Background
Prolonged exercise has been shown to lead to elevated levels of cardiac troponin and altered cardiac function on echocardiography. It is not known if cardiac synchrony is altered by prolonged exercise. The aims of this study were to assess changes in intra–left ventricular mechanical synchrony and circulating levels of cardiac troponin following prolonged exercise and to evaluate the importance of prior exposure to endurance racing.
Methods
Forty-three male participants in a 30-km cross-country race (20 new participants at this event [median, 3 previous endurance races] age matched against 23 repeat participants [median, 31 previous endurance events]) were assessed prospectively 1 to 2 days before and 24 hours after the race using troponin T and Doppler tissue imaging analyzing the standard deviation of time to peak myocardial systolic velocity (T s -SD) in a six-basal, six-midventricular segment model measuring myocardial synchrony. The insertion/deletion (I/D) polymorphism of the angiotensin-converting enzyme (ACE) gene was also analyzed, as I allele carriers reportedly have superior endurance performance, while the D allele predisposes to renin-angiotensin system–induced cardiac remodeling.
Results
Prerace troponin T was undetectable in all runners, and postrace levels were higher in new runners (median, 0.03 μg/L; interquartile range [IQR], 0.01–0.04 μg/L) than in repeat runners (median, 0.01 μg/L; IQR, 0.01–0.02 μg/L) ( P = .03). Although new and repeat runners had similar T s -SD at baseline (32 msec [IQR, 22–43 msec] vs 34 msec [IQR, 29–45 msec], P = .13), dyssynchrony increased only in new runners (40 msec [IQR, 31–47 msec], P < .001; in repeat runners, median, 38 msec [IQR, 29–43 msec], P = .30; median relative difference, +13% vs +5%, P = .02). ACE genotype distribution was similar in both groups. Multivariate analysis showed that (1) a lack of prior endurance exposure; (2) more copies of the ACE D allele; and (3) lower peak systolic velocity were independent predictors of postrace dyssynchrony ( P < .05 for all).
Conclusion
Prolonged exertion increased ventricular mechanical dyssynchrony in new endurance participants and in ACE D allele carriers. The long-term impact of such changes warrants future study.
Endurance events are rapidly growing in popularity, as part of a healthy lifestyle in many individuals. Although the benefits of regular, moderate exercise are undisputed in the vast majority of the population, the impact of prolonged exercise on the heart is less well characterized. Previous research has shown that long-distance running leads to reversible attenuation of global cardiac function, a phenomenon referred to as cardiac fatigue.
Moreover, detectable levels of cardiac troponin occur reproducibly in some participants in endurance exercise, a finding that correlates to the presence of wall motion abnormalities on echocardiography. This has contributed to concerns regarding possible detrimental effects of such events on the cardiovascular system. Although adverse cardiovascular events are uncommon in athletes, less trained individuals run a higher risk. Interestingly, it has been shown that cardiac fatigue and its associated biomarker release occur predominantly in subjects with less training. This suggests that appropriate preparation will afford protection against cardiac dysfunction associated with participation in endurance exercise events. Importantly, published reports in this field made this discovery post hoc, not in a prospective study design.
Intraventricular dyssynchrony is known to develop in the failing heart and has recently received considerable attention in the context of cardiac resynchronization therapy for heart failure. Although the benefit of CRT is limited to patients with severe disturbances of cardiac conduction and wide QRS complexes, mechanical dyssynchrony has also been described in patients with narrow QRS complexes. In fact, because dyssynchrony is seen in such a diverse range of cardiac disorders, it is increasingly felt to constitute an unspecific sign of myocardial failure. Several methods have been proposed to quantify intra–left ventricular (LV) mechanical dyssynchrony. Many studies have used one or more indices of the dispersion of cardiac mechanical activity on the basis of tissue velocities, typically the time from QRS onset to peak systolic velocity. One widely established technique was pioneered by Yu et al. and involves measuring the standard deviation across 12 myocardial segments. Synchrony may be altered following a brief bout of exercise, as shown by studies in patients with systolic heart failure and normal ejection fractions. However, previous research has not clearly shown whether participants in endurance exercise too may exhibit increased mechanical dyssynchrony. If so, this would suggest that athlete’s cardiac fatigue has phenomenologic similarities to clinical heart failure, albeit on a very mild scale.
