Background
The aortic valve and the mitral valve (MV) are coupled via fibrous tissue. Simultaneous dynamic analysis of the two valves’ annuli has demonstrated that they have synchronous and reciprocal behavior. Accordingly, the aims of this study were to characterize mitral-aortic coupling (MAC) in three-dimensional space before and after MV repair and to identify the untoward effects of annuloplasty rings on MAC compared with normal valvular function.
Methods
Real-time three-dimensional transesophageal echocardiography was performed on 28 consecutive patients with degenerative MV disease and severe mitral regurgitation before and after MV repair and in 25 age-matched control subjects. Custom software was used to semiautomatically identify the mitral and aortic annuli throughout the cardiac cycle and to measure parameters describing valvular dynamics.
Results
Patients with mitral regurgitation before MV repair were characterized by altered morphology and function of the MV but preserved MAC because of the maintained ability of the mitral annulus to change size and position. MV repair together with annuloplasty ring implantation forced the mitral annulus to be smaller and less pulsatile, with decreased displacement ability compared with normal mitral annuli. Because of this alteration in MAC, the “unaffected” aortic annulus became less pulsatile and less mobile.
Conclusions
This study shows unwanted and unexpected changes in aortic annular function secondary to mitral valve repair with an annuloplasty ring due to altered MAC mechanisms. These changes may alter the dynamic mechanism of the aortic root that facilitates blood ejection, so MAC should be considered and evaluated from diagnosis to treatment in MV disease.
The aortic valve (AV) and the mitral valve (MV) are coupled via fibrous tissue. Simultaneous dynamic analysis of both valves throughout the cardiac cycle has demonstrated that they have synchronous and reciprocal dynamic behavior. However, the vast majority of studies on their coupling have been performed on each valve separately, primarily because of the lack of methods capable of analyzing the complex temporal dependency and three-dimensional (3D) relationship between the AV and the MV.
The recent introduction of real-time 3D transesophageal echocardiographic (TEE) imaging has enabled the evaluation of the dynamic behavior of the MV, providing new insights into its pathophysiology. In contrast, evaluation of the dynamics of the AV has been limited to animal models, predominantly using radiopaque markers and sonomicrometric crystals, which allow accurate measurements in three dimensions with high temporal resolution. These studies have unveiled the complex mechanisms that characterize AV dynamics, showing how the AV contributes to maximizing left ventricular ejection.
Among these mechanisms, mitral-aortic coupling (MAC) plays an important role because of the reciprocal behavior between the AV and the MV. According to previous studies, constraints imposed by valvular repair or replacement may lead to undesired and unexpected changes on the dynamics of the valve not directly involved in surgery, simultaneously altering MAC. Indeed, recent studies have observed improvements in the severity of mitral regurgitation (MR) after AV replacement, as well as a negative constrictive effect on AV dynamics after MV repair. Despite these observations, there are no studies to date focusing on the 3D evaluation and quantification of the anatomy and dynamics of MAC in patients undergoing MV repair.
We hypothesized that knowledge of the changes that occur in MAC dynamics after MV repair may help in designing more physiologic valvular prostheses and in the development of novel reparative techniques that would avoid affecting the function of either valve. Consequently, the aims of this study were (1) to characterize MAC in 3D space before and after MV repair surgery and (2) to identify the negative effects on coupled valve dynamics by comparing results after MV repair with those obtained in patients with normal valvular function.
Methods
Patient Population
We studied 28 consecutive patients (19 men; mean age, 59 ± 11 years) with degenerative MV disease and severe MR, not affected by rheumatic mitral disease (defined according to American College of Cardiology and American Heart Association 2006 recommendations ), who underwent MV repair after undergoing complete two-dimensional (2D) and real-time 3D TEE examinations.
