External mechanical forces can cause ventricular capture and fibrillation (i.e., commotio cordis). In animals, we showed that chest compressions (CCs) can also cause the phenomenon. The aim of the present study was to determine whether ventricular capture by CCs occurs in humans. Electronic rhythm strips were analyzed in 31 cases of out-of-hospital cardiac arrest. The timing of the CCs was identified from the changes in thoracic impedance between the defibrillator pads. Ventricular capture was defined as QRS complexes of similar morphology occurring intermittently but synchronized with the CC artifact and impedance waveform. Only intermittent ventricular capture was identified to avoid misclassifying constant motion artifacts or intrinsic rhythm as ventricular capture. Of the 29 patients who received CCs for ≥1 minute, minimal or stable motion artifact was present in 24. Intermittent ventricular capture was found in 7 of the 24 patients. In the patients with ventricular capture, the number of ventricular activations (from ventricular capture and native beats) was greater during the CCs than when the CCs was not being performed (18 ± 8.9 vs 9.7 ± 4.0 activations in 15 seconds, p = 0.01). However, in patients without ventricular capture, they were similar (6.8 ± 8.2 vs 7.2 ± 8.8 activations in 15 seconds, p = 0.47). Refibrillation occurred in 22 patients; it began during the CCs in 16 and closely following their initiation in 3. In conclusion, CCs during cardiopulmonary resuscitation can electrically stimulate the heart. Additional studies evaluating the effect of ventricular capture on cardiopulmonary resuscitation outcomes, its relation to refibrillation, and methods to prevent or time ventricular capture by CCs are warranted.
Externally applied mechanical forces, such as precordial thumps or chest wall impacts with baseballs (i.e., commotio cordis) can cause ventricular capture and, in certain cases, ventricular fibrillation (VF). This phenomenon has been extensively studied and is thought to be modulated by the stretch-activated ion channels. We have recently reported that the mechanical forces generated by chest compressions (CCs) can also cause ventricular capture in both swine and canines. Such ventricular capture during CCs occurred after defibrillation of VF of long duration, which, under certain conditions, created long–short cycles of activation that led to refibrillation. Although mechanistically plausible, ventricular capture has not been documented during cardiopulmonary resuscitation (CPR) in humans. Therefore, the primary objective of the present study was to search for evidence of ventricular capture by CCs in cases of out-of-hospital sudden cardiac arrest. The secondary objective was to determine whether refibrillation could be linked to the ventricular capture caused by CCs.
Methods
Patients who sustained out-of-hospital cardiac arrest in the Greater Birmingham, Alabama area from January to August 2008 were studied. The subjects had been enrolled in the Resuscitation Outcomes Consortium-Cardiac Arrest Population-based Registry (ROC-Epistry). The Resuscitation Outcomes Consortium is a clinical research network consisting of 10 clinical centers in the United States and Canada organized to conduct randomized trials pertaining to trauma and out-of-hospital sudden cardiac arrest. The ROC-Epistry Registry was designed to assess how evidence-based resuscitation is implemented in community-based practice. The present observational study, based on existing data sources, met the requirements for minimal risk and waiver of informed consent. Local institutional review board approval was obtained before having access to de-identified data files. Outcomes data were not obtained.
All patients studied were resuscitated in the field using LifePak 12 and 500 defibrillators (Physio-Control, Redmond, Washington). These defibrillators can continuously record electrocardiographic and thoracic impedance waveforms. The rhythm strips recorded electronically were obtained from the defibrillation patches used. Proprietary software (CODE-STAT, version 7.0, Physio-Control) was used to analyze the electronic files, retrieved from the defibrillators. The software allows for analysis of both rhythm strips and thoracic impedance waveforms.
Large or chaotic artifacts produced by CCs can render rhythm strips obtained during CPR uninterpretable. Frequently, however, a stable, oscillating artifact is seen ( Figure 1 ). In these cases, we searched for evidence of ventricular capture caused by CCs. Periods of pulseless electrical activity or asystole, either as the initial rhythm or after successful defibrillation, were used for analysis.
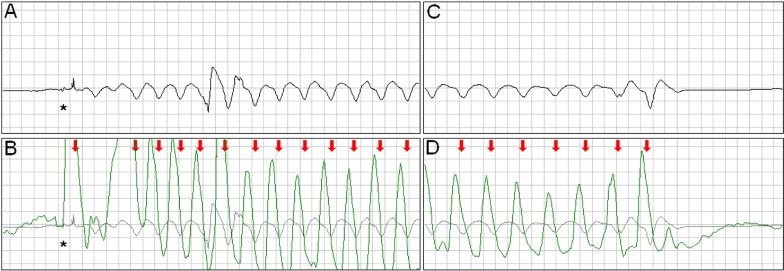
The external defibrillators measured and recorded impedance continuously by passing a safe, high-frequency (14 to 20 kHz), alternating current between the defibrillation pads and measuring the voltage generated. A computer algorithm identified CC events ( Figure 1 ) using a method that has been clinically validated. The software marks each CC event with a red arrow ( Figures 1 to 5 ) .
