(1)
Institute of Pathology, Medical University Graz, Graz, Austria
Lung edema is defined as an accumulation of fluid within alveoli and small bronchi/bronchioles. Edema fluid enters the peripheral lung from the circulation via the interstitium into alveoli. It can be induced by various causes. The most common form is due to congestion of the pulmonary circulation, most often caused by heart failure either due to infarction, valvular diseases, and the like. In these cases the venous flow into the left atrium is reduced, resistance in the venous part of the circulation increases, and leakage of the pulmonary veins increase. The gaps between the endothelial cells increase in size and serum gets into the interstitium and causes interstitial edema. In this case the composition of proteins and electrolytes are essentially similar to their concentration within the bloodstream. However, large proteins usually are lacking, because their large size prevents transudation in the early phases of edema development. In late phase of edema, this changes and also large proteins can be found within the fluid. Edema impairs respiration. Due to hypoxia patients will start with forced breathing. Air mixes with edema fluid resulting in foamy fluid, which can be easily recognized on patient inspection. Reduced oxygenation of the red blood cells and increased resistance in the peripheral circulation finally causes right ventricular failure and death.
4.1 Gross Morphology
Lungs are heavy, sometimes double the weight of normal, and the color is dark red. In cut surface foamy fluid is immediately starting to flow.
4.2 Histology
The alveoli are filled with fluid, most often acellular. A few macrophages might be seen, some of them containing hemosiderin. In more severe cases, also red blood cells will enter the alveoli. The capillaries are dilated and filled with blood. Within the vascular bed, there is no increase of leukocytes (Fig. 4.1).


Fig. 4.1
Lung edema due to cardial insufficiency; the capillaries are dilated, within the alveoli is an eosinophilic fluid and scattered red blood cells. There is no inflammatory cell infiltration. H&E, ×100
4.3 High-Altitude Edema (HAPE)
High-altitude edema is a condition, which morphologically resembles edema due to cardiac insufficiency; however the mechanisms are different. Mountaineering over 3,500 m of altitude can cause headache, vomiting, and finally edema of brain and lung. Mountaineers have to leave the altitude immediately to be rescued. Several drugs have been tested and several of them such as nifedipine will help in therapy as well as in prevention. Research in HAPE has highlighted two main factors responsible for pulmonary edema. One of them is stress failure of pulmonary capillaries resulting in a high-permeability form of edema or even frank hemorrhage [1]. Another factor is defective alveolar fluid clearance. This most likely is based on nonfunctioning endothelial and epithelial nitric oxide synthesis predisposing to hypoxic pulmonary vasoconstriction, capillary stress failure, and alveolar fluid flooding [2]. Asymptomatic alveolar fluid accumulation may be a normal phenomenon in healthy humans shortly after arrival at high altitude. Two fundamental mechanisms determine whether fluid accumulation is cleared or progresses to HAPE: the quantity of liquid escaping from the pulmonary vasculature and the rate of its clearance by the alveolar respiratory epithelium. The former is directly related to the degree of hypoxia-induced pulmonary hypertension, whereas the latter is determined by the alveolar epithelial sodium transport [3]. Several investigations have shaded light on mechanisms underlying these defects.
Potassium voltage channels (Kv1.2, Kv1.5, and Kv2.1) are sensitive to subacute hypoxia. Decreased expression has physiologic effects on membrane potential and cytosolic calcium. K+ channels may also be involved in the mechanism of high-altitude pulmonary edema and hypertension [4]. During normoxia, the redox mediator hydrogen peroxide maintains voltage-gated O2-sensitive K+ channels (Kv) in an oxidized open state. Hypoxic withdrawal of ROS inhibits Kv channels, activates voltage-gated Ca2+ channels, enhances Ca2+ influx, and promotes vasoconstriction. The unique occurrence of hypoxic vasoconstriction in the pulmonary circulation relates to the co-localization of an O2-sensor and O2-sensitive Kv channels in resistance pulmonary arteries [5]. Oxygen tension sensing mechanisms are involved in hypoxic adaptation such as hypoxia-inducible factor-1 (HIF1). Genes involved in adaptation to hypoxia are angiotensin-1-converting enzyme, tyrosine hydroxylase, serotonin transporter, and endothelial NO synthase genes [6].
When the epithelial barrier is compromised at high altitude, the normally high level of VEGF in the alveolar epithelial fluid has access to the pulmonary endothelium, due to openings of tight junctions, where it acutely alters permeability, markedly exacerbating high-altitude pulmonary edema [7]. In the experimental study by Kolluru, leakiness of the endothelial monolayer was increased by twofold under hypoxia compared to cells under normoxia. F-actin stress fibers were depolymerized under hypoxia. Nitric oxide, cyclic guanosine monophosphate (cGMP), and a phosphodiesterase type 5 inhibitor led to recovery from hypoxia-induced leakiness of the endothelial monolayers [8]. Insufficient NO synthesis is also related to augmented oxidative stress and may represent an underlying mechanism predisposing to pulmonary hypertension [9]. Finally the study by Comellas pointed to increased endothelin-1 (ET-1) and decreased alveolar fluid reabsorption in patients with high-altitude pulmonary edema. If the endothelin receptor ETB is blocked, alveolar fluid reabsorption can be reestablished. Exposing endothelial cell cultures to ET-1 resulted in increased NO. ET-1, via an endothelial-epithelial interaction, leads to decreased alveolar fluid reabsorption by activation of endothelial ETB receptors and NO generation [10].