In addition, a group of 25 age-matched control subjects (nine men; mean age, 55 ± 19 years) with normal MV anatomy were also studied. The control group consisted of patients undergoing clinically indicated TEE imaging for the assessment of a cardioembolic source of a stroke. Exclusion criteria were the presence of (1) MV stenosis; (2) MR greater than mild; (3) AV or aortic root disease; (4) atrial fibrillation and cardiac arrhythmias; (5) myocarditis, pericardial, or congenital heart disease; and (6) left ventricular ejection fraction < 55%.
Study subjects were enrolled at Centro Cardiologico Monzino (Milan, Italy) and at the University of Chicago Hospitals (Chicago, IL). The protocol was approved by the respective institutional review boards, and informed consent was obtained from all participants.
Protocol
During surgery, a standard 2D TEE examination was used to evaluate the morphology of the MV apparatus, the severity of the MR jet, and the presence of MV stenosis and systolic anterior motion of the MV. In addition, after endotracheal intubation and before establishing cardiopulmonary bypass, real-time 3D TEE imaging was performed (MR-PRE) and then repeated after the completion of MV repair (MR-POST). During surgical intervention, the TEE recordings were acquired when cardiac pressures and heart rate were stable. Preload was assed using standard echocardiography, and loading conditions were optimized to grant stable hemodynamic conditions. All patients were in sinus rhythm and were examined under deep sedation.
The surgical approach for MV repair varied slightly according to the morphology of the MV pathology and the surgeon’s evaluation and preference. Nevertheless, an annuloplasty ring was inserted in all patients to stabilize the annulus and the suture line. The surgical repair was considered unsuccessful if the echocardiographic study performed after the repair showed (1) significant residual MR (more than mild), (2) mitral stenosis (maximal mean gradient > 6 mm Hg), and (3) the presence of systolic anterior motion of the anterior MV leaflet.
Real-Time 3D TEE Imaging
Three-dimensional TEE studies were performed using the iE33 ultrasound imaging system (Philips Medical Systems, Andover, MA) equipped with a fully sampled matrix-array TEE transducer (X7-2t). The probe was positioned at the midesophageal level with a 120° tilt. Real-time 3D TEE images were acquired using the wide-angled acquisition mode, in which electrocardiographically triggered wedge-shaped subvolumes were obtained over four to seven consecutive cardiac cycles. Each acquired scan volume included the mitral apparatus, the AV, and the proximal ascending aorta.
Real-Time 3D TEE Data Analysis
The real-time 3D TEE data sets were analyzed offline using custom software designed to simultaneously quantify the dynamic behavior of the mitral annulus (MA) and aortic annulus (AoA). Briefly, this was achieved by semiautomatically detecting these structures and then automatically tracking them in 3D space throughout the cardiac cycle using optical flow and region-based matching techniques, based on the analysis of speckle noise patterns within the 3D data sets. The tracked points were displayed frame by frame throughout the cardiac cycle to visually verify their position in 3D space and to allow manual correction when necessary. Finally, several parameters were automatically measured on the detected annuli throughout the cardiac cycle. In addition, left ventricular ejection fraction, left ventricular total stroke volume (SV), and AV area (computed as the planar area of the AV orifice in the aortic root cross-sectional plane) were measured using QLAB software (Philips Medical Systems) on MR-PRE and MR-POST 3D TEE data sets.
MA Quantification
Initially, the first frame of the sequence in which the MV was visualized as closed was selected, and 15 long-axis cut planes evenly rotated around the MA center (in 12° steps) were automatically displayed. On each plane, the operator selected two points, one on each side of the MA. The same procedure was then applied to the last systolic frame, in which the MV leaflets were still closed.
