Right ventricular (RV) volume overload secondary to pulmonary regurgitation is common in patients after initial repair of tetralogy of Fallot (TOF) and is associated with adverse long-term outcomes. The objective of the present study was to determine the effect of branch pulmonary artery stenosis on the RV volume in patients with repaired TOF. We reviewed 178 cardiac magnetic resonance imaging studies in patients with repaired TOF. We defined bilateral stenosis as a Nakata index of ≤200 mm 2 /m 2 and concordant branch pulmonary artery cross-sectional area, unilateral stenosis as 1 branch pulmonary artery cross-sectional area ≤100 mm 2 /m 2 and 1 branch pulmonary artery cross-sectional area >100 mm 2 /m 2 , and restrictive physiology as prograde main pulmonary artery diastolic flow. Of the 178 patients, 20 (11%) had bilateral stenosis, 47 (26%) unilateral stenosis, and 111 (63%) had no stenosis. The RV end-diastolic volume was lower in patients with bilateral (125 ± 27 ml/m 2 ) or unilateral (131 ± 43 ml/m 2 ) stenosis than in those without stenosis (149 ± 35 ml/m 2 , p = 0.021 and p = 0.019, respectively). The main pulmonary artery regurgitant fraction was greater in patients without stenosis (47%, range 2% to 69%) than in those with bilateral (33%, range 9% to 59%; p = 0.009) or unilateral stenosis (40%, range 0% to 71%; p = 0.033). Restrictive physiology was more common in patients with bilateral (13 of 15, 87%) or unilateral (21 of 38, 55%) stenosis than in those without stenosis (28 of 85, 33%; p <0.001 and p = 0.017, respectively). In conclusion, in patients with repaired TOF, bilateral and unilateral branch pulmonary artery stenosis was associated with a greater main pulmonary artery regurgitant fraction and smaller RV end-diastolic volume than those in patients without stenosis, likely owing to the development of restrictive physiology. Branch pulmonary artery stenosis might effectively delay the referral for pulmonary valve replacement.
Significant pulmonary regurgitation (PR) is common after initial repair of tetralogy of Fallot (TOF) and results in right ventricular (RV) dilation, as assessed by cardiac magnetic resonance imaging (CMR). Initially thought to be relatively benign in patients after TOF repair, PR has been subsequently associated with worsened exercise tolerance, ventricular tachyarrhythmia, and sudden death. One long-term outcomes study found that an RV end-diastolic volume z-score of ≥7 was associated with death, sustained ventricular tachycardia, or functional deterioration. The link between branch pulmonary artery stenosis and RV volume overload in patients with repaired TOF has not been clearly delineated. Unilateral branch pulmonary artery stenosis might exacerbate PR and increase the RV systolic pressure, and stenting of unilateral branch pulmonary artery stenosis might decrease PR. We hypothesized that branch pulmonary artery stenosis would be associated with a greater main pulmonary artery regurgitant fraction and larger RV end-diastolic volume.
Methods
We performed a retrospective review of the Texas Children’s Hospital radiology database for patients with TOF who had undergone CMR after initial TOF repair, before any subsequent intervention on the pulmonary valve or branch pulmonary arteries, as part of their clinical care from April 1997 through May 2011. We included patients with TOF and pulmonary atresia who had received a right ventricle-to-pulmonary artery conduit as part of their initial repair and those with TOF and atrioventricular septal defect. We excluded patients with absent pulmonary valve syndrome and those with aortopulmonary collaterals as the major source of pulmonary blood flow. In patients with serial examinations, we gathered data from the most recent CMR before an intervention on the pulmonary valve or a pulmonary artery. We excluded patients for whom the image quality was not sufficient for pulmonary artery measurement.
Clinical data were collected for all cases using the medical record, including demographic information and the timing of interventions. CMR anatomic measurements and flow data were collected, as detailed. We collected hemodynamic data for the patients who had undergone cardiac catheterization within 1 year of the CMR. We only included catheterization data for patients who had not undergone a catheter-based or surgical intervention between catheterization and the CMR study.
