26
Effects of travel or work at high altitudes or low pressures
Exposure to high altitude implies exposure to low ambient pressure as a consequence of the physics of the atmosphere. The exposure can occur gradually, as in mountaineering or high hill walking, or relatively quickly, as in ascent by aeroplane or balloon.
Exposure to gradual terrestrial ascent above about 3000 m puts the susceptible individual at risk of developing altitude illness, a collective term encompassing the major conditions caused directly by hypobaric hypoxia. These include acute mountain sickness and high-altitude pulmonary edema.
Rapid ascent in the atmosphere above about 3000 m on the other hand, as may occur in an aeroplane, gives rise to hypoxic hypoxia (the term given to hypoxia due to insufficient oxygen reaching the blood and a low partial pressure of oxygen in arterial blood), to which all individuals are susceptible. The clinical features of the acute phases of altitude illness are different from those observed in aviation-related hypoxic hypoxia, with differences in diagnosis and treatment.
A high incidence of acute mountain sickness has been reported in tourists flying to high-altitude cities such as Lhasa (3658 m), Leh (3514 m), La Paz (3625 m) and Cuzco (3415 m), so a simple distinction between mountain sickness and aviation hypoxia is not necessarily straightforward.
Occupations exposed to high altitude include mountaineers and mountain guides, with their associated professions, and professional aircrew and frequent business flyers.
26.2 Physics of the high-altitude environment
At sea level the atmosphere exerts a pressure of about 760 mm Hg (101 kPa); it is variably moist, has a temperature that ranges from -60 to +60°C, and moves at wind speeds from 0 to 160 km/h. With increasing altitude, the temperature, pressure and water content of the atmosphere fall and wind speeds increase.
Total gas pressure falls with altitude in a regular manner, halving every 18,000 ft (5500 m; it remains the convention in aviation to use ft for altitude). The oxygen percentage of the atmosphere (20.93%) is constant to very high altitudes, so the same curve can be used to obtain the ambient oxygen pressure by rescaling the ordinate. The oxygen pressure of physiological importance is that which exists in ambient air when it is warmed and wetted on entering the bronchial tree. This raises water vapor pressure to about 47 mm Hg, regardless of the total gas pressure outside. The oxygen pressure in moist inspired gas (PiO2) fully saturated with water vapor at 37°C is given by the relationship:
where PB is barometric pressure and FiO2, the fractional concentration of oxygen in the inspirate, is 0.2093.
26.2.2 Atmospheric temperature
The atmospheric temperature falls at a rate of 1.98°C/1000 ft (300 m) from the standard sea level temperature of 15°C, to the tropopause [the border between the lower level, the troposphere, and the higher level or stratosphere; 40,000 ft (12,200 m)]. It remains stable at 56°C up to about 80,000 ft (24,400 m) and then rises to almost body temperature at about 150,000 ft (46,000 m), but by then air density is so low that its temperature is unimportant.
Atmospheric ozone at high altitude is formed by ultraviolet irradiation of diatomic oxygen molecules which dissociate into atoms. At very high altitudes all oxygen exists in the monatomic form. Lower down, some of this monatomic oxygen combines with oxygen molecules to form the triatomic gas ozone, with concentrations up to 10 ppm. The ozonosphere normally exists between 40,000 and 140,000 ft (12,200 and 42,700 m). Below 40,000 ft (12,200 m) the irradiation is normally too weak for significant amounts of ozone to form in this manner (although it is formed by reactions between hydrocarbons and nitrogen oxides in the presence of sunlight and contributes to tropospheric air pollution). Concentrations of ozone of 1 ppm at sea level can cause lung irritation. However, modern passenger jet aircraft are fitted with catalytic converters in the environmental control system which break down the ozone before it enters the pressurized cabin.
Aircraft occupants are exposed to elevated levels of cosmic radiation of galactic and solar origin. At jet aircraft operating altitudes, galactic cosmic radiation is 2.5-5 times more intense in polar regions than near the equator. The Earth’s surface is shielded from cosmic radiation by the atmosphere, the ambient radiation increasing with altitude by approximately 15% for each increase of around 2000 ft (dependent on latitude).
Cosmic radiation doses
The effect of ionizing radiation depends not only on the dose absorbed, but also on the type and energy of the radiation and the tissues involved. These factors are taken into account in arriving at the dose equivalent, measured in Sieverts (Sv). However doses of cosmic radiation are so low that figures are usually quoted in microsieverts (mSv) or millisieverts (mSv). Calculated and measured doses for aircrew and frequent flyers are well within the occupational exposure limits recommended by the International Commission on Radiological Protection.
