Background
Accurate assessment of the right ventricle is increasingly important. Measures of right ventricular (RV) systolic function, including fractional area change, tissue Doppler (s′ velocity), and tricuspid annular plane systolic excursion, show significant variation, and the impacts of age and gender are unclear. The aim of this study was to determine the effects of gender and age on global and segmental RV systolic and diastolic function using both traditional echocardiographic and two-dimensional strain parameters.
Methods
Detailed transthoracic echocardiographic studies were performed on 142 healthy adult volunteers, with particular emphasis on the right ventricle to determine RV dimensions and function, including fractional area change, tricuspid annular plane systolic excursion, s′ velocity, global and segmental systolic strain, and systolic, early diastolic, and late diastolic strain rates.
Results
Tricuspid annular plane systolic excursion ( r = −0.4, P < .001) and RV s′ velocity ( r = −0.5, P < .001) as well as diastolic functional parameters, including transtricuspid peak E velocity and RV free wall e′ velocity ( r = −0.4, P < .001), decreased with age. Global systolic strain was also reduced, with differential reductions in basal and mid segmental strain with age. Early diastolic strain rate decreased, with a corresponding increase in late diastolic strain rate. RV function parameters, including fractional area change, e′ velocity, strain, and strain rate, were significantly lower in men.
Conclusions
RV functional analysis by two-dimensional strain demonstrates a small yet significant change in global and segmental RV function with age and gender, and therefore adjustment for these measures is required in the evaluation of RV function.
Right ventricular (RV) dysfunction has prognostic significance independent of left ventricular (LV) function in many conditions, including heart failure, RV myocardial infarction, pulmonary embolism, and pulmonary hypertension. However, accurate echocardiographic assessment of the right ventricle is difficult because of its complex geometry, and its retrosternal position limits echocardiographic imaging.
Current guidelines for the quantification of RV systolic function include fractional area change (FAC), tricuspid annular plane systolic excursion (TAPSE), and RV lateral annular tissue Doppler systolic velocity (RV s′). FAC is limited by endocardial border definition and a large range of normality. The latter two measures generalize RV function along a single geometric axis and may represent basal RV segmental function, particularly in instances in which segmental RV dysfunction is present.
Two-dimensional (2D) speckle-tracking strain detects early or subclinical dysfunction in the left ventricle. Strain measures myocardial deformation, and strain rate is the rate at which this deformation occurs. Strain has also been used for RV assessment, and it is relatively load independent, but there is paucity of data on normal reference values, and strain evaluation is limited by the lack of cross-platform standardization.
Our aim was to determine the effects of healthy aging and of gender on global and segmental RV systolic and diastolic function using both traditional and newer echocardiographic techniques of 2D strain. We hypothesized that strain parameters would demonstrate changes in RV function with age and gender.
Methods
The study proposal was approved by the Research Ethics Committee of the University of New South Wales and the Sydney South West Area Health Service. Written consent was obtained from all participants.
We prospectively recruited healthy adult volunteers from the local community. No subject included had been referred to the echocardiography laboratory. A detailed history was obtained by a physician, and subjects were excluded if they were currently or previously on any cardioactive medications or had any cardiovascular risk factors (smoking, hypertension, hypercholesterolemia, and diabetes mellitus) or if they had a history of ischemic or valvular heart disease, cerebrovascular or peripheral vascular disease, previous arrhythmias, or chronic lung disease (chronic obstructive pulmonary disease, asthma, or sleep apnea). Blood tests (including blood count, electrolytes, estimated glomerular filtration rate, thyroid and liver function, and lipids) performed by local doctors were reviewed. Participants were examined by a physician to exclude significant valvular disease and left or right heart failure; pulse rate and blood pressure were recorded as the average of two recordings. An electrocardiogram showing sinus rhythm, combined with a detailed history of lack of palpitations, was deemed sufficient to rule out significant arrhythmias. No recruited subject had any degree of valvular stenosis or greater than mild grade of regurgitation on echocardiography. All subjects underwent stress testing to rule out latent ischemia. Peak expiratory flow rate analysis was performed as a simple screening tool for significant alteration in pulmonary function.
Comprehensive transthoracic echocardiography was performed by three dedicated sonographers using the Vivid 7 system (GE Healthcare, Milwaukee, WI) and a 3.0-MHz variable frequency transducer, with the patient in the left lateral position. Images were obtained from the standard views (parasternal, apical, and subcostal views). A RV focused view as recommended by the American Society of Echocardiography was obtained from a more lateral window at a lower intercostal space than the usual apical view ( Figure 1 ). Five cardiac cycles at end-expiration were stored digitally and analyzed offline.

