Pleiotropic effects of statins have not been fully elucidated. Recently we demonstrated that cholesterol expands when crystallizing and may trigger plaque rupture. The present study evaluated the potential direct effects of statins in altering cholesterol crystallization as a possible mechanism for plaque stabilization independent of cholesterol lowering. Cholesterol powder was dissolved in oil with and without pravastatin, simvastatin, or atorvastatin (10 to 90 mg) and then allowed to crystallize to measure peak volume expansion (ΔVE) in graduated cylinders. Effect of ΔVE on fibrous membrane damage was also evaluated. Human coronary, carotid, and peripheral arterial plaques (65 plaques from 55 patients) were incubated with statin or saline solution using matched plaque segments to evaluate direct effects of statins on preformed crystals. Also, the effect of in vivo use of oral statins on crystal structure was examined by scanning electron microscopy and crystal content in plaques scored from 0 to +3. For all statins, ΔVE decreased significantly in a dose-dependent fashion (0.76 ± 0.1 vs 0 ml at 60 mg, p <0.001). By scanning electron microscopy crystal structure with statins had loss of pointed tip geometries, averting fibrous membrane damage. Cholesterol crystal density was markedly decreased and appeared dissolved in human plaques incubated with statins (+2.1 ± 1.1 vs +1.3 ± 1.0, p = 0.0001). Also, plaques from patients taking oral statins compared to controls had significantly more dissolving crystals (p = 0.03). In conclusion, statins decreased ΔVE by altering cholesterol crystallization and blunting sharp-tipped crystal structure and dissolving cholesterol crystals in human arteries in vivo and in vitro, providing plaque stabilization.
In a recent study we demonstrated that crystallization of cholesterol from a liquid to a solid state resulted in volume expansion (VE). When this occurs in the confined space of an arterial plaque, it can lead to rupture. The process is rapid and driven by pointed shards of cholesterol crystals that can cut through fibrous membranes. Also, we demonstrated that cholesterol crystals abundantly perforated the plaque and intima at the site of plaque rupture in humans with acute coronary and cerebrovascular syndromes. Moreover, ethanol used in tissue preparation for histology dissolved cholesterol crystals, obscuring their potential deleterious effects. Given our observation of ethanol dissolving cholesterol crystals that are critical in perforating plaques during rupture and thus protecting from acute cardiovascular events, we hypothesized that statins could also stabilize plaques by dissolving cholesterol crystals by a similar direct chemical mechanism. Therefore, we evaluated the effect of various statins on cholesterol crystallization to demonstrate their ability to inhibit VE, thus lowering potential damage to the overlying fibrous plaque cap and rupture.
Methods
Mixtures of purified cholesterol powder (2 g, molecular weight 386.7, 95% to 98% pure; Sigma, St. Louis, Missouri) were placed in 10-ml graduated cylinders and dissolved in corn oil at 37°C with and without statins (pravastatin, simvastatin, atorvastatin) while exposed to a water interphase (1 ml) to trigger crystallization. Experiments were repeated 3 times and results averaged for each statin dose and for pure cholesterol. Change in volume expansion (ΔVE) was calculated by subtracting the initial measured meniscus level of liquid cholesterol from the maximal peak of crystals formed after crystallization.
In experiments designed to study ΔVE in the absence of a liquid interphase, cholesterol (2 g) was melted in 10-ml graduated cylinders (Pyrex VISTA, Corning, Inc., Corning, New York) using a heat gun (HAG 1400-U, GAR-TEC, Baden, Germany) and then allowed to cool at room temperature. The experiment was conducted by mixing different doses (0 to 90 mg) of pravastatin, simvastatin, or atorvastatin with the cholesterol powder.
Another set of 3 control experiments at 37°C and 22°C were conducted using sodium chloride (NaCl; 30, 50, and 90 mg) mixed with cholesterol to demonstrate if the effects were specific to statins.
