Electroanatomic Mapping Systems
Anshul M. Patel
Vivek Y. Reddy
Catheter ablation of atrial fibrillation (AF) is dependent on a firm understanding of left atrial (LA) and pulmonary venous (PV) anatomy. There have been a number of different ablation strategies described, but common to all is the need for accurate navigation in the LAPVs. The most common methods of AF ablation focus on (a) isolating the triggers typically located in the PVs and other venous structures, and (b) substrate modification in an attempt to eliminate the ability of the atria to sustain AF.
Conventional fluoroscopy has the advantage of being open-architecture in its ability to visualize catheters—that is, any conventional catheter can be seen by x-ray. In addition, all catheters are visualized in real time. However, fluoroscopy-based catheter mapping also has important limitations. In addition to exposing the patient, operator, and lab staff to ionizing radiation, one can only see a 2-D representation of the anatomy for any given image. The 3-D anatomy of the chamber can be difficult to perceive. To this end, the advent of computer-based electroanatomical mapping (EAM) systems has greatly facilitated ablation of arrhythmias in the face of complex cardiac anatomy. By better assuring that lesions are delivered where intended, EAM systems have the potential to make the procedure easier to perform with less risk of complications.
Fundamentally, EAM systems serve three important functions:
A 3-D anatomical rendering of the relevant cardiac chambers is generated by creating a spatial catalogue of point locations.
Catheters are localized in 3-D in a nonfluoroscopic fashion.
Various types of electrical information are collected and can be projected onto the anatomy to facilitate an understanding of the arrhythmia.
This chapter focuses on the two most commonly used EAM systems in practice today. To localize catheters within the heart, the first uses a magnetic field (magnetic electroanatomic mapping [MEAM]) and the second uses electrical impedance fields (electrical impedance electroanatomic mapping [EiEAM]).

The MEAM system (CARTO, Biosense-Webster, Inc, Diamond Bar, CA) is one of the most widely used EAM systems for ablation. It is based on the premise that a coil placed in a magnetic field will generate an electrical current. The magnitude of the current depends on the field’s strength and orientation of the coil within the field. A processing unit gathers data to determine the location of the catheter tip and its location (Fig. 6.1) (1).
A locator pad is positioned beneath the procedure table and generates three ultralow magnetic fields (5 × 10−6 to 5 × 10−5 Tesla), which code the mapping space around the patient’s chest. These fields contain the information to determine the location and orientation of the sensor to six degrees of freedom (x, y, z, roll, pitch, and yaw). Each coil of the locator pad generates a magnetic field that decays as a function of the distance from that coil. The sensor measures the strength of the magnetic field, thus enabling determination of the distance from each of its sources. These distances become the radii of theoretical spheres around each coil. The intersection of these three spheres is the location of the sensor in space (see Fig. 6.1) (1).
The system requires the use of a proprietary deflectable catheter (Navistar, Biosense-Webster, Inc, Diamond Bar, CA), which comes in variety of sizes (3.5 mm, 4 mm, 8 mm) and in standard solid tip and saline irrigated. The catheter has a locator sensor embedded in its tip that sends signals to the processing unit. Using a point-by-point mapping approach, a detailed 3-D electroanatomic map can be created. Various electrical information is annotated to each of these points and can be displayed in a color code to facilitate arrhythmia interpretation. Examples include local activation timing in relation to a reference electrogram (e.g., the surface QRS or coronary sinus electrogram) and electrogram voltage amplitude maps.
Patient Movement
A back patch is used as a point of spatial reference. Because the navigable magnetic field generated by the system has a limited size, it is critical that the patch be placed to optimize magnetic navigation to every location within the LA-PVs. For the LA-PVs, the patch should be placed on the patient’s back in a paraspinal location at the level of the bottom of the left shoulder blade. In dilated and rotated hearts, these surface landmarks may not always accurately represent the LA-PV position. Ideally, the position of the back patch should be checked under fluoroscopy to ensure that it is behind the chamber of interest.
The back patch is used as a fixed point of reference to minimize fluctuations in the magnetic field and prevent movements of the magnet substantially altering the fidelity of the EAM system. For example, if the magnet is hit by the fluoroscopic C-arm and the magnet moves relative to the patient, it can simply be moved back into place on the MEAM system. However, if the patient moves and the back patch moves relative to the patient (e.g., due to skin laxity), then the map will be improperly referenced. By the same token, it is feasible to move the magnet when a particular location is outside the mappable area to allow catheter detection without worrying that the map is no longer accurate. The only way to confirm that the patch has not moved relative to the patient is to move the catheter to “familiar” locations (posterior LA, roof, inferior, PVs, etc.) and confirm that the MEAM location is the same as the fluoroscopic location.