All CMR studies were performed using a 1.5-T system (Achieva/Intera, Philips Medical Systems, Best, The Netherlands). All pulmonary artery measurements were performed by a single observer (S.A.M.) with expertise in cardiac imaging, using gadolinium-enhanced magnetic resonance angiography, in the orthogonal planes and using an AquariusNET system (TeraRecon, Foster City, California). The measurements were at the following locations: proximal branch pulmonary artery (immediately distal to the main pulmonary artery), narrowest branch pulmonary artery, and distal branch pulmonary artery (immediately proximal to the first segmental branch). Volumetric analyses were performed on a Viewforum workstation (Phillips Medical Systems, Utrecht, The Netherlands) using balanced steady-state free precession acquisitions in the short-axis plane. The endocardium was traced, excluding the papillary muscles, on each of 12 to 14 end-diastolic and end-systolic frames spanning the ventricles. These measurements were used to calculate the RV and left ventricular end-diastolic volumes, end-systolic volumes, and ejection fractions. The end-diastolic volumes were indexed to the body surface area, calculated using the Haycock formula. The phase contrast flow data in the main pulmonary artery, right pulmonary artery, and left pulmonary artery were collected.
The phase contrast flow data were used to calculate the gross forward flow, regurgitant flow, and net flow across the main pulmonary artery or right ventricle-to-pulmonary artery conduit and each branch pulmonary artery. All phase contrast imaging was performed with free breathing. The differential net pulmonary blood flow, differential gross pulmonary blood flow, and regurgitant fraction of each branch pulmonary artery was then calculated. We evaluated the flow versus time plots derived from the phase contrast data for the presence of restrictive physiology, defined by prograde diastolic flow in the main pulmonary artery, and diastolic impairment, defined by an abnormal RV filling pattern (reversal of the tricuspid E and A waves).
We grouped the patients into those with bilateral stenosis, unilateral stenosis, or no stenosis. The group assignment was determined by the Nakata index and branch pulmonary artery cross-sectional area (CSA). The Nakata index was calculated using the smallest calculated branch pulmonary artery CSA on each side: right pulmonary artery CSA (mm 2 ) + left pulmonary artery CSA (mm 2 )/body surface area (m 2 ). We also calculated the differential fractional CSA by dividing the absolute value of the difference between the right pulmonary artery CSA and the left pulmonary artery CSA by the sum of the right pulmonary artery CSA and the left pulmonary artery CSA: differential fractional CSA = |(right pulmonary artery CSA − left pulmonary artery CSA)|/right pulmonary artery CSA + left pulmonary artery CSA. We defined bilateral stenosis as a Nakata index of ≤200 mm 2 /m 2 and the absence of significant size discrepancy between the right and left pulmonary arteries. We defined branch pulmonary artery size discrepancy as differential fractional CSA >0.33 m 2 , similar to another study of the branch pulmonary artery size in patients with TOF. We defined unilateral stenosis as 1 branch pulmonary artery CSA of ≤100 mm 2 /m 2 and 1 branch pulmonary artery CSA >100 mm 2 /m 2 . The patients who did not fit either criterion were designated as having no stenosis. The differential pulmonary blood flow and differential fractional CSA, although commonly used to assess branch pulmonary artery stenosis, are subject to error. If 1 branch pulmonary artery is dilated and the other has a normal caliber, they could demonstrate a significant discrepancy in the absence of stenosis. Therefore, the categorical assessment of branch pulmonary artery stenosis as outlined was our primary assessment.
Continuous data with a normal distribution are reported as the mean ± SD, and data with a non-normal distribution are reported as the median and range. A comparison of the mean values was performed using the paired t test, independent t test, and analysis of variance, as applicable. Nonparametric comparisons were performed using the Mann-Whitney U and Kruskal-Wallis tests. A correlation of the continuous data sets was performed using Pearson’s r for normally distributed data and Spearman’s rho for data that were not normally distributed. All statistical analysis was performed using SPSS software, version 19.0 (IBM, Armonk, New York). The Baylor College of Medicine institutional review board approved the present study.
