Electrocardiographic (ECG) changes resulting from drainage of pericardial effusion (PE) have not been systematically studied. In addition, because PE and peripheral edema produce voltage attenuation of the electrocardiogram, we aimed at exploring whether these 2 conditions could be differentiated by electrocardiogram. We previously showed that peripheral edema attenuates amplitudes of QRS complexes and P waves and published a case report showing that attenuation in PE involves QRS complexes but not P waves. Clinical, laboratory, and echocardiographic data and quantitative measurements of amplitudes of QRS complexes and P waves before and after pericardiocentesis in 43 patients were obtained. Although changes of QRS complexes before and after pericardiocentesis were statistically significant, they were not so for P waves. Other ECG measurements before and after pericardiocentesis did not show statistically significant changes, except for heart rate, which decreased, and PR interval and QRS duration, which increased after the procedure. In conclusion, pericardiocentesis results in augmentation of QRS complexes on electrocardiogram, whereas it does not affect amplitude of P waves; this differential from peripheral edema is attributed to the fact that PE around the atria is much less than around the ventricles, and thus alleviation of PE alters the voltage transfer dynamics to the body surface for the atria much less than it does for the ventricles.
There is no literature on 12-lead electrocardiographic (ECG) changes resulting from pericardial effusion (PE) drainage, although intuitively augmentation of ECG voltage is anticipated. The PE/ECG interaction in PE is complex, but the focus is ECG changes after PE drainage. Peripheral edema, like PE, is associated with attenuated ECG voltage, and its management leads to an augmentation of ECG voltage. Anatomy of the pericardial sac limits the size of PE behind the atria, and thus the question arises as to whether there is less augmentation of ECG voltage of P waves than of QRS complexes with drainage of PE, as shown in a limited experience. In contrast, peripheral edema alleviation leads to a uniform augmentation of ECG voltage of QRS voltage (AQRSV) and P-wave voltage, probably because fluid is an all-permeating body volume conductor, affecting all 4 cardiac chambers equally. An opportunity arose to investigate these issues conclusively, employing the database of a recently published study.
Methods
The database of the present communication includes 43 patients, and the study design is retrospective and prospective. These 43 patients comprise a subgroup of 110 patients who presented from January 2000 to June 2009 with dyspnea resulting from significant PE and had echocardiographically guided pericardiocentesis, with electrocardiograms recorded before and after the procedure; all 43 patients had 2-dimensional echocardiogram obtained before and after the procedure. Data on 34 of these 43 patients had been previously published and were analyzed retrospectively for the purposes of this study. Seven additional patients from the first and 2 from the second collaborating institution were studied prospectively and added to the series. After receiving consent forms, pericardiocentesis was performed under local anesthesia at the bedside, employing echocardiograms for determination of the optimal puncture site and direction of the needle. Biochemical, serologic, and cytologic evaluations of drained fluid were done to determine the cause of PE. The 21 patients with metastatic malignancies had stage IV disease proved by positive cytologic analysis of PE fluid.
Electrocardiograms were obtained before and after pericardiocentesis and constituted paper hardcopies recorded at 25 mm/s and at 10 mm equal to 1.0-mV standardization. Paper hardcopies were mailed, and scans of hardcopies were electronically mailed to 1 of the authors (JEM) who performed manual measurements (described later) being blinded to the identity of patients, sequence of electrocardiograms in reference to pericardiocentesis, and with the upper part of the ECG hardcopy, where automated measurements and diagnoses were included, obliterated. In essence JEM received a “jumbled” stack of ECG tracings, and an electronic file containing 82 electrocardiograms of the 41 patients bearing only a number (numbers 1 to 82). For the 2 patients studied in Elmhurst Hospital Center (Elmhurst, New York), and because this author knew the identity of the patients and the sequence of their electrocardiograms in connection to pericardiocentesis, no manual measurements were performed but automated measurements generated by the electrocardiograph’s algorithm were used instead. Electrocardiograms were analyzed on the computer monitor with 8× magnification and on paper hardcopies using a magnifying glass with similar magnification under strong overhead light, and the most satisfactory version was chosen for measuring peak-to-bottom amplitudes of QRS complexes and P waves in all 12 ECG leads, in millimeters, to the nearest 0.25 mm. Variables used in analyses included amplitudes of QRS complexes and P waves in leads II, V 1 , I, aVF, and V 2 , calculated spatial vector magnitude using the sum of leads I, aVF, and V 2 (Vm), and sums of all 12 ECG leads (sum of QRS amplitude in 12 ECG leads and sum of P-wave amplitude in 12 ECG leads) of electrocardiograms before and after pericardiocentesis; in addition, automated values of heart rate, PR interval, QRS duration, QT and QTc intervals, P-wave axis, QRS axis, and T-wave axis and percent changes of these were employed as variables. The objective for using leads I, aVF, and V 2 was to provide the equivalent of the 3 orthogonal ECG leads, used often in ECG studies, or in recording 3-dimensional vectorcardiogram.
