Chapter 4 Effects of positioning and mobilization
INTRODUCTION

In addition, the physiological and scientific rationale for use of positioning and mobilization for each of the above effects is described. Conceptualizing cardiopulmonary dysfunction as impairment of the steps in the oxygen transport pathway and exploiting positioning and mobilization as primary interventions in remediating this impairment will maximize physiotherapy efficacy. Emphasis is placed on impairment of oxygen transport given that such impairment in large part determines disability and handicap (Verbrugge & Lette 1993), as defined by the World Health Organization (1980), secondary to cardiopulmonary dysfunction.
The following terms (Ross & Dean 1989) have been adopted in this chapter:
CONCEPTUAL FRAMEWORK FOR CLINICAL DECISION-MAKING
The oxygen transport pathway
Optimal cardiopulmonary function and gas exchange reflect the optimal matching of oxygen demand and supply (Dantzker 1983, Weber et al 1983). Oxygen delivery and oxygen consumption based on demand are essential components of the oxygen transport system. Figure 4.1 shows the components of oxygen delivery (O2, namely arterial oxygen content and cardiac output (CO), and the components of oxygen consumption (
O2), namely the arteriovenous oxygen content difference and CO. In health,
O2is approximately four times greater than
O2at rest so there is considerable oxygen reserve that is drawn upon during times of increased metabolic demand such as exercise, stress, illness and repair. Because of the large reserve,
O2is thought to be normally supply-independent. This reserve capacity, however, becomes compromised secondary to acute and chronic pathological conditions. In patients who are critically ill and
O2 is severely compromised,
O2 may be supply-dependent until
O2 reaches a critical threshold, i.e. the level at which metabolic demands are met (Phang & Russell 1993). Below this critical threshold, patients are increasingly dependent on anaerobic metabolism reflected by increased minute ventilation, respiratory exchange ratio and serum lactate levels.
The efficiency with which oxygen is transported from the atmosphere along the steps of the oxygen transport pathway to the tissues determines the efficiency of oxygen transport overall (Fig. 4.2). The steps in the oxygen transport pathway include ventilation of the alveoli, diffusion of oxygen across the alveolar capillary membrane, perfusion of the lungs, biochemical reaction of oxygen with the blood, affinity of oxygen with haemoglobin, cardiac output, integrity of the peripheral circulation and oxygen extraction at the tissue level (Wasserman et al 1987). At rest, the demand for oxygen reflects basal metabolic requirements. Metabolic demand changes normally in response to gravitational (positional), exercise and psychological stressors. When one or more steps in the oxygen transport pathway are impaired secondary to cardiopulmonary dysfunction, oxygen demand at rest and in response to stressors can be increased significantly. Impairment of one step in the pathway may be compensated by other steps, thereby maintaining normal gas exchange and arterial oxygenation. With severe impairment involving several steps, arterial oxygenation may be reduced, the work of the heart and lungs increased, tissue oxygenation impaired and, in the most extreme situation, multiorgan system failure may ensue.
While the oxygen transport pathway ensures that an adequate supply of oxygen meets the demands of the working tissues, the carbon dioxide pathway ensures that carbon dioxide, a primary by-product of metabolism, is eliminated. This pathway is basically the reverse of the oxygen transport pathway in that carbon dioxide is transported from the tissues, via the circulation, to the lungs for elimination. Carbon dioxide is a highly diffusible gas and is readily eliminated from the body. However, carbon dioxide retention is a hallmark of diseases in which the ventilatory muscle pump is operating inefficiently or the normal elastic recoil of the lung parenchyma is lost.