Results
A total of 226 patients who had undergone initial TOF repair and had not yet undergone subsequent pulmonary valve intervention underwent CMR studies at our institution from April 1997 through May 2011. Of these patients, 48 were excluded because of insufficient image quality or availability, including 9, for whom coil artifact obscured the branch pulmonary artery anatomy to an unacceptable degree. The patient characteristics, grouped by the presence and laterality of branch pulmonary artery stenosis, are listed in Table 1 .
All Patients (n = 178) | Bilateral Stenosis (n = 20) | Unilateral Stenosis (n = 47) | No Stenosis (n = 111) | |
---|---|---|---|---|
Male gender | 100 (58%) | 14 (70%) | 23 (49%) | 63 (57%) |
Pulmonary atresia | 7 (4%) | 0 | 3 (13%) | 4 (6.3%) |
Genetic syndrome | 9 (5%) | 3 (15%) ∗ | 4 (9%) | 2 (1.8%) |
Age at initial repair | 1.0 (1 day to 9.9 yrs) | 1.0 (3 months to 5.0 yrs) | 0.7 (1 day to 6.3 yrs) † | 1.07 (1 day to 8.5 yrs) |
Age at cardiac magnetic resonance imaging (yrs) | 15.0 (0.4–55.3) | 14.5 (4.3–28.4) | 6.5 (0.4–23.2) ‡ | 15.9 (5.4–55.3) |
Era of initial repair § | ||||
1960–1980 | 21 (9.3%) | 0 | 0 | 16 (14%) |
1981–1990 | 35 (15.5%) | 4 (20%) | 2 (4%) | 18 (16%) |
1991–2000 | 127 (56.2%) | 12 (60%) | 18 (38%) | 70 (63%) |
2001–2010 | 43 (19.0%) | 4 (20%) | 27 (57%) | 7 (6%) |
∗ p = 0.028, compared to no stenosis group.
† p = 0.003, compared to no stenosis group.
‡ p <0.001, compared to both no stenosis group and bilateral stenosis group.
The mean Nakata index was 325 ± 143 mm/m 2 , the median differential pulmonary blood flow and differential fractional CSA was 10% (range 0% to 52%) and 0.19 (range 0.00 to 0.81), respectively. The mean main pulmonary artery regurgitant fraction was 41 ± 16%, and the mean RV end-diastolic volume was 143 ± 40 ml/m 2 . Flow versus time diagrams were available at the main pulmonary artery level for 178 patients and at the tricuspid valve for 153 patients. Restrictive physiology was present in 77 (43%), and E/A reversal was present in 89 (58%). Cardiac catheterization with full hemodynamic data were performed within 1 year of CMR in 51 patients (29%); 38 (21%) of whom had sufficient image quality for branch pulmonary artery measurement. Branch pulmonary artery dilation was performed in 34 patients (19%), and 8 (4%) had pre- and postbranch pulmonary artery dilation CMR data available.
