Chapter 14 Echocardiography in Respiratory Medicine
Echocardiographic Planes and Right-Sided Heart Structures
The right ventricle (RV) is located anterior to the left ventricle (LV) and is positioned just behind the sternum. When viewed anteriorly, the right ventricular cavity appears triangular in shape; however, it takes a crescentic shape in cross section. This anatomic orientation means that no single acoustic window allows visualization of the whole of the RV, so multiple transducer positions must be used. Standard echocardiography of the right side of the heart includes use of the parasternal (long-axis, right ventricular inflow, short-axis) (see Videos 1 and 2), apical (four-chamber) (see Video 3 ) and (modified four-chamber), and subcostal acoustic windows (see Video 4), generating a report that includes a qualitative description of right-sided heart anatomy and function, with at least one measurement of right atrial and right ventricular size, and a quantitative assessment of right ventricular function (Table 14-1). The venous inflow to the right side of the heart comprises the coronary sinus, superior vena cava, and inferior vena cava. The venous inflow is easily visualized from the parasternal acoustic window, in the right ventricular inflow view (Figure 14-1, A, and Video 5) and from the parasternal acoustic window, in the right ventricular inflow view (see Figure 14-1, B, and Video 4). The right atrium, tricuspid valve, and RV (inlet, free wall, apex, and infundibulum) are seen from the parasternal short axis (see Figure 14-1, C) and modified apical four-chamber views (see Figure 14-1, D). The tricuspid valve comprises septal, anterosuperior, and posterior leaflets, attached by chordae tendineae to papillary muscles located within the septum and lateral walls of the RV. Unlike with the aortic and mitral valves, it is not possible to see all three leaflets of the tricuspid valve from any single two-dimensional view—the septal and anterosuperior leaflets are seen in the apical four-chamber view, with the posterior and anterosuperior leaflets seen in the right ventricular inflow view. The pulmonary valve consists of three cusps without subvalvular apparatus and, along with the main pulmonary artery and proximal branch pulmonary arteries, is best seen on the parasternal short axis (see Figure 14-1, C, and Video 6) and rotated subcostal views. Normal ranges for right ventricular and right atrial size have been derived from normal healthy populations. An important point in this context is that these measures are not indexed to body surface area, so reported values may lose discriminatory power in assessment of these structures at either end of the normal distribution curve for size.
Table 14-1 Normal Limits for Recommended Measurements of Right-Sided Heart Structure and Function
Variable | Abnormal | Illustrated in: |
---|---|---|
Chamber Dimensions | ||
RV subcostal wall thickness | >0.5 cm | Figure 14-1, B |
RVOT PLAX proximal diameter | >3.3 cm | Figure 14-1, C |
RV basal diameter (RVD1) | >4.2 cm | Figure 14-1, D |
RA major diameter | >5.3 cm | Figure 14-1, D |
RA minor diameter | >4.4 cm | Figure 14-1, D |
Systolic Function | ||
Tricuspid annular plane systolic excursion (TAPSE) | <1.6 cm | Figure 14-2, A |
Peak lateral TV annular velocity, S′ | <10 cm/s | Figure 14-2, B |
Myocardial performance index (MPI) | ||
Pulsed-wave Doppler imaging | >0.40 | |
Tissue Doppler imaging | >0.55 | Figure 14-2, C |
Fractional area change (FAC) | <35% | Figure 14-2, D |
Diastolic Function | ||
E/A | <0.8 or >2.1 | Figure 14-3, A |
E/E′ | >6 |
A, velocity of active ventricular filling (see Figure 14-3, A); E, velocity of passive right ventricular filling (see Figure 14-3, A); E′, a tissue Doppler measurement of the motion of the lateral tricuspid valve annulus during early diastole (see Figure 14-2, B); PLAX, parasternal long axis; RV, right ventricle; RVOT, right ventricular outflow tract; RA, right atrium; TV, tricuspid valve.
Data from Rudski L, Lai W, Afilalo J, et al: Guidelines for the echocardiographic assessment of the right heart in adults: a report from the American Society of Echocardiography, J Am Soc Echocardiogr 23:685–713, 2010.