Comprehensive echocardiography was performed using commercially available ultrasound systems. Standard M-mode and 2-dimensional echocardiographic measurements were taken according to guidelines of the American Society of Echocardiography. Left ventricular ejection fraction was measured by the biplane modified Simpson method from apical 4- and 2-chamber views. Left atrial diameter, left ventricular end-diastolic, left ventricular end-systolic, and right ventricular end-diastolic dimensions were measured by M-mode echocardiography. Echocardiography was also performed for the diagnosis, localization, and quantification of PE, which was defined as an echocardiographic lucent separation between the parietal and visceral pericardia. PE was graded as small, moderate, or large based on the greatest width of the echocardiographic free space and the circumference of effusion to the entire heart on the parasternal short-axis view of echocardiogram. A width <10 mm and/or noncircumferential distribution was graded as small, 10 to 20 mm with circumferential distribution as moderate, and >20 mm with circumferential distribution as large. Moderate and large PEs were considered significant.
Statistical analyses were conducted using SPSS 12.0 (SPSS, Inc., Chicago, Illinois). Continuous variables are presented as means ± SDs and frequency percentages, and their values from before and after pericardiocentesis were compared by paired t test. Percent change (Δ%) of a variable was correlated employing Pearson correlation coefficient. A p value <0.05 was considered statistically significant.
Results
Demographics and causes of PE are listed in Table 1 , as are intervals between ECG recordings and pericardiocentesis, blood pressure, and incidence of atrial fibrillation. Figures 1 and 2 depict typical examples of changes noted on echocardiogram and 12-lead electrocardiograms before and after pericardiocentesis. Echocardiographic free space of PE was larger posteriorly than anteriorly in all 43 patients, and PE consisted of exudate in 41 patients and blood in 2 patients. Echocardiographic measurements, pertaining to time before drainage of PE, left ventricular ejection fraction, and incidence of tamponade and valvular heart disease are listed in Table 1 .