We wished to study experienced long-distance race participants compared with new participants and control for age, and we therefore enrolled subjects into a prospective, age-matched design. Besides conventional biochemical analyses we studied the insertion/deletion (I/D) polymorphism of the angiotensin-converting enzyme (ACE) gene, on the basis of reports that this genetic trait plays a role for the heart’s response to prolonged exercise. This is important because D allele carriers have elevated levels of angiotensin II and exhibit adverse cardiac remodeling on activation of the renin-angiotensin system, which places them at an increased risk in the setting of myocardial disorders. We hypothesized that especially inexperienced long-distance runners exhibit increased dyssynchrony after racing, and we sought to establish the impact of carrying the ACE D allele in this setting.
Methods
Subjects
We approached male runners who were registered to participate in the Lidingöloppet 30-km cross-country race, held in September 2008 in Stockholm, Sweden. We recruited a group of new participants who had never taken part in this event before (new participants), as well as an age-matched group of runners with ≥8 previous Lidingöloppet 30-km race participations (repeat participants). Participants completed a questionnaire regarding training habits (hours of exercise per week). The runners’ weights and heights were recorded immediately before and after the race (body mass index was calculated as weight in kilograms divided by the square of height in meters), their blood pressures were recorded by cuff sphygmomanometry, and they underwent blood tests and echocardiography. We also recorded electrocardiograms at rest in a subgroup to ensure that the dyssynchrony measurements used were not influenced by possible alterations of depolarization in this group of trained athletes. Electrocardiography was not repeated after the race, as we have previously shown that QRS width does not increase after endurance running. Cardiovascular fitness was quantified post hoc as peak exercise capacity in a subgroup of participants who underwent standardized supine bicycle stress testing to volitional exhaustion. This study was approved by the institutional committee on human research, and all participants provided written informed consent.
Blood Tests
Blood specimens were taken 99 ± 41 min before the race started and repeated 44 ± 17 min after runners had crossed the finishing line. Blood samples were kept on ice and centrifuged within 4 hours of collection. Cardiac troponin T (TnT) was analyzed without prior freezing (Roche Diagnostics Scandinavia, Bromma, Sweden), and the cutoff for elevation was ≥0.03 μg/L (the clinical decision limit for myocardial necrosis), per the manufacturer’s advice.
Conventional Echocardiography
Baseline echocardiography was performed following a prolonged rest period 1 to 2 days before the race in 35 runners and approximately 3 months after the race in eight subjects (four new and four repeat participants). All subjects underwent postrace echocardiography exactly 1 day after finishing the race. The protocol was designed on the basis of our recent finding that cardiac fatigue is demonstrable for up to 6 days after a race in senior endurance runners. Examinations were performed using commercially available equipment (Vivid 7; GE Vingmed Ultrasound AS, Horten, Norway). Digitized three-cycle loops were analyzed offline at a dedicated workstation (EchoPAC version 6.00; GE Vingmed Ultrasound AS) by a single blinded expert (A.S.). LV mass (LVM) was calculated according to the Penn convention. Left atrial volume was calculated using the prolate ellipse method. LV end-diastolic volume (LVEDV), LV end-systolic volume, and LV ejection fraction (LVEF) were measured using Simpson’s biplane method. Right ventricular (RV) systolic function was assessed by calculating fractional area change (end-diastolic area − end-systolic area). Dimensions were indexed to body surface area.
Doppler Tissue Imaging
Color-coded loops of three beats were recorded onto digital media. Temporal resolution was prioritized by using frame rates > 280 frames/sec to achieve high sensitivity in the analysis of cardiac mechanical events. Tissue velocities were recorded offline as the median of three cardiac cycles. The basal segments of the septum, left lateral wall, and RV free wall were interrogated using a circular sample area (5 × 5 mm). Peak systolic velocity (S m ), early diastolic relaxation (E m ), and late diastolic lengthening velocity (A m ) were recorded. The time from QRS onset to peak systolic velocity (T s ) was recorded ( Figure 1 ) in six segments at the cardiac base and six at the midventricular level (septal, anteroseptal, anterior, lateral, posterior, and inferior). The standard deviation for T s across these 12 segments was computed (T s -SD) as previously described. The maximum difference in T s between any two segments was also recorded (T s -Diff). The difference in T s between basal septum and basal lateral wall (septal-to-lateral [S-L] delay) was computed and defined as positive when septal peak preceded lateral peak. Aortic valve opening was identified by standard pulsed-wave Doppler such that the analysis of T s was based on tissue velocities during systole and not the isovolumetric phase. The coefficient of variation (standard deviation of differences divided by the mean) was 13% for T s and 11.9% for T s -SD (analyzed in 15 echocardiograms randomly selected from the present study population) and 6% to 15% for longitudinal myocardial velocities (analyzed previously in our laboratory). These figures are very similar to those reported using color-coded tissue Doppler to analyze basal segments in a multicenter setting.