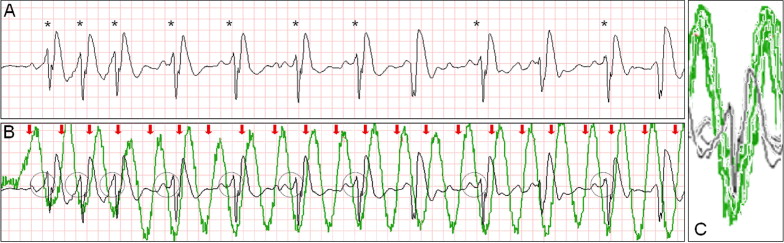
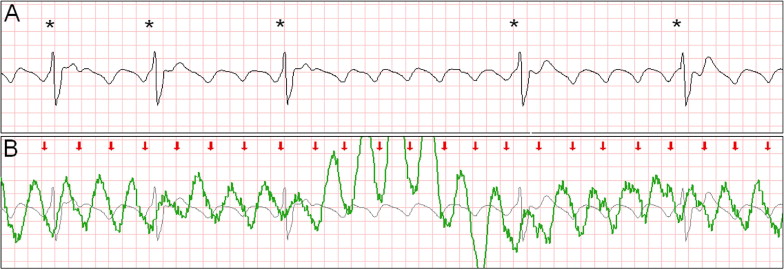
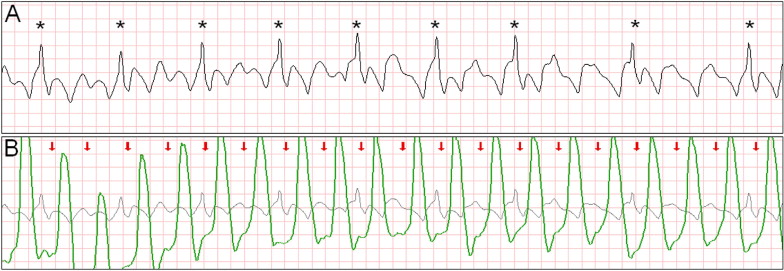
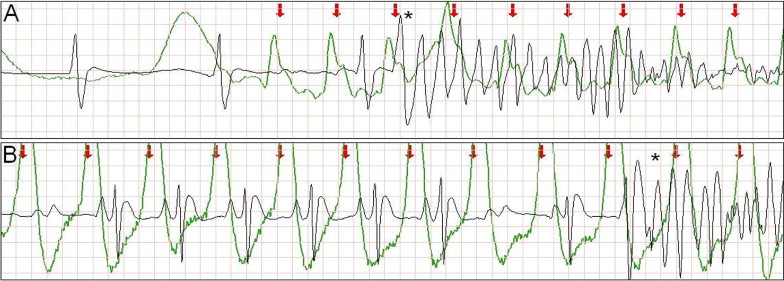
Intermittent ventricular capture was defined as the presence of QRS complexes of similar morphology, that were intermittently associated with the CC cycles determined from thoracic impedance, and that exhibited a constant time relation with the CC artifact seen on the rhythm strip and with the impedance signal. Only cases of intermittent ventricular capture were considered, because of the difficulty in differentiating constant ventricular capture in a 1:1 ratio with the CC from a constant motion artifact or an intrinsic rhythm of coinciding rate. Two groups were defined according to the presence or absence of ventricular capture by CCs. Ventricular activations were defined as QRS complexes that were either intrinsic beats or considered from ventricular capture. Successful defibrillation was defined as the absence of VF 5 seconds after defibrillation.
In the 24 cases in which CCs produced only minor or stable motion artifacts, the number of ventricular activations during a 15-second period while CCs were being performed was compared to a 15-second period in which CCs were not (immediately before the initiation of CCs when possible). In the group of patients in whom ventricular capture was found, the first segment in which it was seen was used. In 2 cases, however, CCs were started within a few seconds of successful defibrillation. In these cases, the 15 seconds before and after the cessation of CCs were used. In the group of patients in whom ventricular capture was not found, the first segment in which CCs produced motion artifact and the 15 seconds preceding it were used.
The number of episodes of refibrillation (in cases in which the initial rhythm was VF) was analyzed. In the cases in which the initial rhythm was not VF, episodes of de novo VF were quantified, and for analysis purposes were categorized as refibrillation. A standard electrocardiographic gain and sweep speed (10 mm/mV and 25 mm/s, respectively) was used for all the figures shown, unless noted otherwise.
The Statistical Package for Social Sciences was used for statistical analysis. The Wilk-Shapiro test was used to assess for normality of the distribution of the data. The number of ventricular activations during a 15-second period was normally distributed. Continuous variables are expressed as the mean ± SD. The paired t test with equal variances not assumed was used to compare the number of ventricular activations before and during CCs in each group (with and without ventricular capture). An independent samples Student’s t test was used to compare the change in the number of ventricular activations before and during CCs in both groups. Statistical significance was defined as a 2-tailed p value <0.05.