Doses of statins used in these experiments were comparable to those that arterial plaques are usually exposed to in humans. This was calculated from the concentration of statin in the human circulation after a single dose of atorvastatin 40 mg and a maximum serum concentration (C max ) (nanograms per milliliter) of 50.1 ng/ml. Because the average amount of free cholesterol present in a disrupted plaque is 30 mg/g and a cardiac output of 5 L/min, 0.3 mg of atorvastatin comes into contact with free cholesterol at 30 mg/min. Our experimental doses of atorvastatin (20 to 40 mg/cholesterol 2 g) were equivalent to atorvastatin 0.3 to 0.6 mg, which are similar to in vivo human exposure. By mixing cholesterol 2 g with atorvastatin 40 mg, the amount of atorvastatin present with cholesterol 30 mg in the test tube was 0.6 mg. For atorvastatin 20 mg, the amount of exposure was 0.3 mg/cholesterol 30 mg. Similar calculations were made for pravastatin 20 mg (0.3 mg/30 mg) and simvastatin 40 mg (0.6 mg/30 mg) and these doses were within physiologic levels.
Scanning electron microscopy (SEM) of cholesterol crystals was performed after crystallization by removing crystals from cylinders and placing on a filter mesh, washing with distilled water, and drying for 12 hours in a vacuum chamber (Speed Vac SC110, Savant Instruments, Inc., Farmingdale, New York) evacuated by a pump (VP110, Franklin Electric, Blufftun, Indiana). A sample of crystals was then transferred to stubs lined with carbon adhesive and gold coated in a sputter coater (EMSCOPE SC500, Emscope, Ashford, United Kingdom). Surfaces were then examined using a JEOL scanning electron microscope (Model JSM-6300F, JEOL, Ltd., Tokyo, Japan). The structure of cholesterol crystals with and without statins was then compared. Cholesterol crystal dissolving was defined as crystals that had lost their needle-tipped and sharp-edge geometry with bending, eroding, fragmenting, and merging into globs.
Assessment of fibrous membrane (mesentery and pericardium) rupture by cholesterol crystallization and VE was performed in the presence or absence of statins. Cholesterol powder 3 g were placed in 4-ml glass test tubes and melted with a heat gun. The fibrous membrane was spread snugly and tied over the orifice of the cooling tube with crystallizing cholesterol at room temperature. Four New Zealand white rabbits were euthanized (Euthasol 1 ml intravenously, DelMarva Laboratories, Midlothia, Virginia) and mesenteric membranes and pericardium were dissected and removed. These fibrous tissues were chosen because of similar composition and thickness to the plaque cap. After crystallization, membrane surfaces were inspected visually and photographed under a dissecting microscope (Wild M7, Heerbrugg, Switzerland; with EOS-Digital Rebel digital camera, Cannon, Tokyo, Japan). Fibrous membranes were then prepared for SEM as previously reported. Animal procedures were performed according to Michigan State University’s animal care and use committee approved protocol in accord with National Institutes of Health guidelines.
Human arterial plaques from coronary arteries were obtained at autopsy from plaque rupture sites of patients who had died from acute myocardial infarction, as previously described. Carotid and femoral artery plaques were obtained from patients undergoing carotid endarterectomy or peripheral bypass surgery. Demographic data were reported by the pathologist’s or surgeon’s office. These were unidentified materials obtained according to Sparrow Hospital Institutional Research Review Committee and Michigan State University institutional review board protocols.
Fresh plaques were cut in 1/2 and each placed in a Petri dish. Matching pieces were incubated in phosphate buffered saline (PBS) or in PBS with a statin (pravastatin 2 mg/ml, simvastatin 10 mg/ml, atorvastatin 10 mg/ml) at 37°C for 48 hours. After incubation, specimens were washed with distilled water, fixed overnight in 4% glutaraldehyde with phosphate buffer (pH 7.4) 0.1 mol/L, and then dehydrated by vacuum for scanning electron microscopic examination. Matching samples treated with statin or untreated were then compared for the amount of crystals present by SEM as previously reported. Briefly, cholesterol crystal content by SEM was scored from 0 to +3, where 0 indicated no crystals, +1 indicated few scattered crystals, +2 indicated extensive crystals in isolated sites, and +3 indicated extensive sheets of crystals covering the entire intimal surface. Two investigators independently read the scanning electron microscopic images and were blinded to a patient’s clinical status. A third reader adjudicated differences.
For light microscopy, fixed tissue specimens were cut and embedded in paraffin on edge. Serial tissue sections (5 μm thick) were then mounted on glass slides. Sections were then stained with hematoxylin and eosin or Masson trichrome stains and examined using a light microscope (Ernst Leitz, GmbH, Wetzlar, Germany).
