Effect of Right Ventricular Apical Pacing and Cardiac Resynchronization Therapy on Left Ventricular Dyssynchrony and Function
Laurens F. Tops
Martin J. Schalij
Jeroen J. Bax
Since the implantation of the first permanent pacemaker in 1958, cardiac pacing has been an effective treatment in the management of patients with bradyand tachyarrhythmias.1 Although new indications for pacing such as drug-refractory heart failure have been introduced,2 sick sinus syndrome and atrioventricular conduction disorders still remain the most important indications for cardiac pacing.3
In general, the endocardial pacing lead is positioned at the right ventricular (RV) apex. However, concerns about longterm effect of pacing at the RV apex have been raised.4 The abnormal electrical and mechanical activation pattern of the ventricles caused by RV apical pacing may have detrimental effects on cardiac structure and function.5 Therefore, in patients with sinus-node disease but with preserved atrioventricular conduction, atrial pacing may be preferred.6 However, in patients with congenital or acquired atrioventricular block, ventricular pacing is inevitable. To preserve cardiac function, biventricular pacing (either “upgrade” from previous RV pacing or “de novo”) may be preferred over RV apical pacing alone in some of these patients.
The detrimental effects of RV apical pacing will be discussed in this chapter. In particular, the evidence as provided by large randomized clinical trials on pacing mode selection and the preclinical and clinical data on the pathophysiology of these negative effects will be reviewed. In addition, the issue of “upgrading” conventional RV apical pacing to biventricular pacing, and the effects on left ventricular (LV) dyssynchrony and function, will be addressed. Finally, the available studies comparing RV apical pacing and biventricular pacing will be reviewed.
RIGHT VENTRICULAR APICAL PACING
For decades, the RV apex has been used as the preferred location to position cardiac pacing leads. The addition of an atrial pacing lead (dual-chamber pacing) may result in more “physiologic” pacing, but the optimal pacing mode in the various pacemaker populations still remains controversial. Large randomized clinical trials have provided important information for selection of the optimal pacing mode.7 But more importantly, these trials have revealed an association between RV pacing and cardiac morbidity (including atrial fibrillation and heart failure) and mortality. In addition, numerous clinical and preclinical studies have investigated the exact effects of RV apical pacing. Importantly, it has been suggested that in some patients, the negative effects of RV apical pacing may be more pronounced than in others. In the following paragraphs the evidence that several large clinical trials on the selection of pacing mode have provided will be discussed. In addition, the pathophysiology of the detrimental effects of RV apical pacing will be reviewed.
Randomized Clinical Trials on Right Ventricular Pacing
Several large, randomized clinical trials have compared dualchamber and ventricular pacing modes in various pacemaker populations.8 In the MOde Selection Trial (MOST), 2,010 patients with sinus node dysfunction were randomized between single chamber ventricular (VVIR) rate modulated pacing versus dual chamber (DDDR) rate modulated pacing.9 After a median of 33 months, no significant difference was observed between the two groups with regard to the primary end-point of all-cause death or nonfatal stroke (VVIR 23.0% vs. DDDR 21.5%, p = 0.48). Interestingly, a substudy of the MOST trial revealed a strong association between RV pacing and the risk of heart failure hospitalization and atrial fibrillation.10 The substudy comprised 1,339 patients (DDDR: n = 707, VVIR: n = 632) with baseline QRS duration <120 ms and available data on cumulative percentage of pacing during follow-up. The median percentage of cumulative pacing was significantly higher in the DDDR than in the VVIR group (90% vs. 58%, p<0.001). In both pacing mode groups, a higher cumulative percentage of pacing was associated with a worse outcome
(Fig. 9.1). It was noted that >40% of ventricular pacing in the DDDR group was associated with an increased risk of heart failure hospitalization (hazard ratio 2.60; 95% CI 1.05 – 6.47; p <0.05) and that >80% of ventricular pacing in the VVIR group was associated with an increased risk of heart failure hospitalization (hazard ratio 2.50; 95% CI 1.44 – 4.36; p <0.05). It was therefore concluded that RV pacing increases the risk of heart failure, even when atrioventricular synchrony is preserved using physiologic DDDR pacing.
