age, sex, exercise conditioning, and other factors. Women have lower VO2 max compared to men, and VO2 max values progressively decline with aging, notably with a sharp decline after the 70s.2,3 The average VO2 max for healthy young men is approximately 12 METs and could be as high as 18 to 24 METs in long-distance runners.4
VO2 max is considered the best surrogate measure for fitness and functional capacity. This is due to the fact that arteriovenous O2 difference remains approximately constant at 15 to 17 mL/dL, and any increase in VO2 max during exercise will have to be attributed to changes in cardiac output. Cardiac output increases four to five times even with modest intensity of exercise because of a two- to threefold rise in HR; at continued higher intensity of exercise, the primary determinant of further augmentation in cardiac output is increased HR because the stroke volume plateaus at 50% to 60% of VO2 max.
Myocardial Oxygen Demand
The amount of O2 used by the myocardium, that is, myocardial O2 uptake, is determined by HR, contractility, and the left ventricular (LV) wall stress. The determinants of LV wall stress are LV end diastolic pressure and volume, wall thickness, and cavity size. Of these variables influencing myocardial O2 demand, HR and blood pressure (BP) remain the most practical and reliable parameters that could be monitored during exercise. Consequently, the product of HR and systolic BP, referred to as double product or rate pressure product, is commonly used to obtain an estimate of myocardial O2 consumption. The normal value of double product ranges from 25,000 (10th percentile) to 40,000 (90th percentile) during peak exercise.5 Higher values of double product correspond to improved myocardial perfusion. Double product has an important role in predicting cardiovascular prognosis.6,7,8
Myocardial Oxygen Supply
Normally, the myocardial O2 supply increases during exercise. This is driven largely by neurohormonal stimulation during exercise that releases vasodilators, such as nitric oxide, and leads to coronary vasodilation.9 Approximately a four- to fivefold augmentation of myocardial blood flow occurs during exercise as a result of coronary vasodilation. In patients with coronary artery disease, myocardial blood flow is compromised. A coronary stenosis of 50% to 70% owing to an atherosclerotic plaque will lead to impairment of this reactive hyperemia during exercise, and a 90% or more stenosis would impair myocardial blood flow even at rest. As a result of the reduced myocardial O2 supply in patients with atherosclerotic coronary artery disease, clinical symptoms of angina pectoris and various ECG changes can occur during exercise.
FUNDAMENTALS OF EXERCISE TESTING
Aerobic Exercise Effects on Cardiovascular Physiology
Heart Rate Response
A decrease in parasympathetic activity and an increase in sympathetic activity lead to a rise in HR immediately after aerobic exercise is started.10 There is a linear increase in HR with continued exercise. The most widely used equation for maximum predicted HR is 220 minus age in years for men and 80% of the above for women.11,12 Wide variability exists among subjects when the formula to predict maximum HR is used. Increase in HR rather than increase in stroke volume is responsible for a far greater augmentation in cardiac output during strenuous exercise. Stroke volume increases by about 50% with exercise, whereas the HR increase is more than 250%.13 Although a resting cardiac output is about 5.5 L/min, an increase of cardiac output up to 30 L/min can be seen in long-distance runners and other well-conditioned athletes. However, the behavior of HR response during exercise could be variable in patients. An exaggerated HR response to exercise is seen in deconditioning, anemia, or LV dysfunction, whereas an inadequate HR response to exercise (“chronotropic incompetence”) is seen with sinus node dysfunction. Multiple determinants, such as blood volume, peripheral vascular resistance, medications (beta-blockers), and body position, can also affect HR response to exercise.
