The aim of this study was to determine the effect of intensified hypoglycemic therapy in patients with type 2 diabetes mellitus on the distribution of lipoprotein-associated phospholipase A2 (Lp-PLA2) activity between high-density lipoprotein and low-density lipoprotein (LDL) and its relation with the lipid profile and other qualitative properties of LDL. Forty-two patients with type 2 diabetes on the basis of poor glycemic control and normal or near normal LDL cholesterol were recruited. Lifestyle counseling and pharmacologic hypoglycemic therapy were intensified to improve glycemic control, but lipid-lowering therapy was unchanged. At 4 ± 2 months, glycosylated hemoglobin had decreased by a mean of 2.1%, but the only effect on the lipid profile were statistically significant decreases in nonesterified fatty acids and apolipoprotein B concentration. LDL size increased and the proportion of electronegative LDL decreased significantly. In parallel, total Lp-PLA2 activity decreased significantly, promoting a redistribution of Lp-PLA2 activity toward a higher proportion in high-density lipoprotein. Improvements in glycemic control led to more marked changes in Lp-PLA2 activity and distribution in patients with diabetes who had not received previous lipid-lowering therapy. In conclusion, optimizing glycemic control in patients with type 2 diabetes promotes atheroprotective changes, including larger LDL size, decreased electronegative LDL, and a higher proportion of Lp-PLA2 activity in high-density lipoprotein.
The mechanism by which patients with type 2 diabetes mellitus have an increased risk for cardiovascular disease is not fully understood. It may be due partly to the lipoprotein modifications and chronic low-grade inflammation associated with diabetes. These patients present atherogenic dyslipidemia, which is characterized by hypertriglyceridemia, low high-density lipoprotein (HDL) cholesterol, and hyper–apolipoprotein B (apoB). In addition, low-density lipoprotein (LDL) particles are small and dense, and indexes of LDL modification, such as lipoperoxidation and negative electric charge, are higher than in normoglycemic subjects. Lipoprotein-associated phospholipase A2 (Lp-PLA2) has been associated with increased cardiovascular risk in general and diabetic populations. Lp-PLA2 is transported in blood by lipoproteins, and it has been suggested that it may play an atherogenic role when bound to LDL but an atheroprotective role when bound to HDL. An abnormal distribution of Lp-PLA2, with a relatively lower content in HDL, has been reported in combined hyperlipidemia and diabetes. This could be related to the preferential association of Lp-PLA2 in small, dense LDL and electronegative LDL particles. Statins and nonstatin lipid-lowering drugs reduce the Lp-PLA2 plasma concentration in parallel with a reduction in LDL cholesterol level. Glycemic control improves the quantitative features of atherogenic dyslipidemia. No study has examined the specific effect of glycemic optimization on the qualitative abnormalities of LDL or changes in Lp-PLA2 distribution in subjects with type 2 diabetes. In the present study, we aimed to assess the effect of glycemic control improvement on Lp-PLA2 concentration and distribution and its relation with LDL size and indexes of LDL modification.
Methods
The study was performed in accordance with the Declaration of Helsinki. The study included 42 patients with type 2 diabetes recruited consecutively from the diabetes clinic on the basis of poor glycemic control, defined as glycosylated hemoglobin >8.5%, and recommended or near-to-recommended LDL cholesterol, defined as LDL cholesterol <3.4 mmol/L (<130 mg/dl) according to National Cholesterol Education Program Adult Treatment Panel III recommendations. Patients’ anthropometric and clinical characteristics are listed in Table 1 . Previous treatment consisted of oral hypoglycemic agents (5 patients), oral hypoglycemic agents plus basal insulin (7 patients) ≥2 doses of insulin with or without metformin (26 patients), and no previous treatment (4 patients). Regarding hypolipidemic drugs, 21 patients were treated with statins and 2 with statin plus ezetimibe. All patients were treated for their diabetes in a clinical practice setting. Treatment included lifestyle counseling and intensification of pharmacologic hypoglycemic therapy with oral agents (metformin or metformin plus sulfonylurea) in 6 patients, insulin therapy in 31 patients, and both (insulin plus metformin or insulin plus metformin and sulfonylurea) in 5 patients. Lipid-lowering therapy was unchanged. All participants attended a specific diabetes education program and were visited in the outpatient unit every 2 to 4 weeks. Assessment was carried out at baseline and after 3 to 6 months of treatment intensification. The study was approved by the hospital’s ethics committee, and all patients gave informed consent. All analyses were performed using fasting plasma obtained in Vacutainer tubes (BD, Franklin Lakes, New Jersey) containing ethylenediaminetetraacetic acid. The basic lipid profile was obtained using fresh samples. All other analyses were performed using ethylenediaminetetraacetic acid–plasma samples frozen at −80°C for <4 months. To minimize interassay variability, samples from the same subject were analyzed in the same batch. The variability of these methods has been reported elsewhere.