Respiration
The catheter is also significantly affected by respiration. Because there is no gating of the image based on respiration, it is incumbent on the operator to acquire points at the same point in the respiratory cycle. During inspiration, one can visualize the characteristic inferior and anterior motion of the catheter tip. By following the catheter position, it is fairly straightforward to recognize and manually gate EAM point acquisition to respiration. The tidal volumes that patients take can vary widely so timing to end inspiration is not optimal. On the other hand, timing to end expiration, which most reproducibly represents the level of the functional residual volume, provides the most spatially accurate maps.
Electrogram Reference Timing and Activation Timing Window
Points acquired during MEAM are annotated with timing information stored as the difference in milliseconds of the electrogram at the tip of the mapping catheter in relation to a reference electrogram chosen by the operator. For AF ablation, there is no fixed reference electrogram because both atrial and ventricular electrograms can have fluctuating cycle lengths. Since AF ablation is largely an anatomically based procedure, electrical timing is not as important as spatial referencing of the catheter tip at each atrial location. The ventricular cycle is the major determinant of catheter location; accordingly, using the surface QRS complex for electrical timing results in temporal gating to the end of ventricular diastole and maximizes the likelihood of beatto-beat spatial stability. On the other hand, gating to an atrial electrogram during AF would result in greater beat-to-beat spatial instability since the atrial electrogram may fall during any phase of the ventricular cycle.
On the other hand, during post-AF ablation atrial tachycardias and atrial flutters, electrogram reference timing becomes critical. It is best to set the activation timing window to a value that encompasses the cycle length of the tachycardia. For example, if the atrial flutter has an atrial cycle length of 240 ms, one would set the timing window to −120 ms to +120 ms. The reference electrogram is also important to create an activation map. The QRS complex on a surface lead or local V electrogram is
less useful in these reentrant or focal arrhythmias because the ventricular response is often variable. It is best to use an atrial electrogram, often using a stable catheter location such as the coronary sinus (CS) catheter. Storing a fluoroscopic image of the CS catheter position is also helpful in case it is dislodged during mapping. When choosing the atrial electrogram reference, it is also important to choose one with a clear and stable deflection (i.e., not fractionated so that judging the beginning of the electrogram is not difficult). It is also critical to choose an atrial electrogram that is in a stable position in the R-R interval as a way to localize the desired electrogram on the reference electrode. Otherwise, it may be challenging to know that one has chosen the same reference electrogram.
less useful in these reentrant or focal arrhythmias because the ventricular response is often variable. It is best to use an atrial electrogram, often using a stable catheter location such as the coronary sinus (CS) catheter. Storing a fluoroscopic image of the CS catheter position is also helpful in case it is dislodged during mapping. When choosing the atrial electrogram reference, it is also important to choose one with a clear and stable deflection (i.e., not fractionated so that judging the beginning of the electrogram is not difficult). It is also critical to choose an atrial electrogram that is in a stable position in the R-R interval as a way to localize the desired electrogram on the reference electrode. Otherwise, it may be challenging to know that one has chosen the same reference electrogram.
Focal or microreentrant atrial tachycardias will usually be color coded with the earliest activation occurring at the center of a spread of colors in an omnidirectional fashion. It is best to target these types of arrhythmias at the center of such focal spread. Macroreentrant atrial arrhythmias will have a color-coded pattern where earliest activation meets latest activation. Because these are reentrant rhythms, there is no true earliest or latest activation. In these cases, the ideal ablation pattern should be across a narrow isthmus, preferably between two areas of scar, not necessarily where the mapping has pointed to the place of “earliest activation.”
Volume Status
AF ablation procedure durations can be long and volume shifts can significantly alter the left atrial anatomy. In the authors’ experience, the anterior portion of the left atrium closer to the mitral annulus appears to be the most affected. Heart rate increases either intrinsic to the patient or due to isoproterenol infusion can significantly decrease the size of the chamber. Also, externally irrigated ablation catheters can infuse a great deal of volume: If irrigating at 30 cc/min during ablation, 100 ablation lesions lasting 1 minute each (a realistic amount of radiofrequency energy for an AF ablation procedure) would result in the infusion of 3 liters of saline into the patient. On the other hand, the use of general anesthesia could result in significant vasodilation and decreased left atrial chamber size.