Statistical analysis was performed using InStat 3 (GraphPad, San Diego, California) and SPSS 17 (SPSS, Inc., Chicago, Illinois). All continuous variables were represented as mean ± SD. One-way analysis of variance with Tukey–Kramer multiple comparison post hoc tests were performed to compare ΔVEs after addition of various doses of statins. Student’s t test was used to compare ΔVEs of cholesterol with NaCl versus pure cholesterol. Also, cholesterol crystal content from the matching segments was compared between those incubated in PBS and in PBS with statin using paired t test. Unpaired t test was used to compare cholesterol crystal content in plaques of patients taking oral statins to those not on statins. Pearson chi-square test was used to evaluate the presence or absence of crystal dissolving in control specimens with and without oral use of statins. A p value <0.05 was used as the criterion for statistical significance.
Results
Effect of statins on cholesterol crystallization was evident by a significant dose-dependent suppression of ΔVE during crystallization ( Figures 1 and 2 ). Compared to statin 0 mg in cholesterol 2 g, the lowest dose that significantly decreased ΔVE was pravastatin, simvastatin, or atorvastatin 10 mg. The dose that decreased ΔVE by 50% was pravastatin 40 mg, simvastatin 20 mg, and atorvastatin 10 to 20 mg.
Similarly, ΔVE was significantly decreased by statins in a dose-dependent fashion for all statins at 37°C. Compared to statin 0 mg in cholesterol 2 g, the lowest dose that significantly decreased ΔVE was pravastatin, simvastatin, or atorvastatin 10 to 20 mg. The dose that decreased ΔVE by 50% was pravastatin 30 to 40 mg, simvastatin 30 mg, and atorvastatin 20 mg ( Figure 3 ).
At room temperature ΔVE for cholesterol 2 g and statin 0 mg versus NaCl 30, 50, and 90 mg was not significantly different (0.45 ± 0.05 vs 0.45 ± 0.05, 0.48 ± 0.03, and 0.48 ± 0.04 ml, p = NS). Similar results were obtained at 37°C (0.45 ± 0.05 vs 0.45 ± 0.05, 0.48 ± 0.03, and 0.48 ± 0.04 ml, p = NS).
By SEM 2 predominant crystal structures were noted, i.e., elongated needle-tipped crystals and flat rhomboid-like crystals ( Figure 4 ). These were identical to crystal structures seen in human plaques. In the presence of statins, including lower doses, crystal structure was markedly altered with loss of pointed tip geometry. Moreover, with statin treatment, there was consistent breakdown and dissolving of crystals resulting in melted globs and piles of fragmented crystals ( Figure 4 ). In the presence of NaCl, crystal structure was similar to that seen with pure cholesterol.
Crystallizing cholesterol expanded from the bottom of the test tube upward and extended beyond the tube orifice within 2 minutes of melting. The fibrous membrane at the tube orifice was visibly perforated and torn by protruding crystals. These findings were noted in all 3 tubes. When the same experiment was repeated in the presence of pravastatin (30, 60, or 90 mg), only minimal expansion (≤0.17 ml) occurred, resulting in little to no visible damage to the fibrous membranes ( Figure 5 ).
Effect of statins was examined in 65 plaques from 55 human coronary, carotid, and femoral arteries that were cut in equal matching halves ( Table 1 ). One-half was incubated in PBS with statin at 37°C for 48 hours and the other 1/2 in PBS only. Cholesterol crystals in statin-treated plaque segments demonstrated dissolving and breakdown of crystals as was seen in vitro for pure crystals treated with statins ( Figure 6 ). Also, after statin incubation residual intimal holes were noted at sites of dissolving crystals ( Figure 6 ). In all 65 plaques, total number of crystals was significantly decreased for each statin and for all statins combined compared to plaque segments treated with PBS only and statin-incubated plaque segments ( Table 2 ).