HR response after termination of exercise can offer prognostic clues in heart disease. A delayed HR recovery, that is, a slow return of HR back to normal after exercise is terminated, suggests decreased vagal activity, an important predictor of mortality in patients with and without angiographic coronary artery disease.14,15,16 Despite some variability, most studies define an abnormal HR recovery as the inability to drop the HR by 12 to 21 bpm, 1 minute after the termination of exercise.17,18,19
Blood Pressure Response
Resistance (Isometric) Exercise Effects on Cardiovascular Physiology
There are clear differences between the cardiovascular physiology of aerobic exercise and resistance (isometric) exercise. The small increase in cardiac output occurring during resistance exercise is primarily mediated by an increase in HR. A decrease in venous return owing to increased intrathoracic pressure is common during resistance exercise. An increase in peripheral vascular resistance seen in resistance exercise limits increases in cardiac output. Increase in both systolic and diastolic BP occurs during resistance exercise.
The net result of both aerobic and resistance types of exercise is an increase in myocardial O2 demand because of increase in HR, BP, and LV wall tension.
CLINICAL APPLICATIONS
Electrocardiographic Exercise Stress Test Procedure
The protocol for exercise ECG stress test involves preprocedural assessment of the patient, recording of baseline ECG and vital signs (including pulse and BP), selection of the appropriate exercise protocol, performance of the test, and interpretation of test findings.
Patient Assessment Preprocedure
In preparation for the exercise ECG test, it is important to perform a thorough clinical assessment of the patient with emphasis on the presence of an appropriate indication and absence of any contraindications. Evaluation of preexisting symptoms particularly those attributable to relevant comorbidities is warranted.
A brief history focused on risk factors and symptoms leading to stress testing should be obtained from the patient and supplemented by chart review. Symptom review allows for identification of symptoms to monitor during the test, especially in patients who present with atypical symptoms of ischemia. Evaluation of a patient’s functional capacity is important for the appropriate selection of exercise protocol, reducing the risk of physical injuries during exercise, and improving the overall safety of the test. Assessment of pretest probability of ischemic heart disease is essential for confirming the appropriateness of ECG exercise testing rather than another type of stress modality. The ideal candidate for ECG exercise testing has a predicted intermediate pretest probability (15%-65%) for ischemic heart disease test, based on the type of chest pain (typical angina, atypical angina, or noncardiac chest pain) and sex of the patient.1 False-positive exercise ECG tests are frequent in those with lower pretest probability, and false-negative tests are of concern in those with higher than 65% pretest probability. Physical examination should be performed with particular attention to the presence of murmurs and orthopedic or neurologic conditions that limit physical activity. Precordial murmurs including late peaking aortic systolic murmurs
should warrant echocardiography to exclude severe aortic stenosis prior to the test. Exercise ECG test is of high value for the diagnosis of ischemia only when the resting ECG is normal.1 In the presence of ST depression, LV hypertrophy, left bundle branch block (LBBB), pre-excitation, or continuously paced rhythm on the resting ECG, exercise ECG becomes uninterpretable for the presence of ischemia.
should warrant echocardiography to exclude severe aortic stenosis prior to the test. Exercise ECG test is of high value for the diagnosis of ischemia only when the resting ECG is normal.1 In the presence of ST depression, LV hypertrophy, left bundle branch block (LBBB), pre-excitation, or continuously paced rhythm on the resting ECG, exercise ECG becomes uninterpretable for the presence of ischemia.