Genetic Analysis of the ACE Gene
Deoxyribonucleic acid was isolated from whole blood using the Qiagen Blood and Cell Culture DNA Midi kit (Qiagen GmbH, Hilden, Germany), and the individuals were genotyped for the I/D polymorphism in the ACE gene by polymerase chain reaction, as reported elsewhere. The polymorphism consists of an insertion of a 287–base pair Alu repeat (EMBL-SVA X62855 ; NC_000017.10 ).
Statistical Analyses
Data are presented as mean ± SD or as median (interquartile range) as appropriate. All statistical analyses were performed using SPSS version 15.0 (SPSS, Inc., Chicago, IL). Variables were analyzed for normality using the Kolmogorov-Smirnov test. Correlations were assessed using Pearson’s r or Spearman’s ρ as appropriate. Differences between groups were analyzed using a Mann-Whitney U test or an unpaired t test as appropriate. Categorical variables were analyzed using χ 2 tests (e.g., elevated troponin vs experience, ACE genotype vs experience). Analysis of variance or Kruskal-Wallis analysis was applied for the analysis of continuous variables between more than two groups as appropriate (e.g., in the case of ACE genotypes). Variables that correlated with T s -SD in bivariate analysis were included in a linear regression model. Residuals were examined for normality and homoscedasticity and also used to identify outliers. For analysis of predictors of dyssynchrony, ACE genotype was treated as an ordinal variable on the basis of the number of copies of the D allele. In all statistical analyses, a two-tailed P value < .05 was considered significant. Power analysis was used to establish the sample size required to detect a difference in peak workload for α = 0.05 and β = 0.80.
Results
A total of 43 male subjects were recruited: 20 new participants (3 events [1–5 events]) and 23 repeat participants (31 previous endurance events [10–55 events]) who were well matched with respect to age ( Table 1 ). Repeat participants were both faster (race duration, 173 ± 29 vs 210 ± 38 min) and leaner (body mass index, 24 ± 2 vs 26 ± 3 kg/m 2 ) than new participants ( P < .01 for both). All subjects had undetectable baseline levels of TnT, rising to 0.02 μg/L (0.01–0.03 μg/L) after the race ( P < .001). Levels of TnT after the race were significantly higher in new than in repeat participants (0.03 μg/L [0.01–0.04 μg/L] vs 0.01 μg/L [0.01–0.02 μg/L], P = .03). Elevation of TnT (≥0.03 μg/L) was seen in 14 of 43 runners (10 of 20 new participants [50%] and 4 of 23 repeat participants [17%]). Conventional echocardiography showed a difference at baseline between new and repeat participants in indexed LVEDV but not indexed LVM or the LVEF ( Tables 1 and 2 ). The race did not lead to altered LV size in either subgroup (new participants, P = .48; repeat participants, P = .10). Heart rate did not differ significantly between groups at baseline or after the race ( Table 1 ). Tissue velocities were similar between groups at baseline (septal S m , 7 ± 1 vs 7 ± 1 cm/sec; septal E m , 9 ± 2 vs 9 ± 1 cm/sec; lateral S m , 7 ± 2 vs 7 ± 2 cm/sec; lateral E m , 12 ± 3 vs 12 ± 2 cm/sec) and did not change ( P > .18), with the exception of lateral E m , which tended to be reduced 24 hours after the race in new participants ( P = .06). RV function did not differ between new and repeat participants, and values 24 hours after the race were similar to baseline (RV S m , 12 ± 1 vs 12 ± 2 cm/sec; RV E m , 12 ± 2 vs 12 ± 2 cm/sec; RV A m , 12 ± 3 vs 12 ± 3 cm/sec; P > 0.14; Table 2 ). Standard 12-lead electrocardiography was performed in 24 subjects (56%) and showed QRS widths to be normal in all subjects and similar between new participants (98 ± 7 ms) and repeat participants (99 ± 10 ms) ( P = 0.82). One repeat participant had left anterior hemiblock and an incomplete right bundle branch block. Peak exercise capacity during supine bicycle stress did not differ between new ( n = 11) and repeat ( n = 16) participants, as shown in Table 1 . Power analysis performed on these data showed that ≥49 subjects would have been required in each group to detect an underlying difference in exercise capacity between subjects in the experienced and beginner groups.