Assessment of Right Ventricular Function by Echocardiography
Tricuspid Annular Plane Systolic Excursion
Tricuspid annular plane systolic excursion (TAPSE) is measured using M-mode echocardiography in the apical four-chamber view to generate an image that illustrates the systolic longitudinal displacement of the lateral tricuspid annulus toward the apex (Figure 14-2, A). Because the septal attachment of the tricuspid annulus is relatively fixed, the major component of longitudinal systolic motion occurs at this point. The greater the displacement, the better is right ventricular function—a value less than 16 mm is considered abnormal. TAPSE correlates closely with right ventricular ejection fraction (RVEF) measured by radionuclide angiography, the “gold standard” modality for assessment of right ventricular function. TAPSE is simple to perform, with reproducible results, and is less dependent on optimal image quality than other measurements. It does not require complex calculations (the result obtained may be multiplied by 3.2 to give a value for RVEF, if desired) and can predict likelihood of death among patients with pulmonary arterial hypertension (PAH). However, TAPSE makes the assumption that displacement of a single segment represents function of the entire RV, which does not apply in the presence of regional dysfunction, particularly that affecting the septum. TAPSE is afterload dependent and falls with increasing severity of PAH.
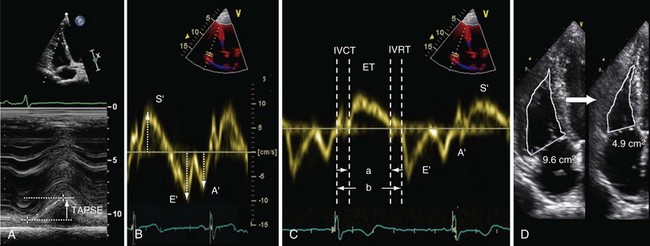
Figure 14-2 Assessment of right ventricular systolic function by various modalities: A, tricuspid annular plane systolic excursion (TAPSE); B, S′ wave measurement by tissue Doppler imaging; C, myocardial performance index (MPI), or Tei index, calculated as isovolumetric relaxation time (IVRT) plus isovolumetric contraction time (IVCT) divided by ventricular ejection time (ET), or a/b − a; D, fractional area change (FAC)—here, from 9.6 cm2 to 4.9 cm2 = 49%. E/E′ is calculated as follows: peak velocity of pulsed-wave Doppler E wave (measured at the tips of the tricuspid valve; see Figure 14-3, A) divided by peak velocity of the E′ wave (measured using tissue Doppler imaging at the lateral tricuspid valve annulus).
Peak Systolic Velocity of Lateral Tricuspid Annulus Displacement
Tissue Doppler imaging (TDI) can be used to measure the velocity of longitudinal displacement of the lateral tricuspid valve annulus during systole (S′) (see Figure 14-2, B). S′ values correlate closely with radionuclide angiography findings, and population-based validation studies indicate that a value less than 10 cm/s is abnormal. The data share the disadvantage of TAPSE in assuming that the velocity of a single wall region reflects the entire function of the complex three-dimensional shape of RV. Velocities are measured using Doppler imaging and therefore can underestimate function if measured off-angle by more than 20 degrees.
Myocardial Performance Index
The right ventricular myocardial performance index (MPI), also known as the Tei index, is the ratio of isovolumetric relaxation time (IVRT) plus isovolumetric contraction time (IVCT) to ventricular ejection time (see Figure 14-2, C). This index gives an accurate, global estimate of both systolic and diastolic right ventricular function. It avoids the need to use the geometric assumptions that are required for volumetric measurement of global right ventricular function, because MPI is based on time intervals from either blood pool Doppler (right ventricular outflow tract [RVOT] and tricuspid valve inflow) or tissue Doppler (lateral tricuspid valve annulus) measurements. A value greater than 0.4 is abnormal for blood pool Doppler, and a value greater than 0.55 is abnormal for tissue Doppler. Advantages of this technique include high reproducibility and the fact that it is relatively unaffected by variation in heart rate. The MPI can be falsely low under conditions of raised right atrial pressure, which may decrease the IVRT.
Fractional Area Change
Fractional area change (FAC) is obtained by tracing the endocardial border of the right ventricle in systole and diastole in the apical four-chamber view (see Figure 14-2, D). The percent FAC is then calculated using the formula (end-diastolic area − end-systolic area/end-diastolic area) × 100%, with a value below 35% considered abnormal. This method correlates closely with magnetic resonance imaging (MRI) assessment of right ventricular function, and reduced FAC is an independent predictor of adverse outcome after pulmonary embolism. The main practical disadvantage arises in defining contours in highly trabeculated RVs.