(Fig. 9.1). It was noted that >40% of ventricular pacing in the DDDR group was associated with an increased risk of heart failure hospitalization (hazard ratio 2.60; 95% CI 1.05 – 6.47; p <0.05) and that >80% of ventricular pacing in the VVIR group was associated with an increased risk of heart failure hospitalization (hazard ratio 2.50; 95% CI 1.44 – 4.36; p <0.05). It was therefore concluded that RV pacing increases the risk of heart failure, even when atrioventricular synchrony is preserved using physiologic DDDR pacing.
Interestingly, in other patient populations the detrimental effects of RV pacing have also become clear. The Dual Chamber and VVI Implantable Defibrillator (DAVID) trial randomized patients with a standard indication for a defibrillator implantation, but without an indication for antibradycardia pacing, between “physiologic pacing” (DDDR mode, lower rate of 70 bpm) or ventricular back-up pacing (VVIR mode, lower rate of 40 bpm).11 At baseline, the majority of the patients had NYHA functional class I or II, mean LV ejection fraction was 27%, and mean intrinsic QRS duration was 120 ms (31% of the patients had QRS >130 ms). The combined primary end-point was freedom from death and absence of hospitalization for new or worsened heart failure. After a median follow-up of 8.4 months, the primary outcome measure was lower in the VVIR-40 group than in the DDDR-70 group (relative hazard 1.61; 95% CI 1.06 – 2.44; p = 0.03). Interrogation of the defibrillator device revealed a significantly higher percentage of ventricular paced beats in the DDDR-70 group at 3 months follow-up (57.9 ± 35.8% vs. 1.5 ± 8.0%, p<0.001). Importantly, a trend toward a worse survival rate at 12 months was noted in patients with a high percentage of pacing at 3 months’ follow-up. From the DAVID trial, it was concluded that “physiologic” DDDR pacing provided no clinical benefit over VVIR back-up pacing in patients with standard indications for a defibrillator.11
Intuitively, atrial-based pacing may be superior over RV apical pacing in selected patient groups, because it allows normal ventricular electrical activation and thus prevents cardiac “desynchronization.” However, large randomized trials have not been able to consistently demonstrate an improved outcome of atrial-based pacing as compared with ventricular pacing. Recently, a meta-analysis was performed including more than 7,000 patients randomized between atrial-based (AAI or DDD) and ventricular pacing.12 Five randomized clinical trials were included with an average follow-up duration ranging from 2.5 to 6.0 years (total of nearly 35,000 patient-years of follow-up). Using individual patient data, no significant reduction in mortality was found with atrial-based pacing (hazard ration 0.95; 95% CI 0.87 – 1.03; p = 0.19). In addition, no differences were found in the composite end-point of stroke or cardiovascular death, stroke, and heart failure hospitalization between the different pacing modes (Fig. 9.2). However, a significant reduction in atrial fibrillation was noted with atrialbased pacing (hazard ratio 0.80; 95% CI 0.72 – 0.89; p<0.001).
In the previously mentioned randomized trials and metaanalysis, no benefit or only modest clinical benefit of “physiologic” DDDR pacing over VVIR pacing could be demonstrated. This may be explained by the higher percentage of ventricular pacing in the DDDR groups, as a result of the short programmed atrioventricular interval. Thus, the beneficial effect of maintaining atrioventricular synchrony by “physiologic” DDDR pacing may be reduced by the deleterious effects of RV apical pacing itself. Recently, new pacing algorithms have been
introduced to minimize RV pacing. These algorithms promote atrioventricular conduction and target maintenance of intrinsic ventricular conduction and avoidance of ventricular desynchronization.13,14 It has been demonstrated that the use of atrioventricular search hysteresis was associated with similar outcomes compared with VVIR backup pacing.13 Recently, the Search AV Extension and Managed Ventricular Pacing for Promoting Atrioventricular Conduction Trial (SAVE PACe) randomized 1,065 patients with sinus node disease and intact atrioventricular conduction between conventional dualchamber pacing and dual-chamber minimal ventricular pacing.14 Using the minimal RV pacing algorithm, the percentage of paced ventricular beats was reduced significantly, as compared with conventional dual-chamber ventricular pacing (9.1 vs. 99.0%, p <0.001). Although the primary end-point was met (development of persistent atrial fibrillation: 7.9% in minimal RV pacing vs. 12.7% in conventional dual-chamber pacing, p = 0.004), there was no significant difference in mortality or heart failure hospitalizations between the two groups.14
introduced to minimize RV pacing. These algorithms promote atrioventricular conduction and target maintenance of intrinsic ventricular conduction and avoidance of ventricular desynchronization.13,14 It has been demonstrated that the use of atrioventricular search hysteresis was associated with similar outcomes compared with VVIR backup pacing.13 Recently, the Search AV Extension and Managed Ventricular Pacing for Promoting Atrioventricular Conduction Trial (SAVE PACe) randomized 1,065 patients with sinus node disease and intact atrioventricular conduction between conventional dualchamber pacing and dual-chamber minimal ventricular pacing.14 Using the minimal RV pacing algorithm, the percentage of paced ventricular beats was reduced significantly, as compared with conventional dual-chamber ventricular pacing (9.1 vs. 99.0%, p <0.001). Although the primary end-point was met (development of persistent atrial fibrillation: 7.9% in minimal RV pacing vs. 12.7% in conventional dual-chamber pacing, p = 0.004), there was no significant difference in mortality or heart failure hospitalizations between the two groups.14