Variable | (n = 43) |
---|---|
Age (years) | 58.1 ± 17.1 |
Men | 25 (58.1%) |
Height (cm) | 164.7 ± 8.7 |
Weight (kg) | 59.9 ± 9.2 |
Underlying causes of pericardial effusion | |
Lung cancer | 11 (25.6%) |
Breast cancer | 5 (11.6%) |
Stomach cancer | 4 (9.3%) |
Pulmonary tuberculosis | 5 (11.5%) |
Postoperative bleeding | 2 (4.7%) |
Uremia | 3 (7.0%) |
Connective tissue diseases | 3 (7.0%) |
Myopericarditis | 3 (7.0%) |
Others (mesothelioma, metastasis of unknown origin, amyloidosis, acquired immunodeficiency syndrome, after myocardial infarction) | 5 (11.6%) |
Unknown | 2 (4.7%) |
2-Dimensional echocardiographic findings | |
Interventricular septum thickness (mm) | 11.3 ± 2.4 |
Posterior wall thickness (mm) | 10.9 ± 2.2 |
Left ventricular end-diastolic diameter (mm) | 43.8 ± 7.2 |
Left ventricular end-systolic diameter (mm) | 27.5 ± 8.3 |
Left atrial diameter (mm) | 34.4 ± 4.1 |
Right ventricular end-diastolic diameter (mm) | 18.5 ± 8.7 |
Left ventricular ejection fraction (%) | 56.3 ± 11.5 |
Greatest width of echocardiographic free space at end-diastole (mm) | 19.1 ± 8.3 |
Greatest width of echocardiographic free space at end-systole (mm) | 26.1 ± 9.1 |
Mitral valve disease | 1 (2.3%) |
Aortic valve disease | 3 (7.0%) |
Atrial fibrillation | 6 (14.0%) |
Findings in pericardiocentesis | |
Amount of effusion drained (ml) | 778.8 ± 308.7 |
Time from before electrocardiography to pericardiocentesis (hours) | 17.2 ± 22.2 |
Time from pericardiocentesis to postprocedure electrocardiography (hours) | 17.5 ± 26.1 |
Systolic blood pressure (mm Hg) | 105.4 ± 23.1 |
Diastolic blood pressure (mm Hg) | 67.6 ± 16.3 |
Heart rate (beats/min) | 93.6 ± 22.1 |
Cardiac tamponade | 7 (16.3%) |