Echocardiographic Measurements
LV end-diastolic and end-systolic volumes were obtained from the apical four- and two-chamber views using Simpson’s method of disks, and biplane LV ejection fraction was calculated. LV s′ velocity was obtained from the septal mitral annulus using Doppler tissue imaging (DTI). Transmitral peak E and A velocities, E/A ratio, and deceleration time were measured. The DTI e′ velocity was obtained and the E/e′ ratio calculated. Biplane maximal left atrial volumes were obtained.
Basal and mid RV diameters and longitudinal dimension were obtained at end-diastole and right atrial (RA) area was obtained at end-systole from the RV focused view. RV end-systolic and end-diastolic areas were measured, and RV FAC was calculated. TAPSE was determined by placing an M-mode cursor through the tricuspid annulus and measuring the peak systolic longitudinal annular motion. RV s′ was measured as the peak systolic velocity using pulsed-wave DTI with the sample placed at the basal lateral RV annulus. The RV myocardial performance (Tei) index was measured as previously described. Transtricuspid peak E and A velocity, E/A ratio, RV e′ velocity (lateral RV annulus), and RV E/e′ ratio were obtained. Pulmonary pressure was estimated from the tricuspid regurgitant velocity by using the modified Bernoulli equation with the addition of maximum RA pressure estimated from the inferior vena cava diameter and collapsibility. Pulmonary vascular resistance (PVR) was estimated using the ratio of tricuspid regurgitant velocity to the RV outflow tract velocity-time integral.
Systolic and diastolic 2D strain parameters were obtained from RV focused images (>60 frames/sec). The right ventricle was divided into six segments (septal and RV free wall basal, mid, and apical segments). Offline analysis was performed with specialized software (EchoPAC; GE Healthcare) developed for measuring LV strain. A region of interest was manually traced along the endocardium in end-systole ( Figure 1 ), with its width adjusted to match the RV wall thickness, excluding pericardium and LV endocardium. Strain and strain rate were measured by an experienced observer ( Figure 2 ). If any of the three RV free wall segments were not approved because of inappropriate tracking or dropout, the patient was excluded ( n = 6). Global RV strain was the average of the RV lateral basal, mid, and apical segments, with the exclusion of the septal segments as previously described. A six-segment model including the RV septal segments was also measured. Subjects were divided by decade for analysis; additionally, analysis was performed with division into three two-decade groups as follows: group 1, 20 to 39 years; group 2, 40 to 59 years; and group 3, ≥60 years ( Supplemental Table 1 ; available at www.onlinejase.com ). All measurements are averages of three consecutive cardiac cycles.

In a subset of 40 subjects, inter- and intraobserver variability was assessed. One observer measured strain twice 1 month apart, blinded to the results of the first measurements. Measurements were performed on the same subjects by a second observer, blinded to the initial results. A further subset of 10 patients underwent repeat apical four-chamber imaging ≥1 hour after the initial scan for test-retest variability.
Statistical Analyses
Measurements were analyzed using SPSS version 16 (SPSS, Inc, Chicago, IL). All values are expressed as mean ± 2 SDs. Linear regression analysis was used to examine the relationship of RV function parameters with age. Multiple linear regression analysis was performed using demographic, anthropometric, and clinically important variables to evaluate the independent predictors of RV strain parameters. Independent t test was used to analyze gender differences, with parameters indexed to body surface area (BSA). Differences among age groups were examined by using one-way analysis of variance. Intraobserver, interobserver, and test-retest variability are expressed using intraclass correlation coefficients and coefficients of variation.
Results
This study included 142 healthy subjects (mean age, 45 ± 15 years; range, 20–81 years; 53% men) of various ethnicities (52% Caucasian, 24% South Asian, 20% Southeast Asian, and 4% other ethnicities). There were no significant correlations between height, weight, or BSA and age ( Table 1 ). With increasing age, systolic, diastolic, and mean arterial blood pressure increased, whereas heart rate and peak expiratory flow rate decreased. All parameters remained within normal limits.