Factors contributing to cardiopulmonary dysfunction
An analysis of the factors that contribute to cardiopulmonary dysfunction provides the basis for assessment and prescribing the parameters of positioning and mobilization, to enhance oxygen transport for a given patient. The treatment is directed at the specific underlying contributing factors. In some cases, e.g. low haemoglobin, the underlying impairment of oxygen transport cannot be affected directly by physical intervention. However, mobilization and exercise can improve aerobic capacity in patients with anaemia, a factor not directly modifiable by non-invasive physiotherapy interventions, by increasing the efficiency of other steps in the oxygen transport pathway (Williams 1995). Further, even though some factors are not directly modifiable by non-invasive physiotherapy interventions, they influence treatment outcome, and thus need to be considered when planning, modifying and progressing treatment. Fluid imbalance for example is the result of many factors, including reduced muscle activity (Koomans & Boer 1997). Physiotherapy that incorporates body position and exercise challenges may have a profound effect on fluid balance by maintaining fluid balance between the intra- and extravascular compartments. Research is needed to address this compelling proposition.
Ageing and weight deserve special consideration. Patients who are older have progressively lower lung function, and oxygen transport reserve capacity overall (Rossi et al 1996). These changes predispose this population, who tend to constitute the majority of patients in the intensive care unit (ICU), to complications. In addition, obesity often complicates the clinical picture of patients in the ICU: the heavier the patient, the greater the risk. Body positioning and mobilization are particularly important is offsetting the deleterious effects of being recumbent with the added physical burden of adipose tissue surrounding the chest wall (Beck 1998).
Multisystem organ dysfunction and failure may lead to or result from cardiopulmonary dysfunction; thus they are associated with significant mortality and mor bidity. In these conditions, multiple factors impair multiple steps in the oxygen transport pathway, so identifying which steps are affected and amenable to physiotherapy interventions is central to optimal treatment outcome (Dean 1994b, 2006a).
Consultation with the ICU team, including a nutritionist, is critical to enhancing physiotherapy outcomes (Dean & Perme 2006). Increased metabolic demands of patients in the ICU, coupled with the demands of interventions, necessitate appropriate nutritional support to prevent or address malnutrition. Such screening has been shown to lead to better outcomes from ICU in terms of reduced complications, reduced hospital stays and reduced costs (Reid & Allard-Gould 2004).
Anaemaia associated with critical illness (Shander 2004) is a concern for the physiotherapist whose goal is to optimize O2. Haemoglobin is fundamental to optimal
O2, however, optimal management of anaemia is not performed universally. Special attention should be paid to haemoglobin status and its remediation should be a focus of discussion by the multidisciplinary team.
Assessment and monitoring
The physiotherapist needs to be particularly skilled at assessment and monitoring in the ICU both for safety reasons and when monitoring treatment response. Response to treatment can vary from minute to minute. The intervention is prescribed and progressed, and modified as needed during a treatment. Assessment has been addressed in Chapter 1 and includes a range of measures and indices of each step in the oxygen transport pathway (Dean 1999). Further, the physiotherapist needs to recognize that oxygen transport deficits and threats are mediated through impairments of virtually all organ systems not only the lungs and heart (Dean 1997). Consistent with the World Health Organization’s Definition of Health (World Health Organization 2006) and the International Classification of Function (World Health Organization 2002), consideration is also given to assessing activity and health-related quality of life measures even for patients in the ICU setting. Central to prescription and progression of treatment is the patient’s response. For safe and optimal outcomes, response-driven treatment progression is paramount, rather than blind adherence to a protocol-driven intervention. This approach helps to provide seamless care from the ICU back to the community with reduced risk of re-admission to the hospital, in the interest of lifelong health.
THERAPEUTIC EFFECTS OF POSITIONING AND MOBILIZATION
To improve oxygen transport in acute cardiopulmonary dysfunction
Positioning and mobilization have profound acute effects on cardiovascular and cardiopulmonary function and hence on the up-regulation of oxygen transport capacity (Table 4.1). These effects translate into improved gas exchange overall: reduction in the fraction of inspired oxygen, pharmacological and ventilatory support (Burns & Jones 1975, Dean 2006b, 2006c, Svanberg 1957). Such effects need to be exploited in the management of acute cardiopulmonary dysfunction with the use of positioning and mobilization as primarytreatment interventions to enhance oxygen transport and as between-treatment interventions (Dean & Ross 1992b). The physiotherapist’s role is to prescribe these interventions judiciously, to optimize gas exchange and oxygen transport overall. This role is distinct from routinepositioning and mobilization often performed jointly by the physiotherapy and nursing staff. The aim of routine positioning and mobilization is primarily to reduce the adverse effects of restricted mobility, including pulmonary complications, bedsores and contractures. Critical-illness neuropathies and myopathies can lead to irreversible consequences and need to be prevented or at least detected early and managed.