Image Integration
A point-by-point acquisition process to create a 3-D anatomic map is time consuming and can be prone to error. Advances in computerized tomography (CT) and magnetic resonance (MR) imaging can now generate detailed 3-D reconstructions of the LAPVs. The images can be used side by side with EAM maps as a visual aid. But most useful, it is now possible to directly integrate these 3-D images with EAM data to guide real-time catheter ablation for AF within the highly featured CT/MR anatomy (2,3). It also may improve the safety and efficacy of the procedure (4).
We have shown in a series of in vitro and in vivo experiments the feasibility of integrating MRI and electroanatomic mapping data (5,6). The greatest utility of this strategy is to provide guidance for real-time catheter manipulation quickly and more accurately than MEAM alone. Effective registration requires a properly acquired CT or MR image. The images should extend from above the arch of the aorta to just below the diaphragm. The images should be acquired at end expiration at the level of the functional residual volume rather than the end-inspiratory state often used by radiologists. This prevents the characteristic superior-inferior displacement of the left atrium and the splaying-narrowing of the PVs with respiration (7).
Software-based thresholding, cutting, and region-growing tools are used to segment the vessels and cardiac chambers on a regional basis. These chamber subregions are then connected or deleted as appropriate to assemble 3-D renderings of the LA
(containing at least a suitable proximal segment of each of the PVs) and the entire thoracic aorta. Although any structure within the body can be used for the registration process, we have found that employing the aorta for registration is the quickest and most reliable strategy. In our experience, a number of other structures were assessed but found to be suboptimal. For example, the superior vena cava and coronary sinus are relatively thin-walled structures that can easily be deformed with catheters making them less reliable and more time consuming to use. The pulmonary artery is less deformable, but technically more complex to fully map. The only disadvantage with aorta registration is the requirement for a femoral arterial puncture.
(containing at least a suitable proximal segment of each of the PVs) and the entire thoracic aorta. Although any structure within the body can be used for the registration process, we have found that employing the aorta for registration is the quickest and most reliable strategy. In our experience, a number of other structures were assessed but found to be suboptimal. For example, the superior vena cava and coronary sinus are relatively thin-walled structures that can easily be deformed with catheters making them less reliable and more time consuming to use. The pulmonary artery is less deformable, but technically more complex to fully map. The only disadvantage with aorta registration is the requirement for a femoral arterial puncture.
When using the aorta, endoluminal contact EAM points are acquired in the descending aorta and aortic arch to fix the map in both the long and short axes (Fig. 6.2A). In the arch, it is important to acquire points along both the bottom and top of the arch to fix it vertically as well as points contacting the left and right walls of the arch of the aorta to properly fix rotational movement. One should not acquire EAM points outside the boundaries of the CT/MR image, because these points will reduce the accuracy of the registration. After acquiring points in the aorta and registering to the corresponding CT/MR image, the EAM and CT/MR coordinate systems are now linked (Fig. 6.2B). Accordingly, when the catheter is placed into the left atrium, a good level of registration already exists. Any LA-PV registration errors encountered at this point are largely due to the respiratory state during CT/MR imaging—which affects the relative position of the LA-PVs to the aorta.
Additional points are then acquired in the LA-PVs (20 to 40 points) superiorly, inferiorly, and on the posterior wall. A few points on the anterior wall are useful, but since the anterior wall is the structure most sensitive to volume-related anatomical difference, our preference is to minimize the number of points acquired at this location (Fig. 6.3). Points are taken at 5 to 10 contact locations in each pulmonary vein. The “tube pullback” function for rapid acquisition of points can be used, but should be done only after asking the patient to hold breathing at end expiration. Alternatively, standard EAM points can be acquired in the PVs. In any event, the catheter should be touching the vein wall, which may require some torque. During left superior PV mapping, the catheter is undeflected superiorly so it touches the superior aspect of the vein; for the left inferior PV, the catheter is torqued so it touches the posterior aspect of the vein. For the right superior PV, the catheter is torqued anteriorly, and for the right inferior PV, it is torqued to just touch the posterior wall. However, too much torque might deflect the thin walls of the vein, causing anatomic distortion. The LA can then be registered, using the EAM points from all of these structures.