Health risks of cosmic radiation
Whilst it is accepted that there is no level of ionizing radiation exposure below which effects do not occur, current epidemiological evidence indicates that the probability of airline crew members or passengers suffering any abnormality or disease as a result of exposure to cosmic radiation is very low.
The physiological effects of flight are distinguished from those of terrestrial high altitude because exposures are relatively rapid, brief and not cumulative. Flyers do not adapt to the hypoxic environment, unlike inhabitants of terrestrial high altitudes. However, the aircraft can be a means of transporting an individual to a high-altitude destination.
Although ambient oxygen pressure is related exponentially to altitude, falling progressively with ascent from the surface of the Earth, the pressure of oxygen to be found in the lungs does not have the same relationship. That pressure is determined by two equations. The alveolar ventilation equation states that alveolar CO2 pressure (PaCO2) depends only on CO2 excretion (CO2) and alveolar ventilation (Va), so:
where k is a constant. The alveolar air equation states that, since at any one time there is a fixed trading ratio (R) between oxygen uptake and CO2 excretion (R = CO2/O2), alveolar oxygen pressure (PaO2) can be calculated from the moist inspired oxygen pressure (PiO2*) and alveolar PCO2, so:
Progressive hypoxia leads to a mild hyperventilation (i.e. a rise in Va and fall in PaCO2). When arterialized blood leaves a healthy lung, the oxygen pressure is some 10 mm Hg less than that in the alveoli, due to uneven matching of ventilation to perfusion, some anatomical shunting and an almost nominal obstacle to diffusion. In resting individuals, the alveolar-arterial oxygen gradient does not change much with altitude, although the relative importance of the factors contributing to it alter considerably as explained below, so subtracting a further 10-15 mm Hg describes the relationship between arterial oxygen pressure and altitude.
The most important change is the loss of pressure driving oxygen from the alveoli to blood, as the fall in alveolar PO2 is much greater than that in mixed venous PO2 (because of the shape of the oxygen dissociation curve, Figure 26.1). As a result the alveolar- venous gradient for oxygen diffusion is reduced and equilibration is slower than at ground level.
When people ascend to altitude in a matter of minutes, rather than over several days, they react to hypoxia by an increase in blood flow and a modest hyperventilation, limiting the effects of hypoxia. Individuals abruptly exposed to altitudes of 10,000 ft (3000 m) and above suffer mental and physical effects, and this is the ceiling above which aviators are provided with oxygen. To allow a margin of safety, the maximum certified cabin altitude in civilian passenger aircraft is 8000 ft (2440 m), at which barometric pressure is 565 mm Hg and arterial oxygen pressure is around 55 mm Hg (Figure 26.1), and venous oxygen pressures have fallen by only by 1-2 mm Hg. Even at this altitude, there is a decrease in performance. The latest generation passenger aircraft are manufactured from newer materials that are lighter and stronger, allowing a lower cabin altitude to be maintained at higher aircraft altitudes. Two physiological features of altitude hypoxia are important for safety in aviation. The first is the total lack of awareness of cerebral impairment. The second is that there is a ‘time of useful consciousness’, which describes how rapidly consciousness is lost, thus dictating how quickly the condition must be recognized and corrective action taken. The time of useful consciousness is the interval after the onset of hypoxia during which an individual can carry out some purposeful activity. The general relationship between this time interval and the altitude of sudden exposure is shown in Table 26.1. This diminishes from about 4 min at 25,000 ft (7620 m) to a minimum of roughly 15 s, which is reached at around 40,000 ft (12,200 m). This represents the sum of the 7 s or so required for blood to travel from the lungs to the brain and the time needed for the brain to utilize the oxygen already dissolved in its substance.