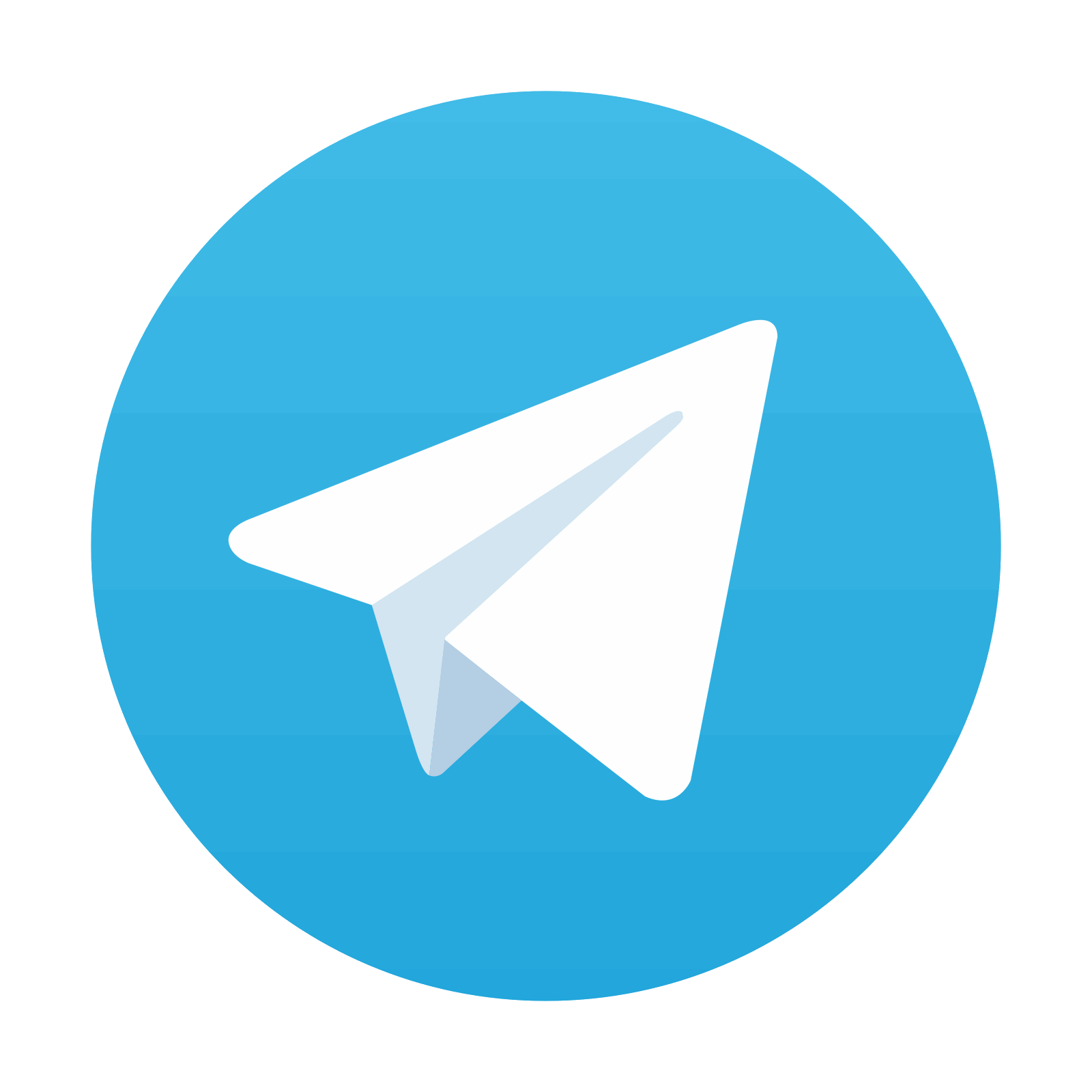
Stay updated, free articles. Join our Telegram channel

Full access? Get Clinical Tree
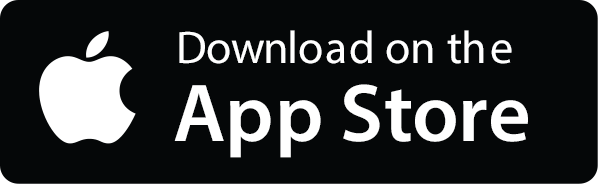
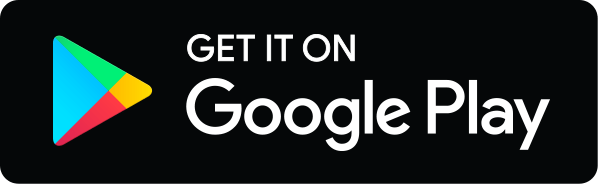