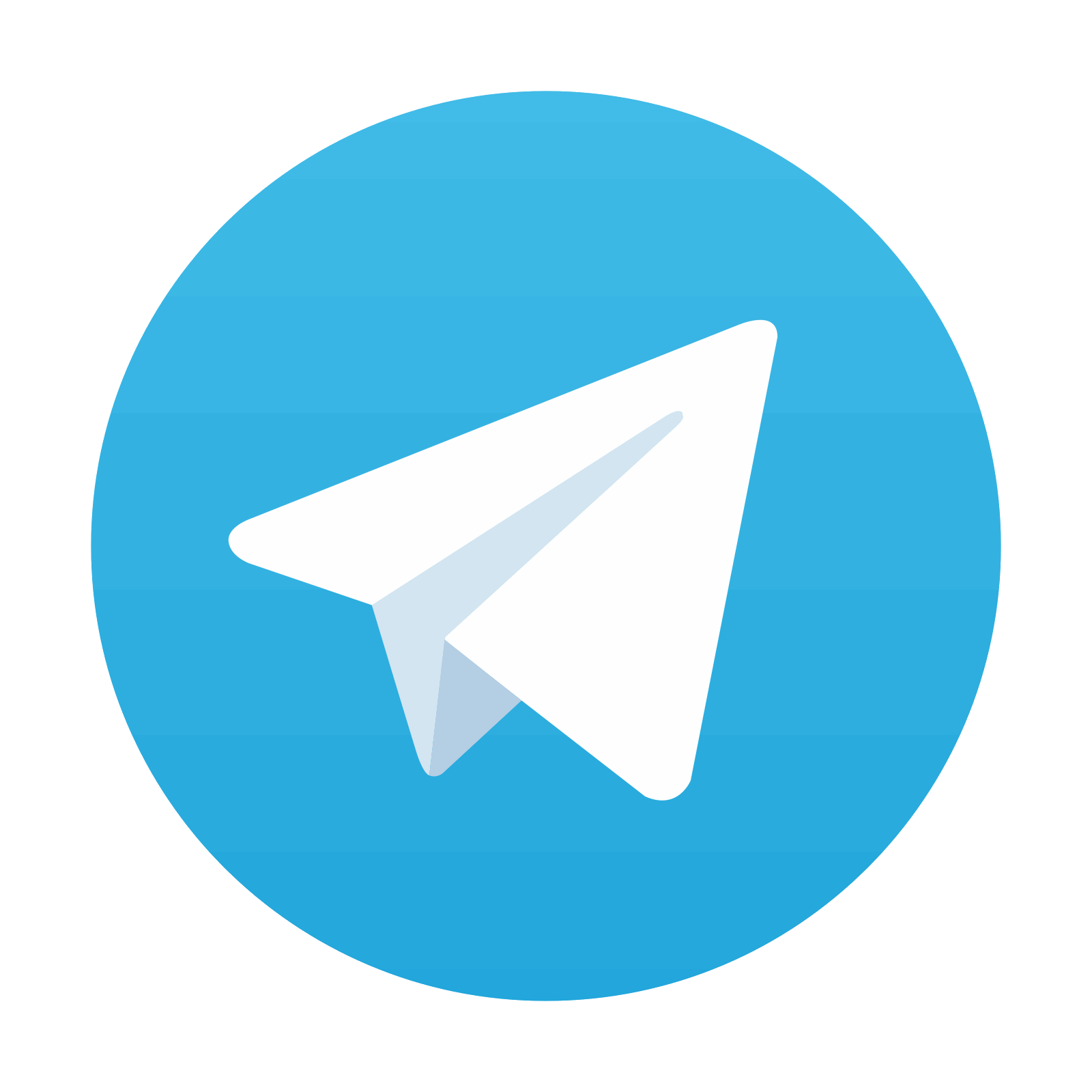
Stay updated, free articles. Join our Telegram channel

Full access? Get Clinical Tree
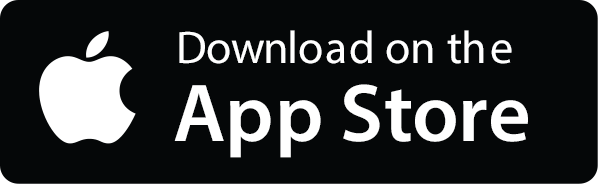
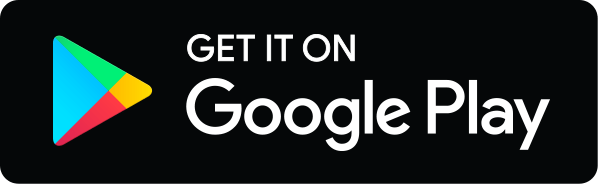