Table 26.1 The time of useful consciousness following the sudden loss of oxygen supply for a healthy individual at rest and moderately active, both for a progressive decompression and a rapid decompression
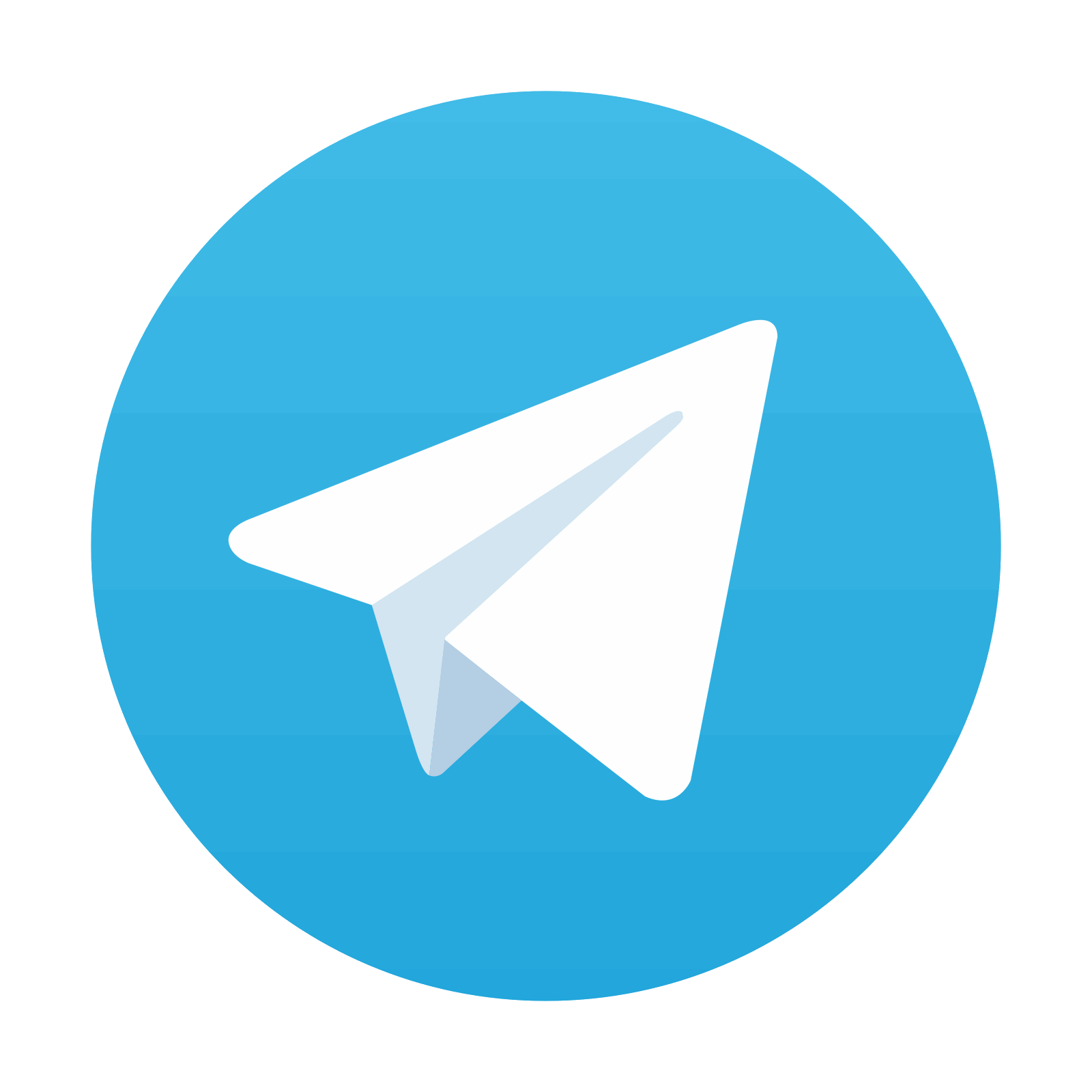
Stay updated, free articles. Join our Telegram channel

Full access? Get Clinical Tree
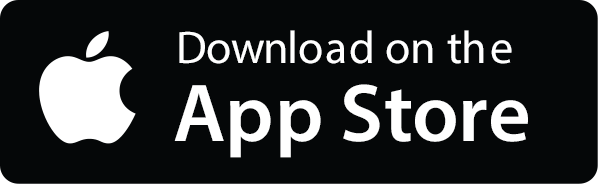
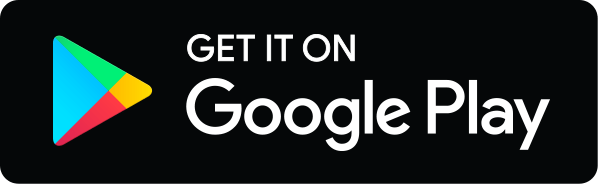