Variable | Baseline | After Glycemic Optimization |
---|---|---|
Women/men | 15/27 | — |
Age (years) | 61 ± 12 | — |
Diabetes duration (years) | 37 ± 14 | — |
Retinopathy | 59.0% | — |
Coronary heart disease | 17.9% | — |
Insulin use | 75.0% | 85.7% |
Cholesterol-lowering medication | 59.5% | 59.5% |
Body mass index (kg/m 2 ) | 29.0 ± 5.8 | 28.8 ± 6.0 |
Glycosylated hemoglobin (%) | 9.6 ± 1.8 | 7.4 ± 0.8 ⁎ |
Lipid profiles included total cholesterol, triglyceride, very low density lipoprotein cholesterol, LDL cholesterol, HDL cholesterol, nonesterified fatty acids, apoB, and apolipoprotein A-I (all reagents were from Roche Diagnostics GmbH, Basel, Switzerland, except nonesterified fatty acids, from Wako Chemicals, Neuss, Germany). Cholesterol in lipoprotein fractions was measured using a direct method that quantifies HDL cholesterol (HDL-C Plus; Roche Diagnostics GmbH), according to the National Cholesterol Education Program recommendations. All determinations were carried out on a Hitachi 917 autoanalyzer (Roche Diagnostics GmbH).
LDL size was determined by nondenaturing polyacrylamide gradient (2% to 16%) gel electrophoresis, using as standard a mixture of sera with several LDL bands of known size, as previously described. LDL phenotype B was defined as a predominant LDL diameter <25.5 nm.
Oxidized LDL was quantified by commercial enzyme-linked immunosorbent assay (Oxidized LDL; Mercodia, Uppsala, Sweden) according to the manufacturer’s instructions. The percentage of electronegative LDL was determined from total LDL by anion-exchange chromatography in a MonoQ HR 5/5 column (GE Healthcare, Uppsala, Sweden) using a sodium chloride stepwise gradient, as previously described.
Lp-PLA2 activity was measured using 2-thio platelet-activating factor (Cayman Chemicals, Ann Arbor, Michigan) as a substrate, according to the manufacturer’s instructions. Results are expressed as micromoles per minute per milliliter. To determine the distribution between lipoprotein fractions, apoB-containing lipoproteins (LDL plus very low density lipoprotein) were precipitated from serum using dextran sulfate, as previously described. Briefly, 200 μl of serum was mixed with 50 μl of dextran sulfate reagent, incubated for 5 minutes at room temperature, and centrifuged for 10 minutes at 10,000g. Twenty microliters of supernatant (containing HDL but not LDL or very low density lipoprotein) was collected and assayed for Lp-PLA2 activity.
Statistical analysis was performed using SPSS version 15 (SPSS, Inc., Chicago, Illinois). Data are expressed as mean ± SD, and Kolmogorov-Smirnoff tests were used to assess the distributions of variables. Groups were compared using Student’s paired t tests for paired data (normal distribution) or Wilcoxon’s tests (non-normal distribution). Correlations between changes in each parameter were analyzed using Pearson’s R test. A p value <0.05 was considered statistically significant.
Results
Anthropometric and clinical characteristics of patients are listed in Table 1 . After therapy intensification, glycosylated hemoglobin decreased significantly by >1% in all patients ( Table 1 ). Lipid parameters of patients with type 2 diabetes before (baseline) and after 4 ± 2 months of hypoglycemic therapy intensification are listed in Table 2 . Although the only quantitative lipid parameters that changed significantly after intensified glycemic control were plasma concentrations of nonesterified fatty acids and apoB, the decrease in glycosylated hemoglobin was correlated positively with changes in plasma triglyceride (R = 0.388, p = 0.006) and negatively with changes in HDL cholesterol (R = −0.552, p <0.001).