After automatic tracking, the MA points were connected to obtain the annular line ( Figure 1 ). To describe the dynamic behavior of the MA, the sequence of 3D MA surfaces and annular lines were used to quantify the following parameters throughout the cardiac cycle : (1) MA surface area, as the area of the triangular mesh connecting all MA points, from which maximum and minimum MA surface area were obtained during the cardiac cycle, as well as their absolute difference; (2) MA surface area change, normalized to MA surface area at end-diastole (its maximum value represented MA pulsatility, i.e., the ability of the MA to change size); (3) MA longitudinal displacement, as the average motion of all MA points with respect to its end-diastolic position, from which the maximum motion was quantified; (4) MV height, defined as the difference between the saddle horn and the lowest MA point in the orthogonal direction to the MV plane, from which maximum and minimum MV height were obtained throughout the cardiac cycle, as well as their absolute difference; (5) MA diameters: D AP , starting from the saddle horn and passing through the MA center, together with its orthogonal diameter ( D CC ); and (6) the ratio between the 3D MA surface area and the MA surface planar projection (i.e., the 2D area obtained by geometrically projecting the MA surface on a plane).

AoA Quantification
In this study, the term “aortic annulus” refers to the line representing the insertion of the aortic cusps into the sinuses of Valsalva ( Figure 1 ). This line was used as a reference because it has the same definition that is used for the MA (i.e., the insertion of the leaflet) and is an anatomic landmark that reflects the 3D nature of the AV. In the first frame of the sequence in which the AV was visualized as closed, 15 cut planes (12° apart) passing through the axis orthogonal to the AV plane were displayed. Two AoA points were identified in each of these planes, and the positions of the coaptation point and of the interatrial septum were manually marked. The same procedure was then applied to the first frame in which the AV leaflets were visualized as closed, after the end of ejection.
The AoA points were automatically tracked throughout the cardiac cycle and connected using three splines, one for each cusp in each frame ( Figure 1 ). A point on the interatrial septum was used as the reference position to identify the noncoronary aortic cusp and thus to identify the left and right cusps.
From these contours, the following parameters were calculated frame by frame: (1) AoA area, projected on the AoA base plane, from which minimum and maximum in the cardiac cycle were extracted and used to compute AoA pulsatility (defined as the maximum minus the minimum value normalized to end-diastolic AoA area); (2) absolute and percentage variation of AoA projected area during the cardiac cycle; (3) the distance between the centers of the AoA and the MA; (4) the angle between the MA and the AoA, defined as the angle between the aortic and the mitral plane, respectively computed as the plane interpolating MA and AoA points; (5) the distance between the MA center and the AoA center with the saddle horn; and (6) AoA 3D displacement, from which the displacements in the aortic root axial and left ventricular longitudinal directions were calculated (dashed line in Figure 2 ).

Statistical Analysis
Computed parameters were averaged for all studied patients and are presented as mean ± SD. For each parameter, the data distribution normality (Kolmogorov-Smirnov) test was used. To test for presurgical differences between the control group and the MR-PRE patients, unpaired t tests or Mann-Whitney U tests were applied for normally or not normally distributed data, respectively. To study the effect of annuloplasty, differences between presurgery and postsurgery were tested using paired t tests or Wilcoxon’s tests for normally or not normally distributed data, respectively. To study the inverse remodeling and collateral effects induced by the surgical procedure on MA and AoA parameters, unpaired Student’s t tests were applied between the control and MR-POST groups. For all comparisons, differences were considered significant at P < .05. Interobserver and intraobserver variability of the main parameters computed using the presented method have been published and were <10% for intraobserver measures; as expected, interobserver variability was slightly higher (8.0 ± 4.3%).