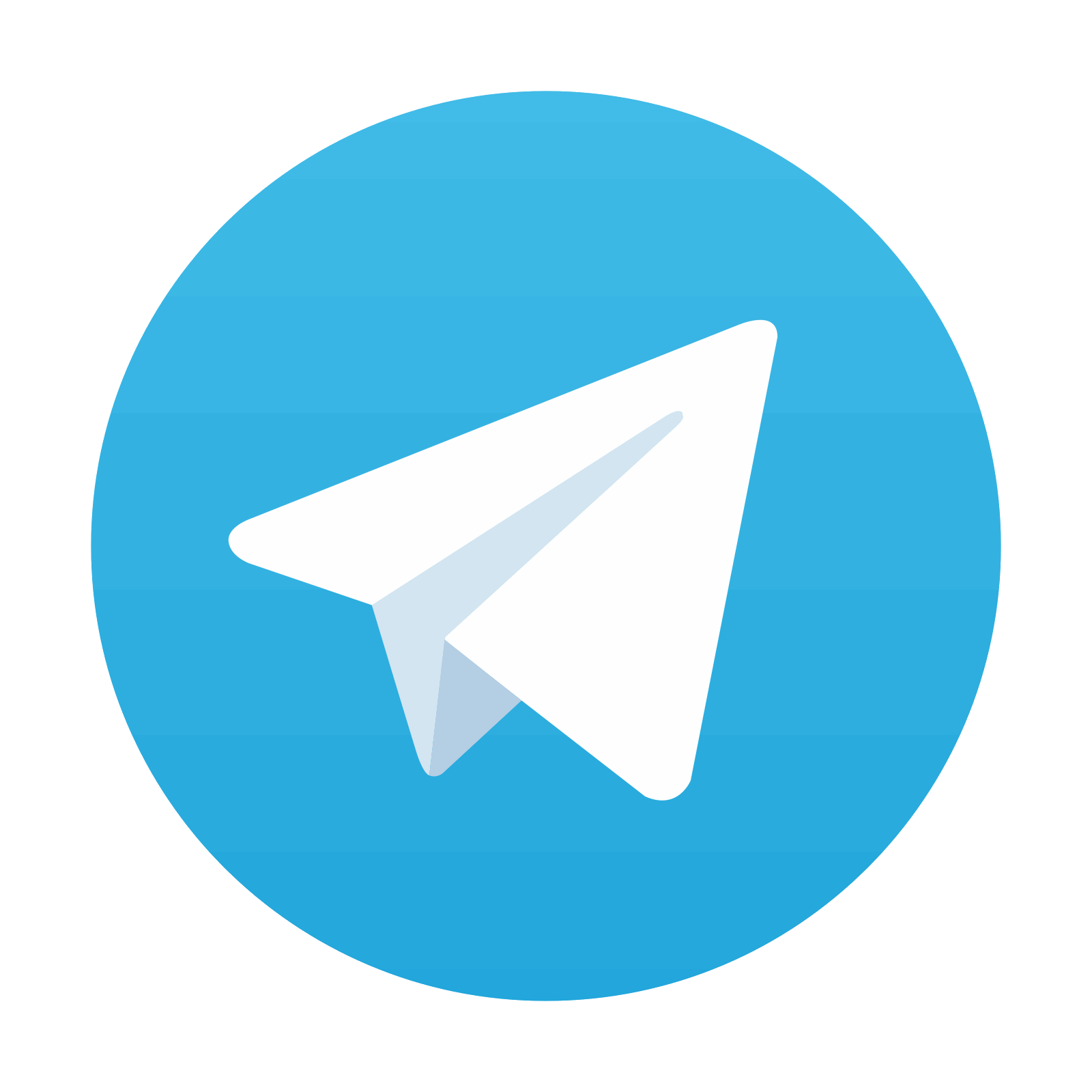
Stay updated, free articles. Join our Telegram channel

Full access? Get Clinical Tree
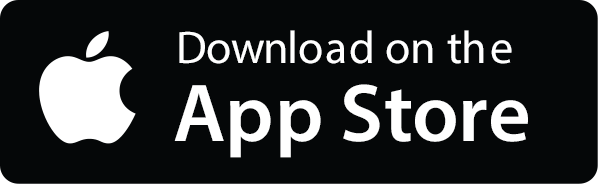
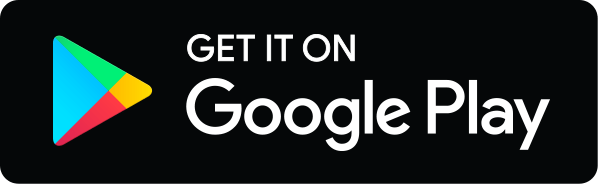