Arteries Studied | Cases | Men | Oral Statins | Clinical Presentation | Plaque Disruption |
---|---|---|---|---|---|
Coronary | 4 | 2 (50%) | unknown | acute myocardial infarction 4 (100%) | 4 (100%) |
Carotid | 43 | 20 (47%) | 32 (74%) | neurologic symptoms 35 (81%) | 36 (84%) |
Femoral | 8 | 6 (75%) | 4 (50%) | claudication 8 (100%) | 7 (88%) |
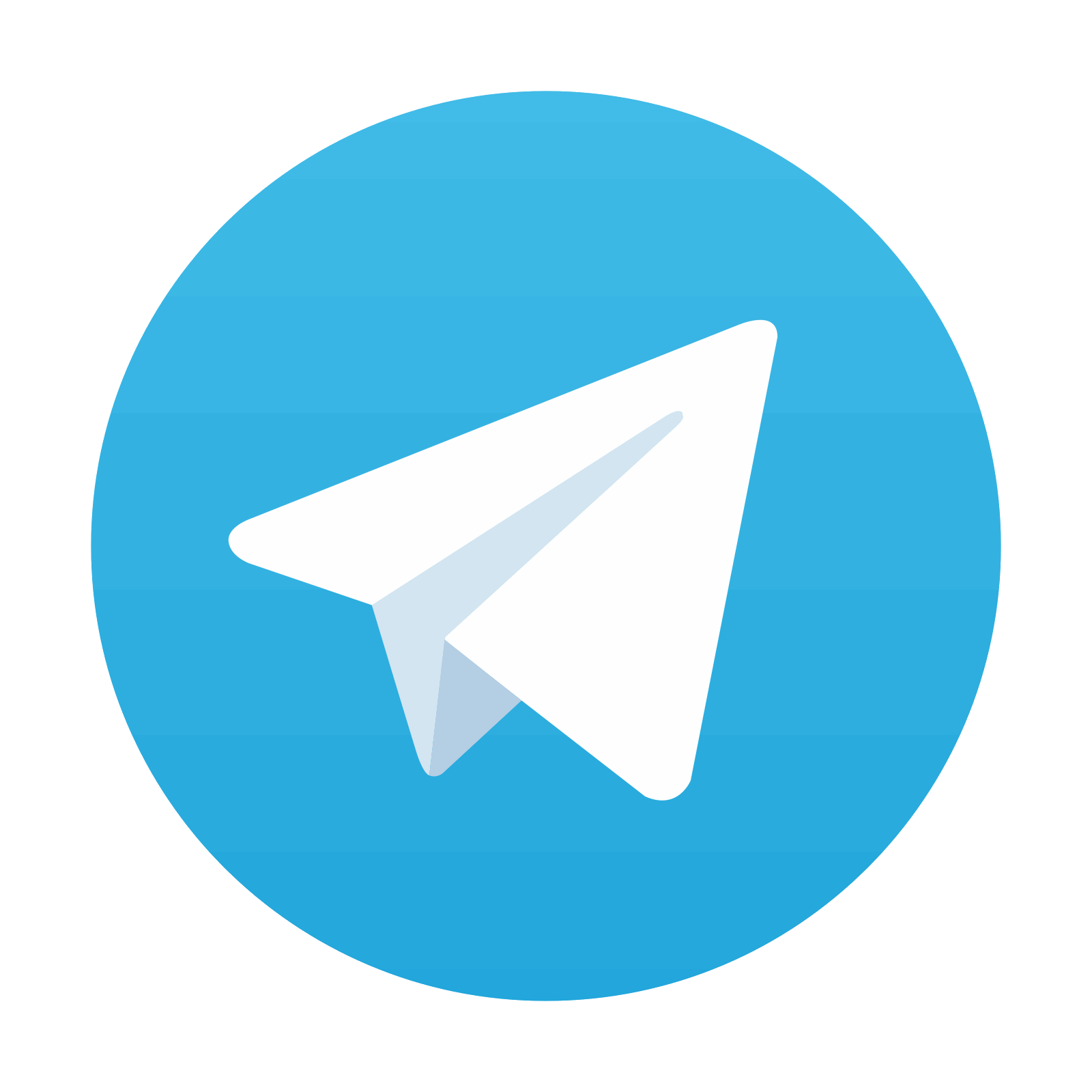
Stay updated, free articles. Join our Telegram channel

Full access? Get Clinical Tree
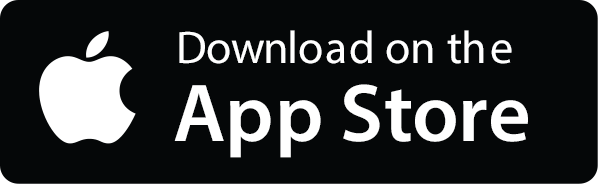
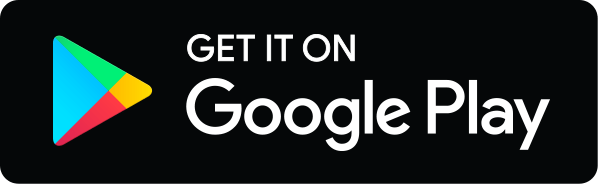