Table 4.1 Acute effects of upright positioning and mobilization on oxygen transport
Systemic response | Stimulus | |
---|---|---|
Positioning (supine to upright) | Mobilization | |
Cardiopulmonary | ||
Cardiovascular |
AP, anteroposterior; ↑, increases; ↓, decreases; A-aO2, alveolar-arterial oxygen gradient; A/
, alveolar-ventilation perfusion; PaO2, partial pressure of oxygen in arterial blood
(Adapted from Dean and Ross (1992a) and Imle and Klemic (1989))
Non-invasive care in the form of physiotherapy has a particularly important place in the contemporary ICU unit. Advances in medical management have contributed to the avoidance of ICU admissions in patients who would have been admitted to the ICU a couple of decades ago. Similar advances have also contributed to patient survival; many of those who survive today may not have survived a couple of decades ago. Furthermore, advances in ventilatory support including non-invasive mechanical ventilation have helped to titrate ventilatory support more effectively to each patient, and in turn, reduce the need for intubation and invasive ventilation, and the iatrogenic effects of invasive ventilatory support. These advances have increased the demand on physiotherapy to optimize O2, and minimize undue
O2. Priming the oxygen transport system with progressive gravitational and exercise stress is the primary responsibility of the physiotherapist in the ICU. This requires a high level of expertise in monitoring and judiciously challenging the oxygen transport system through prescriptively challenging the patient, while minimizing risk to haemodynamic and ventilatory status. As patient survival has improved, the severity of the illness of patients in the ICU has increased, and patients can be kept alive longer. Thus, the physiotherapist needs to assess patients early, in order to establish the appropriate timing for intervention. Weaning or freeing patients from mechanical ventilation is a priority from the beginning. Weaning failure is not uncommon given the severity of the patients’ status, and warrants careful consideration by the team as a whole in helping to prevent failure in both those patients who are non-invasively and invasively ventilated, and contributing to weaning success (Moretti et al 2000, Squadrone et al 2004). However, the physiotherapist needs to do everything possible to optimize
O2through intervention, and minimize the need for mechanical ventilation, particularly invasive ventilation because it is associated with poorer clinical outcomes (Bernieh et al 2004).
Mechanical ventilation, both non-invasive and invasive, needs to be thoroughly understood by the physiotherapist in terms of advantages and disadvantages, and these may differ depending on the patient’s status and needs (Brochard 2003). The physiotherapist should be particularly interested in promoting non-invasive ventilation as it facilitates body positioning and mobilization. These interventions have evidence-based support for optimizing oxygen delivery when prescribed appropriately. When a patient is intubated and receiving invasive mechanical ventilation, this in no way precludes the prescription of these interventions. Patients decondition rapidly when gravitational and exercise stressors are removed. It is particularly important to offset these effects in patients with a history of prolonged debility before admission, and elderly patients (Sevransky & Haponik 2003).
Extubation success is a primary concern of the physiotherapist. Failure to extubate is associated with prolonged hospital stay and, in turn, healthcare cost (Seymour et al 2004). Body positioning and mobilization maintain or increase general body conditioning, which may be argued is fundamental to successful weaning from mechanical ventilation. This may be particularly important for patients with neuromuscular conditions who are weak and at high risk of aspiration (Vianello et al 2000). While the objective of optimizing conditioning may initially appear a premature priority given the status of patients who are severely ill in the ICU, cough strength that can be correlated to general body strength, or the converse, a weak cough related to general debility, can be predictive of extubation outcome (Khamiees et al 2001). Being able to tolerate a spontaneous breathing trial is key to eventual weaning. Furthermore, maintaining spontaneous breathing may help minimize sedation and improve cardiopulmonary function in some patients, for example patients with acute lung injury and receiving airway pressure-released ventilation (Putensen et al 2001). Weaning needs to be tailored to each patient and should not be unnecessarily prolonged (Nevins & Epstein 2001).