Results
The MV repair procedure was successfully performed in all patients. None were excluded before repair because of the presence of more than mild MR. All images obtained with the matrix-array TEE transducer were of high quality, resulting in 100% feasibility for both MA and AoA tracking throughout the cardiac cycle. Temporal resolution of the acquired data was relatively high and not significantly different among groups (control, 30 ± 12 Hz; MR-PRE, 29 ± 14 Hz; MR-POST, 34 ± 13 Hz). According to the MV morphology associated with the surgeons’ choices, 12 patients received St. Jude Tailor Flexible rings (mean size number, 32 ± 2; St. Jude Medical Inc., St. Paul, MN), eight patients received Carpentier-Edwards Physio annuloplasty rings (mean size number, 33 ± 3; Edwards Lifesciences, Irvine, CA), four patients received Cosgrove-Edwards rings (size number 28; Edwards Lifesciences), two received Simplici-T rings (size numbers 62 and 69; Medtronic, Inc., Minneapolis, MN), one received a Sorin Memo 3D ring (size number 32; Sorin Biomedica Cardio s.r.l., Saluggia, Italy), and one received a Carpentier-McCarthy-Adams IMR ETlogix ring (size 28; Edwards Lifesciences). Rings were secured predominantly using interrupted sutures, but because of different ring types in five subjects, a nonpledgeted mattress suture was used, and in two subjects, valvular U clips were used for the sutures.
Effects of Pathology on the MA and AoA
The presurgical cumulative results for the control group and MV patients are presented in Table 1 . As expected, the main alterations of the MV apparatus due to the presence of MR occurred in the MA, not affecting the AoA or MAC. The MA was dilated both at its minimum size during systole and at its maximum size during diastole ( Figure 3 ). Even if the change in MA dimension during the cardiac cycle was significantly larger in the MR-PRE subjects, MA pulsatility, represented by its percentage area change, was not different compared with controls. Interestingly, in MR-PRE patients, not only did the MA area enlarge but also the MA nonplanarity, as measured by the MA height, which was increased compared with control patients.
Variable | Control ( n = 25) | MR-PRE ( n = 28) | P (unpaired t test) |
---|---|---|---|
Maximal MA displacement (mm) | 8.5 ± 2.9 | 10.4 ± 2.4 | NS |
Minimal MA surface area (cm 2 ) | 7.8 ± 2.2 | 12.6 ± 3.3 | <.001 |
Maximal MA surface area (cm 2 ) | 9.9 ± 2.4 | 16.4 ± 4.0 | <.001 |
MA surface area change (%) | 21.2 ± 9.3 | 23.4 ± 7.9 | NS |
MA surface area change (cm 2 ) | 2.1 ± 1.0 | 3.8 ± 1.6 | <.01 |
Minimal MA height (mm) | 6.4 ± 1.5 | 7.6 ± 1.9 | NS |
Maximal MA height (mm) | 10.0 ± 2.0 | 13.5 ± 3.2 | <.01 |
MA height change (mm) | 3.5 ± 1.7 | 5.9 ± 2.8 | <.05 |
Minimal anteroposterior diameter (mm) | 25.3 ± 9.9 | 35.1 ± 5.0 | <.001 |
Maximal anteroposterior diameter (mm) | 30.1 ± 10.8 | 42.8 ± 5.0 | <.001 |
Minimal intercommissural diameter (mm) | 31.5 ± 5.0 | 41.0 ± 6.5 | <.001 |
Maximal intercommissural diameter (mm) | 35.8 ± 4.7 | 45.7 ± 7.0 | <.001 |
Projected area/3D surface area (%) | 93 ± 2 | 95 ± 3 | NS |

Effects of Surgical Intervention on MAC in MR Patients
The measured parameters for the MR-PRE and MR-POST populations are presented in Table 2 . Most parameters describing the MA and AoA underwent significant changes with MV repair. Interestingly, the expected changes in MA dimension and dynamics due to MV repair with the implantation of an annuloplasty ring were associated with alterations in AoA pulsatility (MR-PRE, 30 ± 14%; MR-POST, 22 ± 10%; see Figure 3 ). A global constriction of the mitral-aortic complex was noted, together with a reduction in the distance between the AV and MV centers, and a reduction of the intervalvular angle (MR-PRE, 126.3 ± 16.2°; MR-POST, 116.9 ± 10.7°; P < .002). As an effect of surgery, no significant regurgitant volume was present after the intervention; consequently, as expected, the computed total SV (MR-PRE, 118 ± 38 mL; MR-POST, 69 ± 17 mL) and ejection fraction (MR-PRE, 64 ± 9%; MR-POST, 53 ± 9%) were reduced in MR-POST patients. Measured AV area showed no changes after annuloplasty (MR-PRE, 3.6 ± 0.6 cm 2 ; MR-POST, 3.5 ± 0.7 cm 2 ).