Bilateral stenosis was present in 20 of 178 patients (11%), unilateral stenosis in 47 (26%), and 111 (63%) had no stenosis. The RV end-diastolic volume was lower in the patients with bilateral stenosis (125 ± 27 ml/m 2 ) and those with unilateral stenosis (131 ± 43 ml/m 2 ) compared to those without stenosis (149 ± 35 ml/m 2 , p = 0.021 and p = 0.019, respectively; Figure 1 ). No difference was seen in the RV end-diastolic volume or main pulmonary artery regurgitant fraction between those with bilateral stenosis and those with unilateral stenosis (p = 0.794 and p = 0.327, respectively). Patients without stenosis had greater main pulmonary artery regurgitant fraction (47%, range 2% to 69%) than those with bilateral branch pulmonary artery stenosis (33%, range 9% to 59%; p = 0.009) and those with unilateral stenosis (40%, range 0% to 71%; p = 0.033; Figure 1 ). No difference was found between patients with bilateral stenosis and those with unilateral stenosis or without stenosis in terms of the RV ejection fraction, left ventricular ejection fraction, or left ventricular end-diastolic volume. No difference was found between the patients with unilateral stenosis and those without stenosis in terms of the RV ejection fraction or left ventricular ejection fraction, although patients with unilateral stenosis had a lower left ventricular end-diastolic volume than patients without stenosis (70 ± 14 ml/m 2 vs 77 ± 16 ml/m 2 , p = 0.025). A smaller Nakata index was associated with a decreased main pulmonary artery regurgitant fraction (R S = 0.20, p <0.001), smaller RV end-diastolic