From all the large trials, it has become apparent that ventricular pacing, even in the setting of “physiologic” dualchamber pacing, is associated with an increased risk of adverse events. However, in daily clinical practice not all patients who receive RV apical pacing will experience these adverse events. It appears that some patients are more susceptible to the detrimental effects of RV apical pacing than others, probably related to underlying structural heart disease. In general, the negative effects of RV apical pacing may be explained by the abnormal electrical and mechanical activation pattern during RV apical pacing. In the following paragraphs, the underlying pathophysiology of the detrimental effects of RV apical pacing will be discussed.
Detrimental Effects of Right Ventricular Apical Pacing
Already in 1925 it was recognized by Wiggers that ventricular pacing resulted in reduced ventricular pump function in mammals.15 Since then, this observation has been confirmed in numerous other animal studies.16 In general, these negative effects have been attributed to the abnormal electrical and mechanical activation pattern of the ventricles induced by RV apical pacing. During RV pacing, the conduction of the electrical wave front propagates through the myocardium, rather than through the His-Purkinje conduction system. As a result, the electrical wave front propagates more slowly and
induces heterogeneity in electrical activation of the myocardium. Using endocardial mapping of the LV in 40 patients, Vassallo et al. demonstrated that the electrical activation pattern during RV apical pacing is very similar to left bundle branch block.17 A single breakthrough was located in the interventricular septum in the majority of the patients. In addition, the site of latest activation was predominantly located at the inferoposterior base of the LV, with a total endocardial activation time similar to left bundle branch block.17
induces heterogeneity in electrical activation of the myocardium. Using endocardial mapping of the LV in 40 patients, Vassallo et al. demonstrated that the electrical activation pattern during RV apical pacing is very similar to left bundle branch block.17 A single breakthrough was located in the interventricular septum in the majority of the patients. In addition, the site of latest activation was predominantly located at the inferoposterior base of the LV, with a total endocardial activation time similar to left bundle branch block.17
Similar to the changes in electrical activation of the ventricles, the mechanical activation pattern of the LV is changed during RV apical pacing. Importantly, not only the onset of mechanical contraction is changed, but also the pattern of mechanical contraction.16 In several studies using animal models, it has been demonstrated that the early-activated regions near the pacing site exhibit rapid early systolic shortening, resulting in pre-stretch of the late activated regions.18,19 As a consequence, these regions exhibit an increase in (delayed) systolic shortening, imposing systolic stretch to the early activated regions exhibiting premature relaxation. This abnormal contraction pattern of the various regions of the LV may result in a redistribution of myocardial strain and work and subsequent less effective contraction.19
Both the abnormal electrical and mechanical activation patterns of the ventricles negatively affect cardiac function. An overview of the effects of RV apical pacing on cardiac function is provided in Figure 9.3. The most important effects are related to cardiac metabolism and perfusion, remodeling, hemodynamics, and mechanical function. The effects on cardiac metabolism and perfusion have been clearly demonstrated in an animal model of cardiac pacing, where it was noted that the abnormal activation pattern with paradoxical stretching of the different regions within the LV resulted in significant changes in regional myocardial perfusion.20 Similarly, it has been demonstrated that RV apical pacing may negatively influence LV perfusion. Even in the absence of coronary artery disease, myocardial perfusion defects may be present in up to 65% of the patients after long-term RV pacing.21,22 It has been noted that the perfusion defects are mainly located near the pacing site (inferior and apical segments) and tend to increase with time.22
In addition, cardiac pacing may result in regional and global LV remodeling. Changes in cardiac structure ranging from the cellular to macroscopic level have been noted after long-term cardiac pacing. Karpawich et al.23 demonstrated histological changes in 14 patients with congenital complete
atrioventricular block, requiring RV pacing. Endomyocardial biopsies were acquired after long-term (median 5.5 years) permanent RV pacing. In these patients, cellular and intracellular alterations were noted, including mitochondrial variations and degenerative fibrosis.23
atrioventricular block, requiring RV pacing. Endomyocardial biopsies were acquired after long-term (median 5.5 years) permanent RV pacing. In these patients, cellular and intracellular alterations were noted, including mitochondrial variations and degenerative fibrosis.23
Furthermore, RV pacing may result in asymmetrical remodeling of the LV. In an animal model of cardiac pacing, it was demonstrated that LV wall thickness may decrease in early-activated regions, whereas it may increase in lateactivated regions (Fig. 9.4), accompanied by a change in myocyte thickness.24 Finally, global remodeling resulting in an increase in LV volumes may occur after long-term pacing. Both in animal24 and in human25 studies, changes in LV size have been reported. Importantly, severe LV remodeling may subsequently lead to functional mitral regurgitation and left atrial remodeling.