Variable | MR-PRE ( n = 28) | MR-POST ( n = 28) | P (paired t test) |
---|---|---|---|
MA | |||
Maximal MA displacement (mm) | 10.4 ± 2.4 | 5.4 ± 1.9 | <.001 |
Minimal MA surface area (cm 2 ) | 12.6 ± 3.3 | 5.0 ± 1.4 | <.001 |
Maximal MA surface area (cm 2 ) | 16.4 ± 4.0 | 5.5 ± 1.5 | <.001 |
MA surface area change (%) | 23.4 ± 7.9 | 8.9 ± 5.8 | <.001 |
MA surface area change (cm 2 ) | 3.8 ± 1.6 | 0.5 ± 0.4 | <.001 |
Minimal MA height (mm) | 7.6 ± 1.9 | 4.0 ± 2.3 | <.001 |
Maximal MA height (mm) | 13.5 ± 3.2 | 6.8 ± 2.7 | <.001 |
MA height change (mm) | 5.9 ± 2.8 | 2.8 ± 0.9 | <.001 |
Minimal anteroposterior diameter (mm) | 35.1 ± 5.0 | 22.4 ± 3.2 | <.001 |
Maximal anteroposterior diameter (mm) | 42.8 ± 5.0 | 24.6 ± 3.7 | <.001 |
Minimal intercommissural diameter (mm) | 41.0 ± 6.5 | 25.9 ± 4.5 | <.001 |
Maximal intercommissural diameter (mm) | 45.7 ± 7.0 | 27.9 ± 4.7 | <.001 |
Projected area/3D surface area (%) | 95 ± 3 | 96 ± 3 | <.05 |