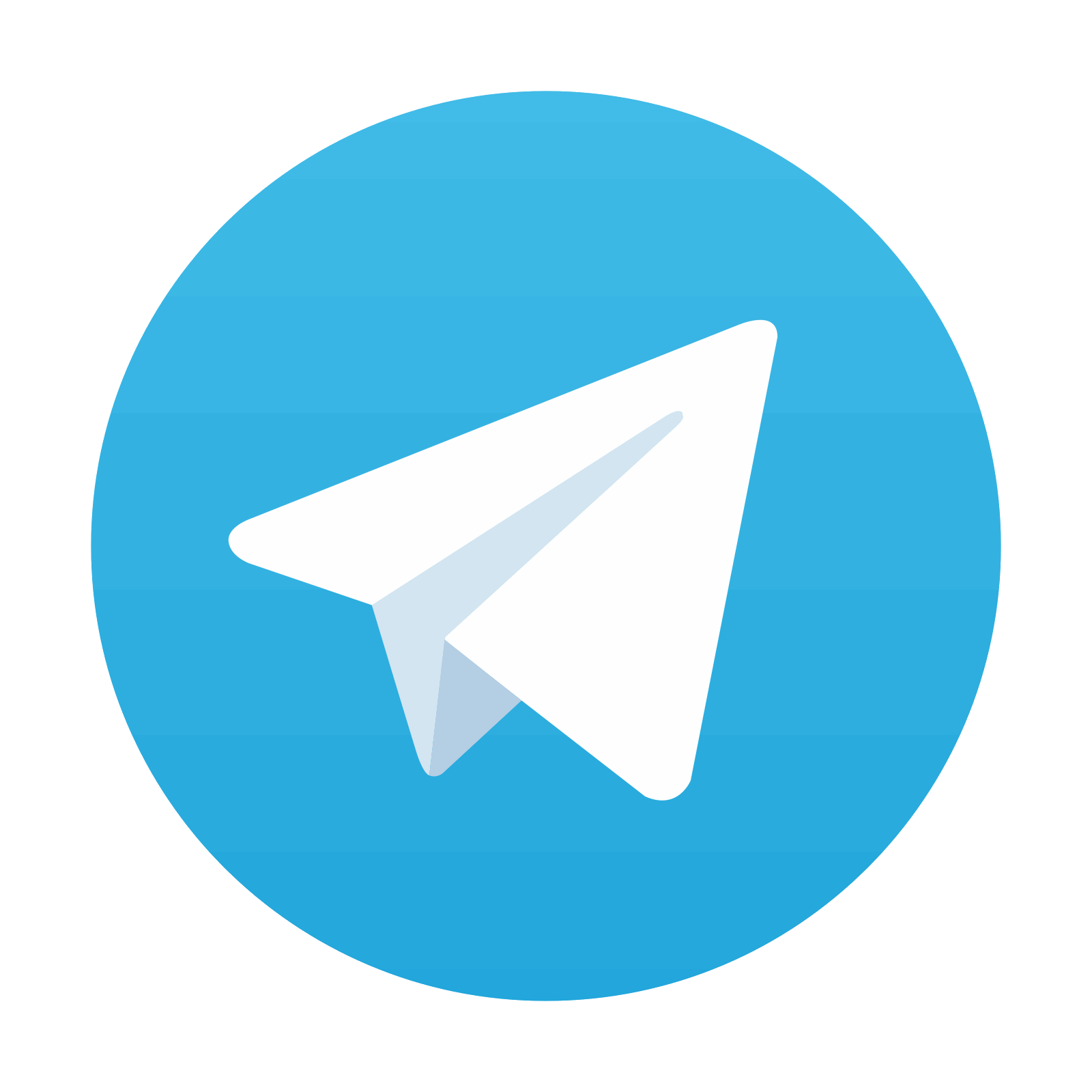
Stay updated, free articles. Join our Telegram channel

Full access? Get Clinical Tree
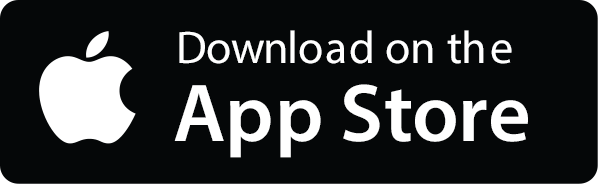
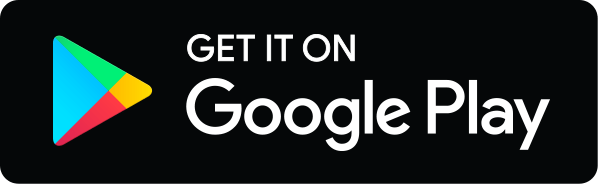