In addition, the abnormal electrical and mechanical activation of the LV may result in changes in hemodynamic properties and global mechanical function. Pacing at the RV apex may result in a decrease in cardiac output and may change LV filling properties.26 Finally, changes in myocardial strain and timing of regional strain may occur during RV apical pacing. Using magnetic resonance imaging in an animal model of cardiac pacing, Prinzen et al. demonstrated that regional myocardial strain is changed during pacing.19 A significant decrease in strain was observed in the regions close to the pacing site, whereas an increase in myocardial strain was observed in remote regions. Importantly, timing of peak regional strain is also changed during pacing. This is often referred to as “mechanical dyssynchrony.”27 The presence of LV dyssynchrony after long-term RV apical pacing has been related to a decrease in LV systolic function and deterioration in functional capacity.28 However, not all patients will develop significant mechanical dyssynchrony after long-term RV apical pacing. In the following paragraphs, the assessment of ventricular dyssynchrony with echocardiography and the relation with RV apical pacing will be discussed.
MECHANICAL DYSSYNCHRONY IN RIGHT VENTRICULAR APICAL PACING
Transthoracic echocardiography is the most widely used technique to assess the extent of ventricular dyssynchrony. Right ventricular apical pacing may induce both interventricular dyssynchrony (between the RV and the LV), as well as intraventricular dyssynchrony (within the LV) in some patients. Various echocardiographic techniques are available for the assessment of cardiac mechanical dyssynchrony. These include conventional Doppler techniques, tissue Doppler imaging, strain analysis, and novel three-dimensional echocardiography. The majority of the techniques have been used to quantify inter- and intraventricular dyssynchrony in heart failure patients referred for CRT. Using echocardiography, the prediction of a favorable response to CRT may be improved, rather than using the conventional selection criteria (severe heart failure, LV ejection fraction <35%, QRS >120 ms) alone.29 Similarly, these techniques can be used to detect the presence of ventricular mechanical dyssynchrony during acute and long-term RV apical pacing.
Interventricular Dyssynchrony
For the assessment of interventricular dyssynchrony (mechanical dyssynchrony between the RV and the LV), conventional Doppler techniques are typically used. For both ventricles, the electromechanical delay is calculated as the time from QRS to the onset of pulmonary systolic flow (RV electromechanical delay) or aortic systolic flow (LV electromechanical delay). The time difference between the RV and LV electromechanical delay represents interventricular dyssynchrony (Fig. 9.5). Previously, a difference of ≥40 ms in electromechanical delay between the RV and the LV has been used to indicate the presence of interventricular dyssynchrony.30,31
It has been demonstrated that RV apical pacing is associated with an increase in interventricular dyssynchrony. In 33 patients with a DDDR pacemaker for atrioventricular block or sinus node dysfunction, the effect of RV apical pacing on interventricular dyssynchrony was investigated.32 After longterm RV apical pacing, an echocardiogram was performed during intrinsic rhythm and during RV pacing. An increase in interventricular dyssynchrony was observed, most prominent in patients with an LV ejection fraction <35% (mean interventricular delay increased from 22 ± 17 to 58 ± 14, p <0.001). During RV apical pacing, 15 of the 33 patients (45%) exhibited significant interventricular dyssynchrony.32
Intraventricular Dyssynchrony
Several echocardiographic techniques are available for the assessment of LV dyssynchrony. The assessment of intraventricular dyssynchrony with echocardiography has mainly been used prior to CRT. However, all these techniques have also been used to study the effects of RV apical pacing in various pacemaker populations.