New runners | Repeat runners | ||
---|---|---|---|
Variable | ( n = 20) | ( n = 23) | P |
Age (years) | 46 ± 8 | 46 ± 6 | .79 |
Previous participations ∗ | 0 (0– 0) | 11 (9– 18) | <.001 |
Previous endurance events | 3 (1– 5) | 31 (10– 55) | <.001 |
Endurance events per year | 2 (0– 3) | 3 (2– 3.5) | .02 |
Training (km/week) | 20 (10–35) | 30 (18.5– 32.5) | .26 |
Body weight, baseline (kg) | 87 ± 10 | 79 ± 10 | .008 |
Body weight, change (%) | −2 ± 1 | −2 ± 1 | .55 |
Systolic/diastolic blood pressure (mm Hg) | 130 ± 13/79 ± 7 | 125 ± 11/77 ± 6 | .15 |
Heart rate (beats/min) | 62 ± 11 | 57 ± 8 | .10 |
Heart rate, postrace (beats/min) | 64 ± 10 | 59 ± 12 | .09 |
Race duration (min) | 210 ± 38 | 173 ± 29 | .001 |
Peak exercise capacity (W) † | 290 ± 44 | 315 ± 58 | .22 |
IVSd (mm) | 10 ± 2 | 10 ± 2 | .71 |
PWDd (mm) | 8 ± 1 | 8 ± 1 | .68 |
LVMi (g/m 2 ) | 88 ± 22 | 95 ± 19 | .28 |
ACE genotype | .93 | ||
DD | 6 (30%) | 6 (27%) | |
ID | 7 (35%) | 9 (41%) | |
II | 7 (35%) | 7 (32%) |
∗ Participations refers to previous participations at the Lidingöloppet 30-km race.
New runners | Repeat runners | ||
---|---|---|---|
Variable | ( n = 20) | ( n = 23) | P |
LAVi, baseline (mL/m 2 ) | 20 ± 6 | 23 ± 5 | .12 |
LAVi, postrace (mL/m 2 ) | 22 ± 5 | 22 ± 6 | .98 |
LVIDDi, baseline (mm/m 2 ) | 26 ± 2 | 27 ± 2 | .008 |
LVIDDi, postrace (mm/m 2 ) | 26 ± 3 | 27 ± 2 | .16 |
LVEDVi, baseline (mL/m 2 ) | 60 ± 11 | 70 ± 7 | .001 |
LVEDVi, postrace (mL/m 2 ) | 62 ± 8 | 66 ± 9 | .17 |
LVEF, baseline (%) | 58 ± 5 | 59 ± 5 | .76 |
LVEF, postrace (%) | 60 ± 5 | 60 ± 5 | .91 |
RVEDAi, baseline (cm 2 /m 2 ) | 10 ± 2 | 11 ± 2 | .46 |
RVEDAi, postrace (cm 2 /m 2 ) | 10 ± 2 | 11 ± 2 | .45 |
RV FAC, baseline (%) | 40 ± 8 | 43 ± 5 | .23 |
RV FAC, postrace (%) | 42 ± 8 | 42 ± 9 | .71 |
Measures of Intra-LV Dyssynchrony
At baseline, T s in the septum was longer in repeat participants than in new participants (base, 177 ± 37 vs 156 ± 36 ms, P = .06; mid septum, 190 ± 46 vs 166 ± 38 ms, P = .05), but peak velocity occurred earlier at the posterior wall (136 ± 35 vs 158 ± 34 ms, P = .02). There were no differences between values at baseline and 24 hours after the race for S-L delay in the group overall (−9 ms [−57 to 3 ms] vs −15 ms [−68 to 11 ms], P = .40). Among repeat participants, 18 of 23 subjects (78%) had longer S-L delays at the septum than at the lateral wall (i.e., negative S-L delays), while this was the case in only 12 of 20 new participants (60%), leading to a significant difference in S-L delay at baseline between the two groups ( P = .01; Table 3 ). The race tended to have opposite effects on S-L delay in the two subgroups: an increase in S-L delay (129% [−50% to 346%]) in new participants but a reduction (−32% [−126% to 6%]) in repeat participants ( P = .06).