Variable | Baseline | After Glycemic Optimization | p Value | Percentage Change |
---|---|---|---|---|
Nonesterified fatty acids (mmol/L) | 0.72 ± 0.33 | 0.59 ± 0.25 | 0.018 ⁎ | −18 |
Triglycerides (mmol/L [mg/dl]) | 1.88 ± 1.77 (166 ± 156) | 1.37 ± 0.74 (121 ± 65) | 0.059 | −27 |
Total cholesterol (mmol/L [mg/dl]) | 4.94 ± 1.23 (191 ± 48) | 4.72 ± 1.13 (183 ± 44) | 0.192 | −4.4 |
HDL cholesterol (mmol/L [mg/dl]) | 1.24 ± 0.39 (48 ± 15) | 1.27 ± 0.40 (49 ± 15) | 0.270 | 2.4 |
LDL cholesterol (mmol/L [mg/dl]) | 2.91 ± 0.95 (113 ± 37) | 2.81 ± 1.00 (109 ± 39) | 0.448 | −3.4 |
Apolipoprotein A-I (g/L) | 1.45 ± 0.33 | 1.48 ± 0.29 | 0.261 | 2.1 |
ApoB (g/L) | 0.94 ± 0.27 | 0.87 ± 0.26 | 0.036 ⁎ | −7.4 |
LDL size (nm) | 25.72 ± 0.61 | 25.96 ± 0.55 | 0.008 ⁎ | 0.1 |
Oxidized LDL (U/L) | 62.4 ± 18.8 | 60.1 ± 18.7 | 0.428 | 3.7 |
Electronegative LDL (%) | 7.6 ± 3.4 | 6.7 ± 2.4 | 0.026 ⁎ | −11 |
The statistically significant increment in LDL size (also reflected in a higher LDL cholesterol/apoB ratio, 3.07 vs 3.19) observed after glycemic optimization ( Table 2 ) was correlated negatively with the decrease in glycosylated hemoglobin (R = −0.304, p = 0.033) and plasma triglyceride (R = −0.388, p = 0.006) and positively with changes in HDL cholesterol (R = 0.552, p <0.001). Patients with LDL subfraction phenotype B (LDL particle diameter <25.5 nm) were studied separately. Five of 11 patients showing LDL phenotype B at baseline changed to phenotype A after the optimization of glycemic control. This change was accompanied by an increase in HDL cholesterol in these subjects (from 1.34 ± 1.15 to 1.42 ± 1.16 mmol/L, p <0.001), although no statistical significance was obtained in plasma triglycerides (from 1.89 ± 2.04 to 1.42 ± 0.34 mmol/L, p = 0.488). LDL size was not the only qualitative change in LDL promoted by glycemic optimization, as the electronegative LDL proportion also decreased significantly after glycemic control was improved. In contrast, the oxidized LDL concentration was not modified.
Figure 1 shows changes in total Lp-PLA2 activity and Lp-PLA2 distribution after the improved glycemic control. Total activity decreased significantly by 10%, mainly because of a decrease in Lp-PLA2 activity associated with apoB-containing lipoproteins ( Figure 1 ). As a consequence, the relative distribution of Lp-PLA2 increased in HDL and decreased in apoB-containing lipoproteins ( Figure 1 ). Changes in total Lp-PLA2 were correlated mildly with total cholesterol (R = 0.340, p = 0.030), LDL cholesterol (R = 0.329, p = 0.038), and apoB (R = 0.349, p = 0.025), but stronger correlations were obtained with HDL cholesterol (R = −0.458, p = 0.003), apolipoprotein A-I (R = −0.374, p = 0.016), and glycosylated hemoglobin (R = 0.494, p = 0.001). The same significant correlations were observed with the relative proportion of Lp-PLA2 in HDL (data not shown).
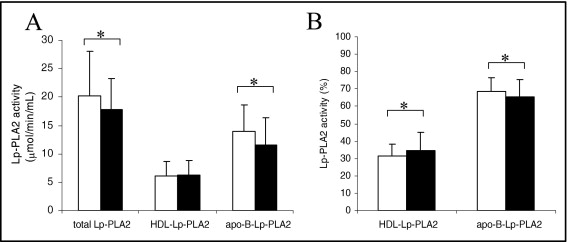
Although patients who had received lipid-lowering drugs had a more favorable overall lipid profile than those who had not, there was no statistical difference in any specific parameter of the lipid profile or in the qualitative characteristics of LDL between the 2 groups at baseline ( Table 3 ). Relative changes in the lipid profile or in the qualitative characteristics of LDL after intensified hypoglycemic therapy were also similar in the 2 groups. Regarding Lp-PLA2, patients who were receiving lipid-lowering therapy at baseline had lower Lp-PLA2 activity and higher relative content in HDL than those who were not receiving this therapy ( Figure 2 ). Intensified hypoglycemic treatment decreased significantly Lp-PLA2 activity and increased significantly the proportion of Lp-PLA2 in HDL in patients who did not receive previous lipid-lowering therapy but not in those receiving lipid-lowering therapy.