Septal-to-Posterior Wall Motion Delay
This parameter is derived from conventional echocardiography and represents the delay in mechanical activation between the interventricular septum and the posterior wall of the LV. Typically, an M-mode recording from the parasternal short-axis view at the level of the papillary muscles is used to evaluate the septal-to-posterior wall motion delay. The interval between the maximal posterior displacement of the septum and the maximal inward displacement of the posterior wall is calculated. Previously, a septal-to-posterior wall motion delay ≥130 ms has been used to define the presence of LV dyssynchrony, and predict a favorable response to CRT.33
In 1977, Gomes et al. demonstrated the effect of RV apical pacing on the delay between the septum and the posterior wall.34 During the acute onset of cardiac pacing in 12 patients, it was noted that, during RV apical pacing, there was an early rapid pre-ejection posterior motion of the interventricular septum. In addition, the posterior wall of the LV exhibited a delayed contraction, resulting in a significant delay between the activation of the septum (61 ± 5 ms) and the posterior wall (116 ± 18 ms).34 After long-term RV apical pacing, this septal-to-posterior wall motion delay may still be present. In a retrospective cohort of 55 patients undergoing atrioventricular node ablation and subsequent permanent RV apical pacing, the effect of long-term RV pacing on the septal-toposterior wall motion delay was studied.28 It was noted that after a mean of 3.8 ± 1.7 years of RV apical pacing, the septal-to-posterior wall motion delay had significantly increased (from 63 ± 31 ms to 121 ± 64 ms, p <0.05), and 49% of the patients exhibited a significant delay between the septum and the posterior wall.
Tissue Doppler Imaging
Dedicated tissue Doppler imaging is a well-validated technique that allows assessment of myocardial velocities throughout the cardiac cycle.35 During off-line analysis, samples are placed at basal (and mid-ventricular) LV segments to detect regional peak systolic and diastolic myocardial velocities. In addition, the time needed to reach peak systolic velocities can be derived from the reconstructed myocardial velocity curves. By calculating the difference in time-to-peak systolic velocities of various LV segments, LV dyssynchrony can be calculated. In heart failure patients referred for CRT, various cut-off values have been proposed to define the presence of dyssynchrony.29