volume (R P = 0.22, p = 0.003), and smaller left ventricular end-diastolic volume (R P = 0.20, p = 0.010). A smaller Nakata did not correlate with the RV (R P = 0.05, p = 0.500) or left ventricular (R P = 0.01, p = 0.861) ejection fraction.
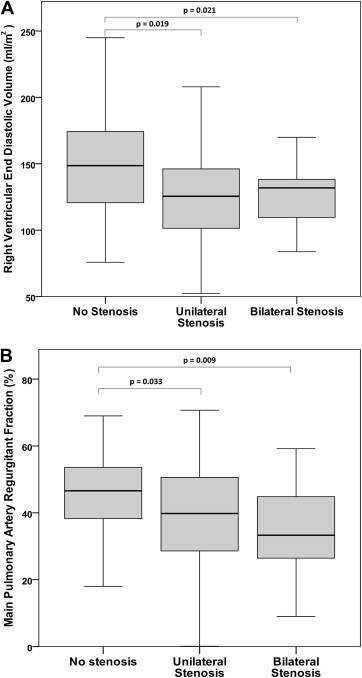
Patients with bilateral stenosis were more likely to have restrictive physiology (13 of 15, 87%) than those without stenosis (28 of 85, 33%; p <0.001) or those with unilateral stenosis (21 of 38, 55%; p = 0.030). Patients with unilateral stenosis had a greater rate of restrictive physiology than those without stenosis (p = 0.017). The hemodynamic data, grouped by the presence and laterality of branch pulmonary artery stenosis, are listed in Table 2 .
Variable | No Stenosis (n = 99) | Unilateral Stenosis (n = 47) | p Value | Bilateral Stenosis (n = 20) | p Value |
---|---|---|---|---|---|
Right ventricular end-diastolic volume (ml/m 2 ) | 149 ± 35 | 131 ± 43 | 0.019 | 125 ± 27 | 0.021 |
Pulmonary artery regurgitant fraction (%) | 47% (2–69%) | 40% (0–71%) | 0.033 | 33% (9–59%) | 0.009 |
Prograde pulmonary artery diastolic flow | 28/85 (33%) | 21/38 (55%) | 0.017 | 13/15 (87%) ∗ | <0.001 |
Right ventricular systolic pressure (mm Hg) (n = 51) | 34 ± 4 | 42 ± 11 | 0.238 | 46 ± 16 | 0.109 |
Right ventricular end-diastolic pressure (mm Hg) (n = 51) | 8 ± 3 | 8 ± 4 | 0.975 | 10 ± 4 | 0.590 |
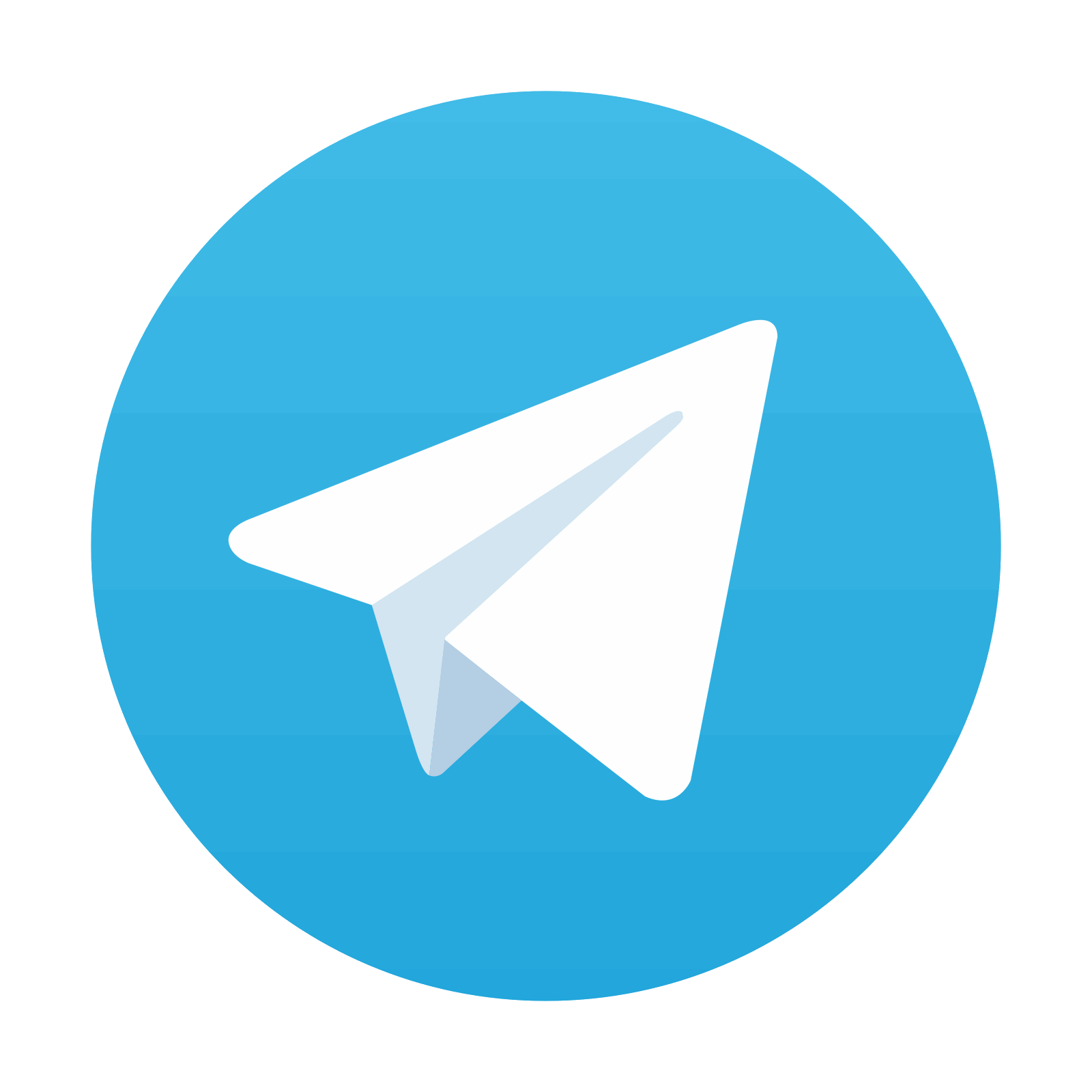
Stay updated, free articles. Join our Telegram channel

Full access? Get Clinical Tree
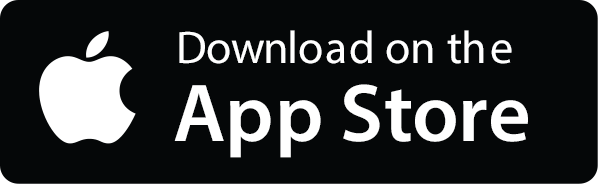
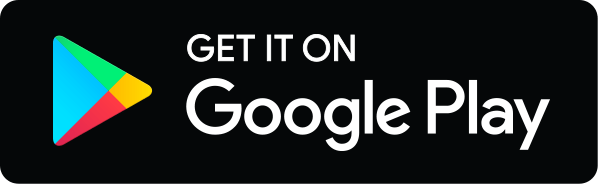