New runners | Repeat runners | |||||
---|---|---|---|---|---|---|
Variable | Before | After | P | Before | After | P |
S-L delay (ms) | −3 (−18 to +9) ∗ | −20 (−77 to +14) | .10 | −47 (−74 to −2) ∗ | −12 (−67 to +11) | .10 |
T s -SD (ms) | 32 (22 to 43) | 40 (31 to 47) | .001 | 34 (29 to 45) | 38 (29 to 43) | .30 |
T s -Diff (ms) | 96 (69 to 112) | 105 (92 to 130) | <.01 | 104 (95 to 128) | 102 (84 to 113) | .22 |
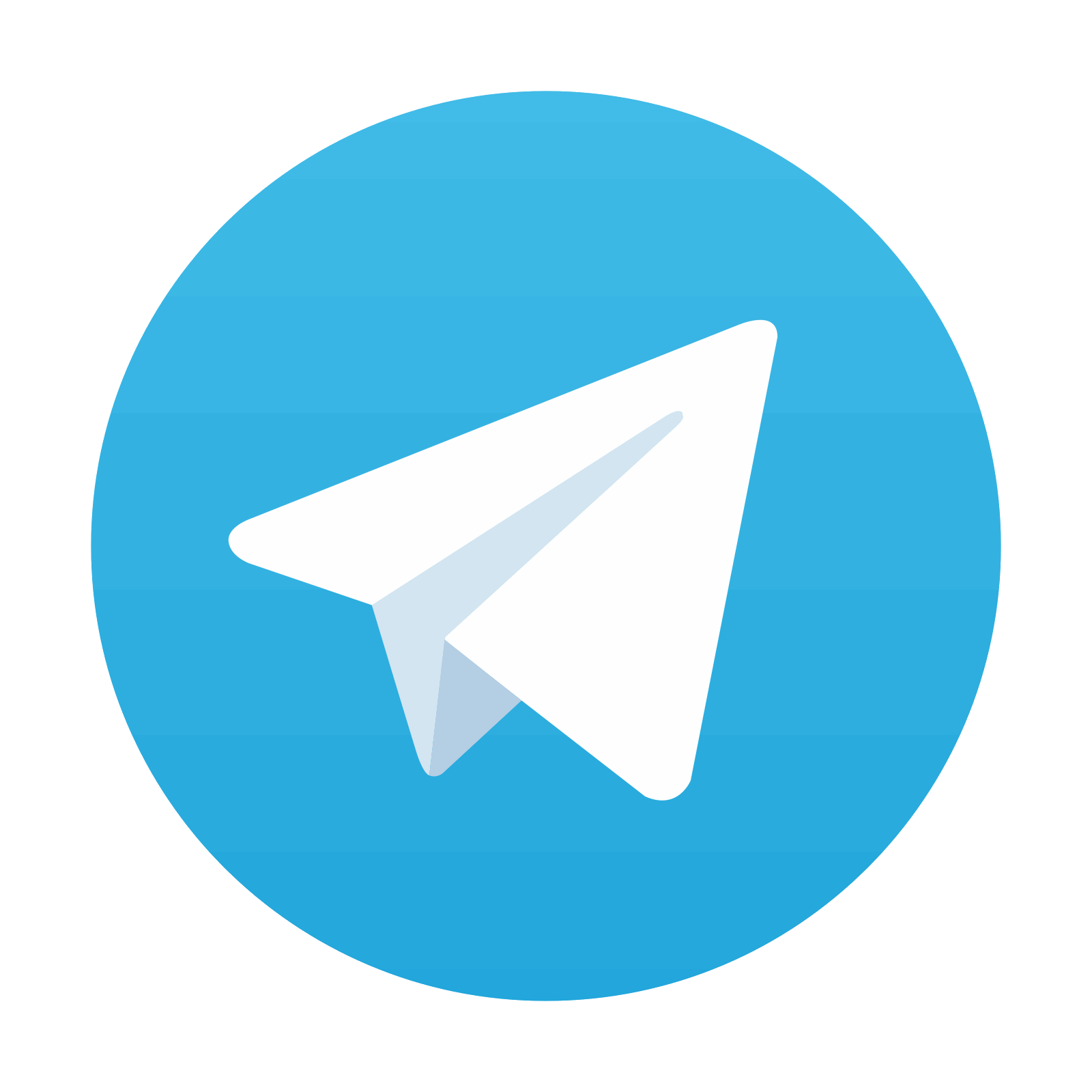
Stay updated, free articles. Join our Telegram channel

Full access? Get Clinical Tree
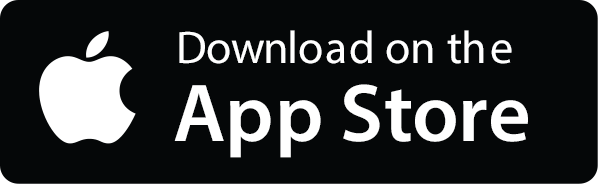
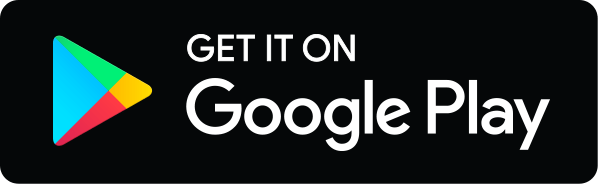