Tissue Doppler imaging has been used to study the effects of RV apical pacing on LV dyssynchrony in a number of studies.28,36,37 The acute effect of RV apical pacing on tissue Doppler-derived dyssynchrony parameters has been demonstrated in a cohort of 56 patients with conventional pacemaker indications.37 During RV pacing, a significant increase in LV dyssynchrony was observed: The median intraventricular electromechanical delay increased from 28 ms during intrinsic rhythm (interquartile range from 16 to 49 ms) to 40 ms during RV apical pacing (interquartile range from 24 to 80 ms, p <0.001). In 28 patients (50%), significant LV dyssynchrony (defined as LV electromechanical delay >41 ms) was present during RV pacing.37 Similar results have been reported after long-term RV apical pacing.28 Finally, tissue Doppler imaging may be used during the follow-up of
patients with congenital complete heart block and RV pacing, to study the extent of LV dyssynchrony.36
patients with congenital complete heart block and RV pacing, to study the extent of LV dyssynchrony.36
Strain Analysis
The assessment of myocardial strain allows differentiation between active contraction and passive motion of the myocardium. In particular during RV pacing, exact assessment of the timing of active contraction is important. Various approaches for myocardial strain analysis exist, including tissue Doppler imaging, derived strain, and novel speckle-tracking strain analysis.38 With these techniques, detailed analysis of myocardial deformation can be acquired, as well as timing of peak systolic strain. By calculating differences in time-to-peak systolic strain of various segments, intraventricular dyssynchrony can be assessed. With the use of speckle-tracking strain analysis, it has been demonstrated that a difference in time-to-peak strain between the septum and the posterior wall ≥ 130 ms is indicative of severe LV dyssynchrony, and can predict a favorable response to CRT.39
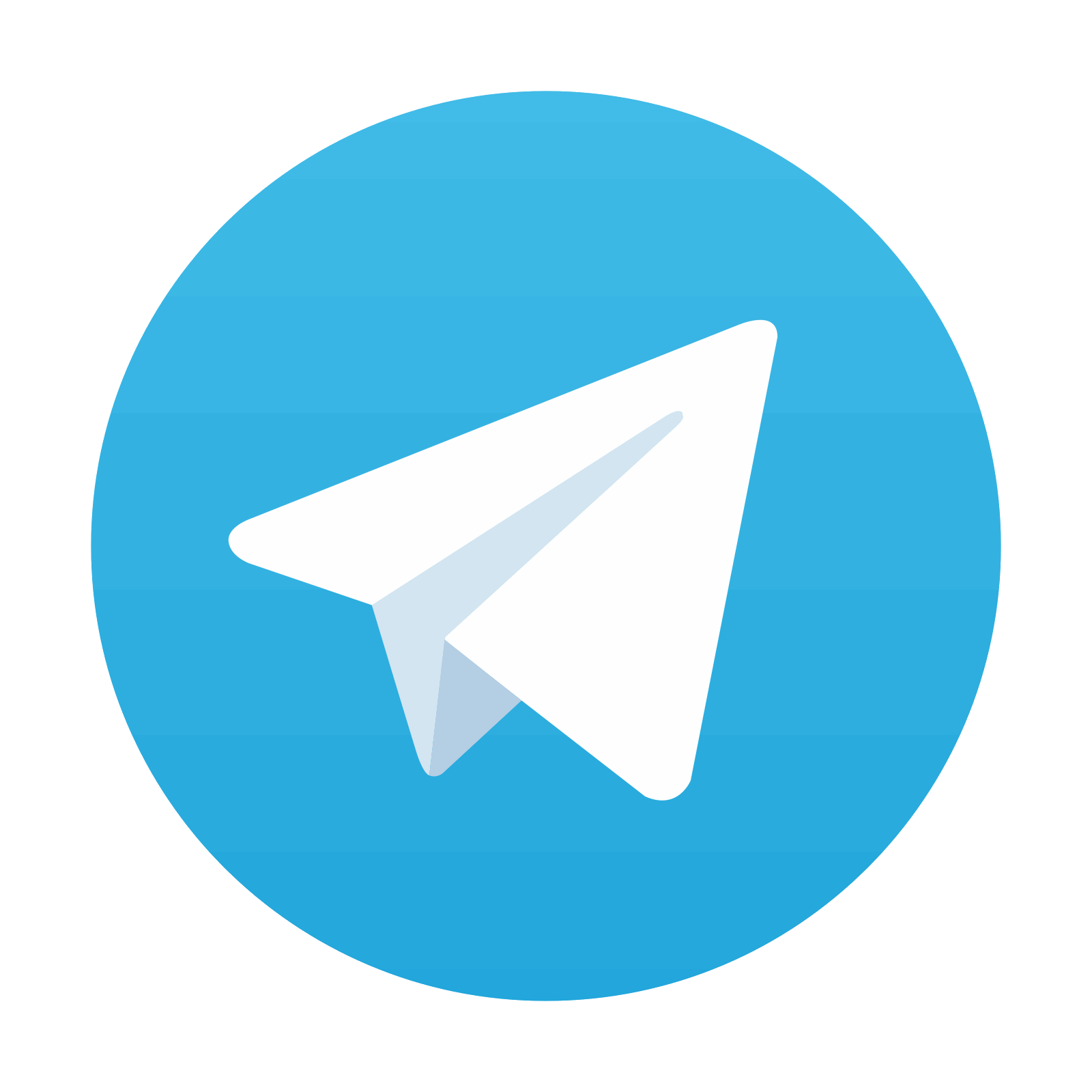
Stay updated, free articles. Join our Telegram channel

Full access? Get Clinical Tree
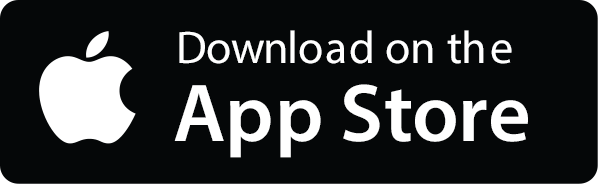
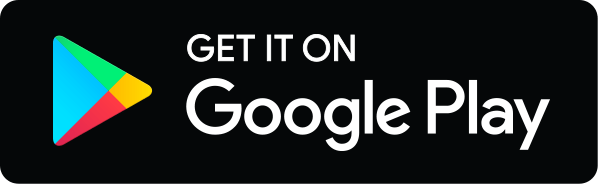