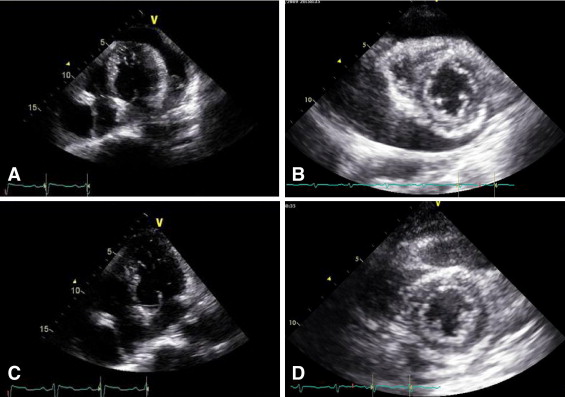
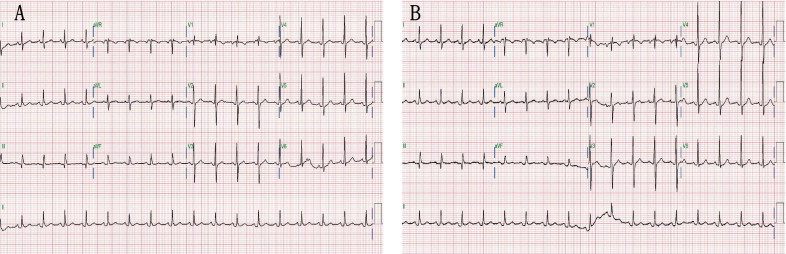
QRS complex and P-wave variables before and after pericardiocentesis are listed in Tables 2 and 3 . All changes for QRS complex were statistically significant, except for those of lead aVF; however, none of the changes for P wave were significant, except for those in lead V 2 ( Table 2 ). Other ECG measurements before and after pericardiocentesis did not reveal statistically significant changes, except for heart rate, which decreased, and PR interval and QRS duration, which increased after the procedure ( Table 3 ).
ECG Leads | Before | After | Patients | p Value | Δ% |
---|---|---|---|---|---|
QRS complex | |||||
Lead II | 6.30 ± 2.76 | 6.95 ± 2.81 | 42 | 0.016 | 15.36 ± 30.84 |
Lead V 1 | 7.42 ± 3.24 | 8.71 ± 4.70 | 43 | 0.022 | 28.74 ± 59.00 |
Lead I | 5.14 ± 2.17 | 6.19 ± 2.77 | 42 | 0.005 | 33.85 ± 64.51 |
Lead aVF | 5.10 ± 2.28 | 5.14 ± 2.69 | 43 | 0.866 | 6.73 ± 36.43 |
Lead V 2 | 14.34 ± 6.79 | 16.60 ± 7.30 | 43 | 0.011 | 20.93 ± 54.19 |
Sum of leads I, aVF, and V 2 | 16.73 ± 7.36 | 18.94 ± 7.11 | 42 | 0.010 | 21.29 ± 43.08 |
Sum of QRS-complex amplitudes in 12 leads | 99.42 ± 34.98 | 114.93 ± 43.63 | 42 | 0.002 | 17.97 ± 32.07 |
P wave | |||||
Lead II | 1.33 ± 0.50 | 1.40 ± 0.53 | 36 | 0.385 | 10.52 ± 44.26 |
Lead V 1 | 0.98 ± 0.39 | 1.13 ± 0.52 | 37 | 0.069 | 29.77 ± 73.46 |
Lead I | 1.00 ± 0.42 | 1.06 ± 0.43 | 36 | 0.336 | 11.59 ± 36.96 |
Lead aVF | 1.00 ± 0.38 | 0.91 ± 0.46 | 37 | 0.283 | 1.87 ± 57.34 |
Lead V 2 | 0.71 ± 0.39 | 0.88 ± 0.42 | 37 | 0.014 | 46.86 ± 83.41 |
Sum of leads I, aVF, and V 2 | 1.68 ± 0.46 | 1.73 ± 0.56 | 36 | 0.572 | 6.74 ± 35.94 |
Sum of P-wave amplitudes in 12 leads | 10.43 ± 3.19 | 11.00 ± 3.40 | 36 | 0.213 | 8.83 ± 28.38 |
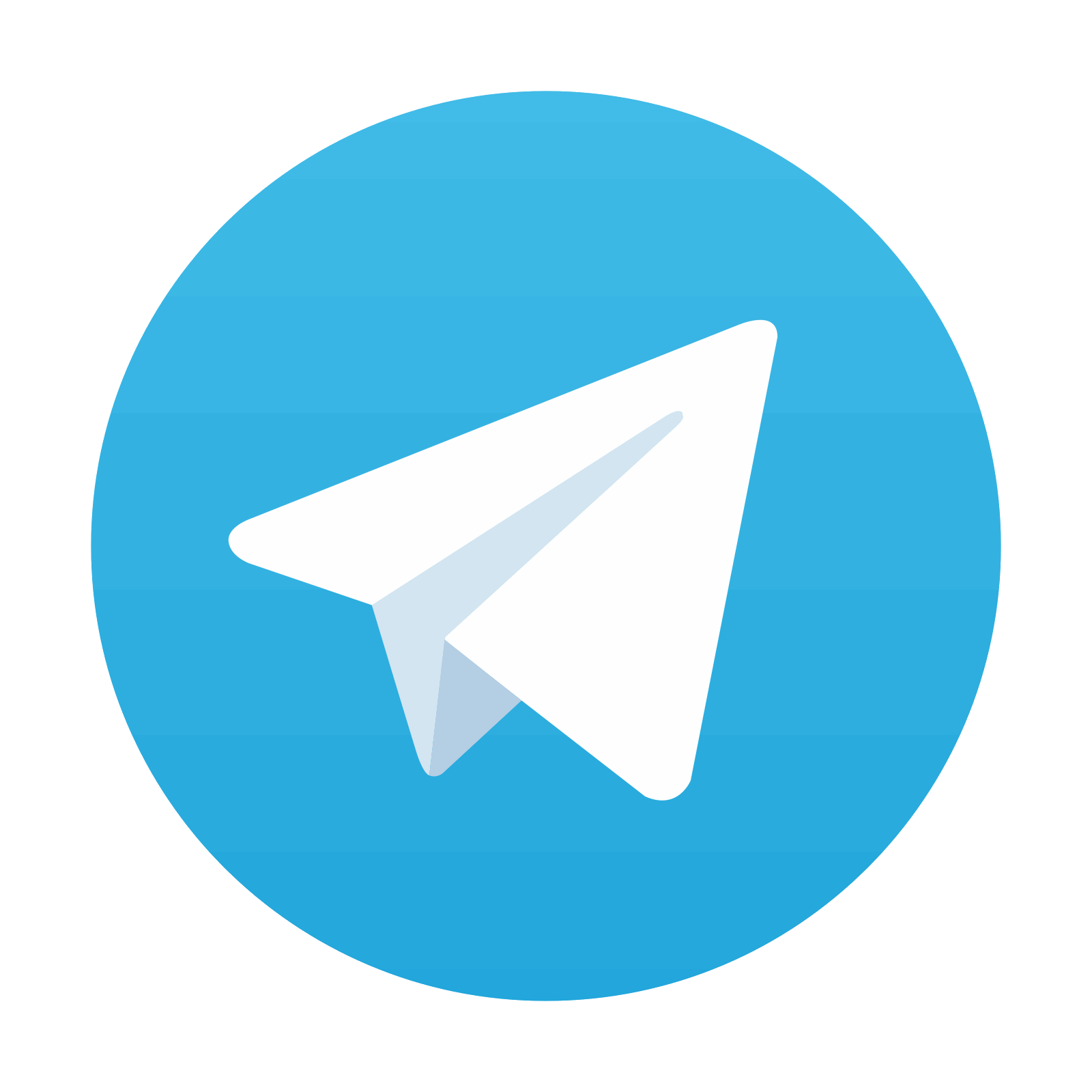
Stay updated, free articles. Join our Telegram channel

Full access? Get Clinical Tree
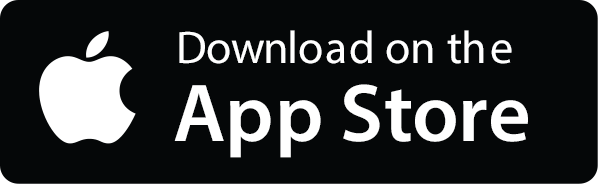
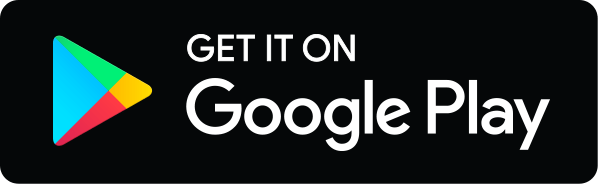