Figure 4.3 illustrates positioning upright and mobilization of a patient in the ICU. The progression is based on ongoing assessment and monitoring, with a gravitational challenge followed by progressive movement. The rationale and the details of ongoing monitoring follow.
Positioning
Physiological and scientific rationale.
The distributions of ventilation (A), perfusion (
) and ventilation and perfusion matching in the lungs are primarily influenced by gravity and therefore body position (Clauss et al 1968, West 1962, 1977). The goal is to reduce closing volume and optimize functional residual capacity (Manning et al 1999). The intrapleural pressure becomes less negative down the upright lung. Thus, the apices have a greater initial volume and reduced compliance than the bases. Because the bases are more compliant in this position, they exhibit greater volume changes during ventilation. In addition to these gravity-dependent inter-regional differences in lung volume, ventilation is influenced by intra-regional differences, which are dependent on regional mechanical differences in the compliance of the lung parenchyma and the resistance to airflow in the airways. Perfusion increases down the upright lung such that the
A/
ratio in the apices is disproportionately high compared with that in the bases. Ventilation and perfusion matching is optimal in the mid-lung region. Manipulating body position alters both inter-regional and intra-regional determinants of ventilation and perfusion and their matching. When choosing specific positions to enhance arterial oxygenation for a given patient, the underlying pathophysiology impairing cardiopulmonary function, the effects of bed rest/recumbency and restricted mobility, extrinsic factors related to the patient’s care and intrinsic factors related to the patient need to be considered.
Although the negative effects of the supine position have been well documented for several decades (Dean & Ross 1992b, Dripps & Waters 1941), supine or recumbent positions are frequently assumed by patients in hospital. These positions are non-physiologic and are associated with significant reductions in lung volumes and flow rates and increased work of breathing (Craig et al 1971, Hsu & Hickey 1976). The decrease in functional residual capacity (FRC) contributes to closure of the dependent airways and reduced arterial oxygenation (Ray et al 1974). This effect has long been known to be accentuated in older persons (Leblanc et al 1970), patients with cardiopulmonary disease (Fowler 1949), patients with abdominal pathology, smokers and individuals who are obese.
The haemodynamic consequences of the supine position are also remarkable. The gravity-dependent increase in central blood volume may precipitate vascular congestion, reduced compliance and pulmonary oedema (Blomqvist & Stone 1983, Sjostrand 1951). The commensurate increase in stroke volume increases the work of the heart (Levine & Lown 1952). Within 6 hours, a compensatory diuresis can lead to a loss of circulating blood volume and orthostatic intolerance: i.e. haemodynamic intolerance to the upright position. Bed rest deconditioning has been attributed to this reduction in blood volume and the impairment of the volume-regulating mechanisms rather than physical deconditioning per se (Hahn-Winslow 1985). Thus, the upright position is essential to maximize lung volumes and flow rates and this position is the only means of optimizing fluid shifts such that the circulating blood volume and the volume-regulating mechanisms are maintained. The upright position coupled with movement is necessary to promote normal fluid regulation and balance (Lamb et al 1964).