Variable | Value | r | P |
---|---|---|---|
Men | 75 (53%) | ||
BSA (kg/m 2 ) | 1.81 ± 0.19 | 0.06 | NS |
Height (cm) | 168 ± 10.2 | 0.1 | NS |
Weight (kg) | 71.7 ± 13.4 | 0.06 | NS |
Systolic BP (mm Hg) | 118 ± 11 | 0.43 | <.001 |
Diastolic BP (mm Hg) | 73 ± 9 | 0.22 | .01 |
MAP (mm Hg) | 88 ± 8 | 0.34 | <.001 |
Heart rate (beats/min) | 77 ± 11 | −0.32 | <.001 |
Peak expiratory flow (L/min) | 496 ± 115 | −0.26 | .007 |
Indexed LV EDV (mL/m 2 ) | 46.1 ± 11.9 | 0.10 | NS |
Indexed LV ESV (mL/m 2 ) | 19.0 ± 6.9 | 0.02 | NS |
Indexed LA volume (mL/m 2 ) | 25.7 ± 8.1 | 0.30 | <.001 |
LV EF (%) | 58.7 ± 8.1 | −0.31 | <.001 |
LV s′ (cm/sec) | 7.6 ± 1.5 | −0.34 | <.001 |
Mitral peak E (m/sec) | 0.74 ± 0.15 | −0.37 | <.001 |
Mitral peak A (m/sec) | 0.60 ± 0.19 | 0.55 | <.001 |
LV E/e′ | 10.1 ± 5.0 | 0.62 | <.001 |
LV and RV Characteristics
LV systolic function (LV ejection fraction and s′ velocity), although within the normal range, demonstrated a small yet significant reduction with age ( Table 1 ). Age-related LV diastolic changes were observed, with reductions in peak E and e′ velocities and an increase in E/e′; an increase in indexed maximum left atrial volume was observed.
RV length and RV mid diameter decreased with a corresponding increase in RV basal diameter with age, and the changes persisted despite correction for BSA. No significant change in RV area, FAC, or Tei index was seen with age ( Table 2 ). Both TAPSE and RV s′ velocity decreased with age, albeit with values within the normal range. RV s′ was higher