AoA | |||
Minimal AoA projected area (cm 2 ) | 4.2 ± 0.9 | 4.4 ± 1.2 | NS |
Maximal AoA projected area (cm 2 ) | 5.4 ± 1.1 | 5.4 ± 1.2 | NS |
AoA projected area change (cm 2 ) | 1.2 ± 0.5 | 0.9 ± 0.4 | <.01 |
AoA projected area change (%) | 30 ± 14 | 22 ± 10 | <.02 |
Axial AoA displacement (mm) | 6.0 ± 2.5 | 3.9 ± 1.7 | <.001 |
Longitudinal AoA displacement (mm) | 7.7 ± 2.3 | 3.9 ± 1.6 | <.001 |
MAC | |||
Distance between AoA center and mitral-aortic junction (mm) | 12.8 ± 1.3 | 15.2 ± 4.4 | <.01 |
Distance between MA center and mitral-aortic junction (mm) | 18.6 ± 2.9 | 12.1 ± 2.1 | <.001 |
Distance between AoA center and MA center (mm) | 27.9 ± 2.9 | 23.0 ± 2.0 | <.001 |
Angle between MV and AV (°) | 126.3 ± 16.2 | 116.9 ± 10.7 | <.002 |
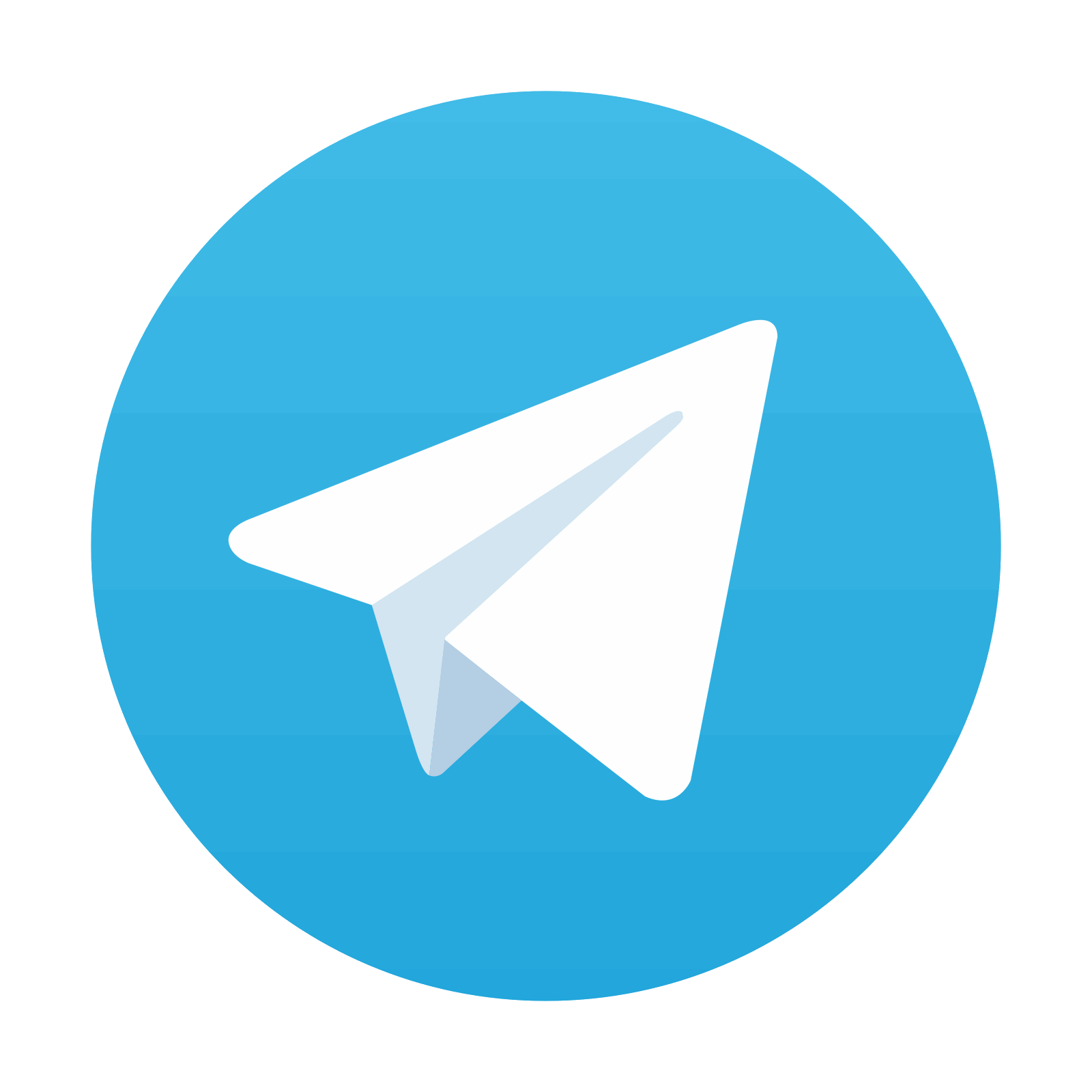
Stay updated, free articles. Join our Telegram channel

Full access? Get Clinical Tree
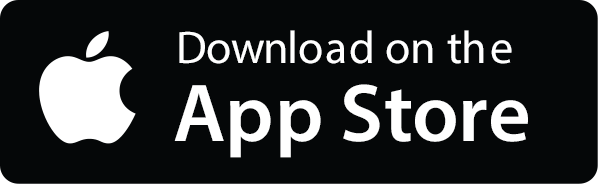
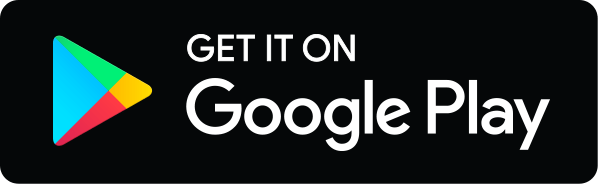