The upright position is a potent stimulus to the sympathetic nervous system. This is an important clinical effect, which offsets impaired blood volume and pressure-regulating mechanisms secondary to recumbency (Hahn-Winslow 1985
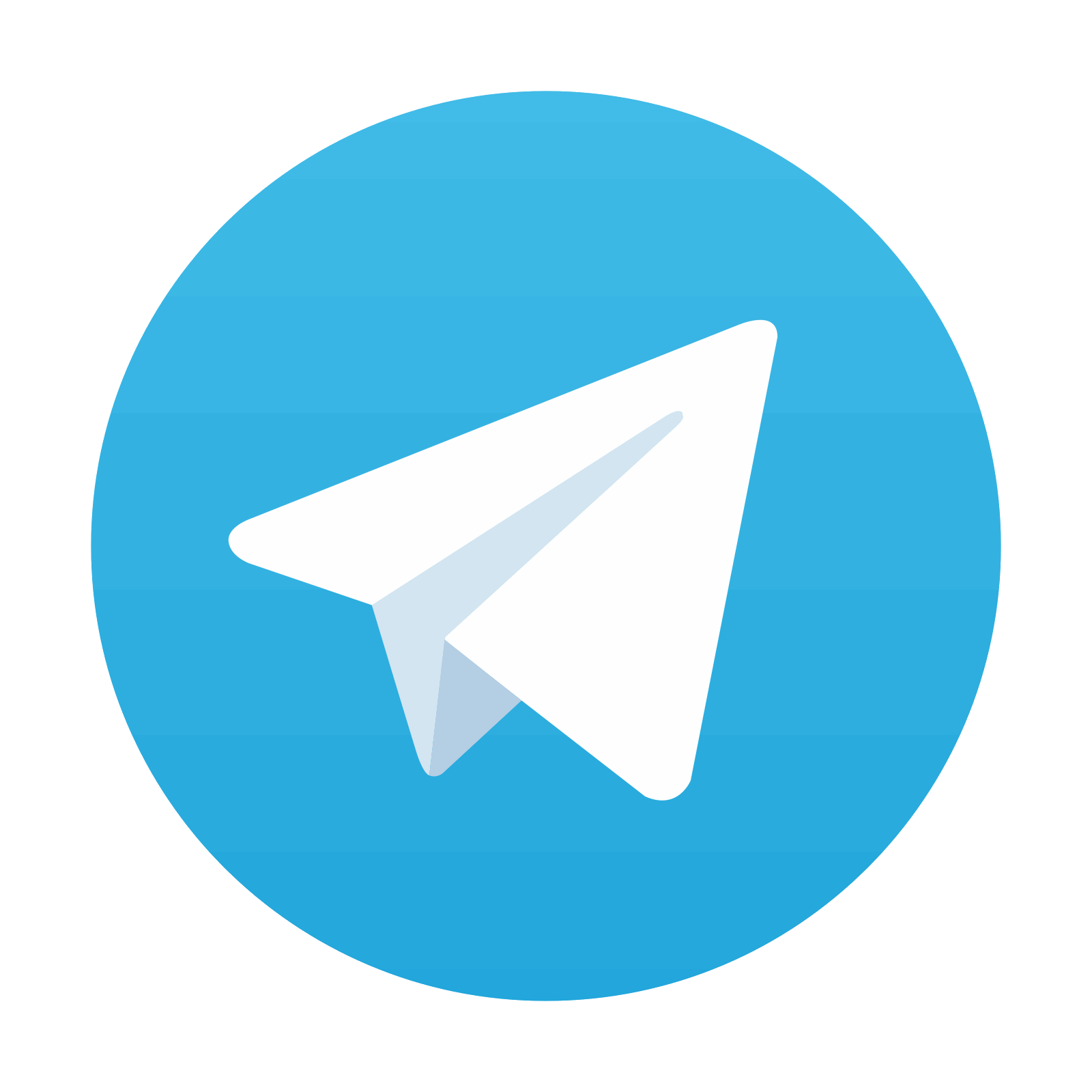
Stay updated, free articles. Join our Telegram channel

Full access? Get Clinical Tree
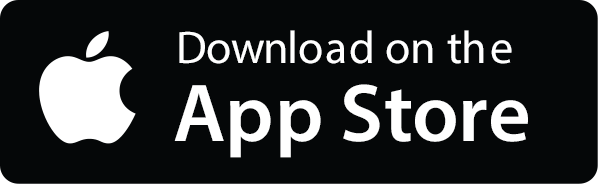
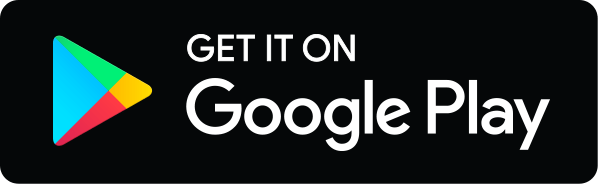