than LV s′ velocity. Transtricuspid peak E velocity, E/A ratio, and RV lateral e′ velocity decreased with age. The RV E/e′ ratio remained unchanged with age, as did RA area. Pulmonary pressures and PVR, although within normal limits, increased with age ( Table 2 ).
Variable | Mean ± SD | r | P |
---|---|---|---|
RV length (mm) | 71.1 ± 8.0 | −0.25 | .003 |
Indexed RV length (mm/m 2 ) | 39.5 ± 4.5 | −0.26 | .002 |
RV basal diameter (mm) | 31.9 ± 4.4 | 0.33 | .001 |
Indexed RV basal diameter (mm/m 2 ) | 17.8 ± 2.6 | 0.35 | <.001 |
RV mid diameter (mm) | 26.8 ± 4.1 | −0.17 | .04 |
Indexed RV mid diameter (mm/m 2 ) | 14.9 ± 2.7 | −0.17 | .05 |
RV EDA (cm 2 ) | 16.0 ± 3.3 | −0.10 | NS |
RV ESA (cm 2 ) | 9.3 ± 2.4 | −0.18 | NS |
RV FAC (%) | 42.4 ± 6.4 | 0.04 | NS |
TAPSE (mm) | 22.1 ± 2.3 | −0.44 | <.001 |
Doppler Tei index | 0.31 ± 0.06 | 0.04 | NS |
RV s′ (cm/sec) | 11.9 ± 1.5 | −0.51 | <.001 |
RV e′ (cm/sec) | 10.8 ± 2.4 | −0.36 | <.001 |
RV a′ (cm/sec) | 10.9 ± 2.7 | 0.50 | <.001 |
Tricuspid E (cm/sec) | 48.2 ± 9.8 | −0.37 | <.001 |
Tricuspid E/A ratio | 1.5 ± 0.4 | −0.29 | .003 |
RV E/e′ | 3.9 ± 1.8 | 0.03 | NS |
RA area (m 2 ) | 14.1 ± 3.2 | 0.16 | NS |
Pulmonary pressure (mm Hg) | 21.4 ± 7.4 | 0.43 | <.001 |
PVR (Wood units) | 1.3 ± 0.4 | 0.36 | <.001 |
RV wall thickness (mm) | 4.3 ± 1.0 | 0.08 | NS |
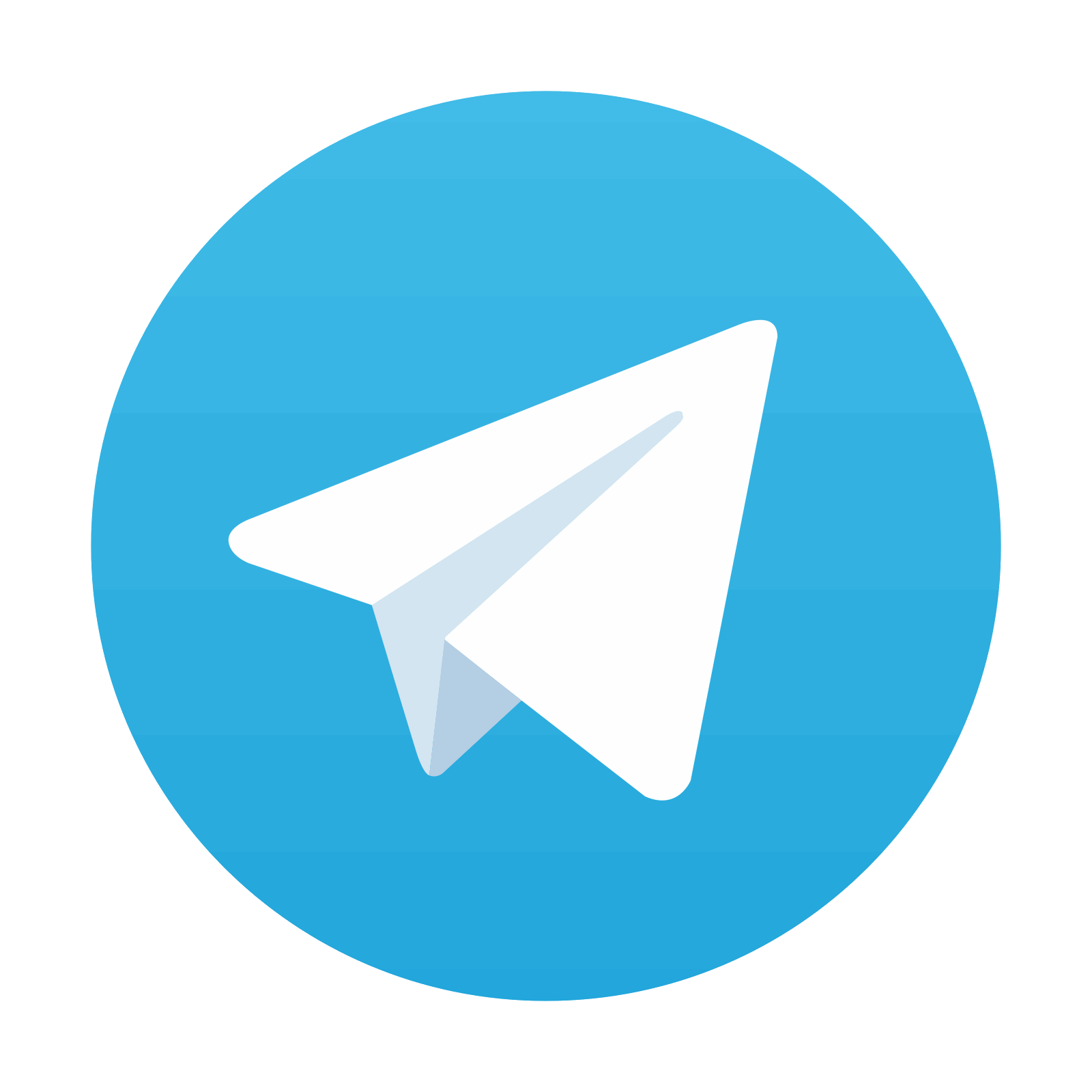
Stay updated, free articles. Join our Telegram channel

Full access? Get Clinical Tree
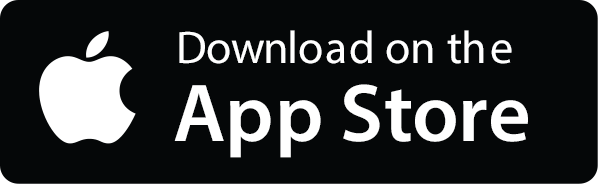
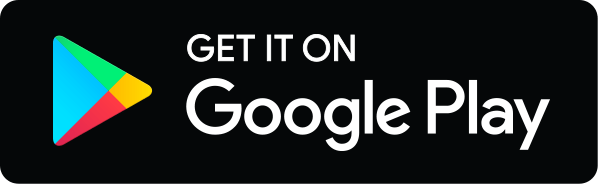
