Pulmonary arterial hypertension is most often diagnosed in its advanced stages because of the nonspecific nature of early symptoms and signs. Although clinical assessment is essential when evaluating patients with suspected pulmonary arterial hypertension, echocardiography is a key screening tool in the diagnostic algorithm. It provides an estimate of pulmonary artery pressure, either at rest or during exercise, and is useful in ruling out secondary causes of pulmonary hypertension. In addition, echocardiography is valuable in assessing prognosis and treatment options, monitoring the efficacy of specific therapeutic interventions, and detecting the preclinical stages of disease.
Pulmonary hypertension (PH) is a hemodynamic and pathophysiologic condition defined as an increase in mean pulmonary artery pressure (MPAP) of ≥25 mm at rest as assessed by right-heart catheterization (RHC). It can be found in multiple clinical conditions with distinct pathogenetic and clinical features, such as pulmonary arterial hypertension (PAH) and left-heart, lung, and thromboembolic diseases ( Table 1 ). In particular, PAH is characterized by the presence of precapillary PH due to relative blood flow obstruction proximal to the lung capillary bed and increased pulmonary vascular resistance (PVR). This results in right ventricular (RV) pressure overload, ultimately leading to right-heart failure and death. PAH has an estimated prevalence of 30 to 50 cases per million individuals, affects women more frequently than men, and can be idiopathic, heritable, drug or toxin induced, or associated with other medical conditions, such as congenital heart disease (CHD), connective tissue disease, human immunodeficiency virus infection, portal hypertension, schistosomiasis, and chronic hemolytic anemia ( Table 2 ).
Definition | Characteristics | Clinical group(s) ∗ |
---|---|---|
PH | MPAP ≥ 25 mm Hg | All |
Precapillary PH | MPAP ≥25 mm Hg PCWP ≤15 mm Hg CO normal or reduced † | 1. PAH 3. PH due to lung disease 4. Chronic thromboembolic PH 5. PH with unclear and/or multifactorial mechanisms |
Postcapillary PH | MPAP ≥ 25 mm Hg PCWP > 15 mm Hg CO normal or reduced † | 2. PH due to left-heart disease |
Passive | TPG ≤ 12 mm Hg | |
Reactive (out of proportion) | TPG > 12 mm Hg |
∗ As defined in Table 2 .
† High CO can be present in hyperkinetic conditions, such as systemic-to-pulmonary shunts (only in the pulmonary circulation), anemia, hyperthyroidism, and so on.
1. PAH |
1.1. Idiopathic |
1.2. Heritable |
1.2.1. BMPR2 |
1.2.2. ALK1, endoglin (with or without hereditary hemorrhagic telangiectasia) |
1.2.3. Unknown |
1.3. Drug and toxin induced |
1.4 Associated with |
1.4.1. Connective tissue diseases |
1.4.2. HIV infection |
1.4.3. Portal hypertension |
1.4.4. Congenital heart disease |
1.4.5. Schistosomiasis |
1.4.6. Chronic hemolytic anemia |
1.5. Persistent PH of the newborn |
1. Pulmonary veno-occlusive disease and/or pulmonary capillary hemangiomatosis |
2. PH due to left-heart disease |
2.1. Systolic dysfunction |
2.2. Diastolic dysfunction |
2.3. Valvular disease |
3. PH due to lung disease and/or hypoxia |
3.1. Chronic obstructive pulmonary disease |
3.2. Interstitial lung disease |
3.3. Other pulmonary diseases with mixed restrictive and obstructive patterns |
3.4. Sleep-disordered breathing |
3.5. Alveolar hypoventilation disorders |
3.6. Chronic exposure to high altitude |
3.7. Developmental abnormalities |
4. Chronic thromboembolic pulmonary hypertension |
5. PH with unclear and/or multifactorial mechanisms |
5.1. Hematologic disorders: myeloproliferative disorders, splenectomy |
5.2. Systemic disorders: sarcoidosis, pulmonary Langerhans cell histiocytosis, lymphangioleiomyomatosis, neurofibromatosis, vasculitis |
5.3. Metabolic disorders: glycogen storage disease, Gaucher disease, thyroid disorders |
5.4. Others: tumoral obstruction, fibrosing mediastinitis, chronic renal failure on dialysis |
Given the nonspecific symptoms and subtle physical signs, particularly in the early stages, a high clinical index of suspicion is necessary to detect the disease before irreversible pathophysiologic changes occur. In this regard, transthoracic echocardiography, by providing direct and/or indirect signs of elevated pulmonary artery pressure (PAP), is an excellent noninvasive screening test for patients with symptoms or risk factors for PH, such as connective tissue disease, anorexigen use, pulmonary embolism, heart failure, and heart murmurs. It may also provide key information on both the etiology and the prognosis of PH.
In this review, we discuss the diagnostic and prognostic role of echocardiography in PAH.
Pulmonary Hemodynamics in the Echocardiography Lab
Table 3 lists Doppler echocardiographic indices for the evaluation of patients with clinical suspicion of PH. Doppler echocardiography enables the reliable estimation of PAP, because in the absence of pulmonary flow obstruction, tricuspid regurgitation (TR) peak velocity (TRV) and RV outflow tract acceleration time have linear positive and negative correlations, respectively, with systolic PAP (SPAP) and MPAP measured by RHC. Furthermore, peak early diastolic and end-diastolic velocities of pulmonary regurgitation correlate significantly with MPAP and pulmonary artery end-diastolic pressures.
Key indices (cutoff) | Additional indices (cutoff) | Complementary indices (cutoff) | Research tools |
---|---|---|---|
Pulmonary hemodynamics | |||
SPAP = 4 × TRV 2 + RAP (TRV > 2.8–2.9 msec: SPAP 36 mm Hg) | MPAP = TVI TR + RAP (≥25 mm Hg) | AT RVOT (<100 msec) log 10 (EPSPAP) = −0.004(PAAT) + 2.1 | PVCAP = SV/4 × (TRV 2 − PRV 2 ) (<0.8 mL/mm Hg predicts mortality in PAH patients) |
RAP = IVC size and collapsibility (>2.1 cm, collapse < 50%: RAP 15 mm Hg) | RV IVRT′ (by DTI; >65 msec: SPAP > 40 mm Hg; <59 msec: RAP > 8 mm Hg) | ||
MPAP = 4 × PRV 2 + RAP (≥25 mm Hg) | MPAP = 0.61 × SPAP + 2 mm Hg | MPAP = 90 − 0.62 × AT RVOT MPAP = 79 − 0.45 × AT RVOT | |
DPAP = 4 × (PRV ED) 2 + RAP | |||
PVR = TRV/TVI RVOT (cm) × 10 + 0.16 (>0.2: PVR > 2 WU; <0.15: normal PVR) | PVR = SPAP/(HR × TVI RVOT ) (>0.076: indexed PVR > 15 RU) | FVE RVOT (midsystolic “notch”) | |
PCWP = 1.9 + 1.24 × E/E′ (E/E′ >15: PCWP > 15 mm Hg) | LAVi (>31 mL/m 2 ) | ||
Impaired RV systolic function | |||
TAPSE (<16 mm) | Tei index: (IVRT + IVCT)/ET (>0.40 by PW Doppler; >0.55 by DTI) | 3D RV EF (<44%) | |
RV FAC (<35%) | RV LPSS (≥−19%) | ||
S′-wave velocity by DTI (<10 cm/sec) |
PVR may be estimated by dividing TRV (in meters per second) by the time-velocity integral of the RV outflow tract (in centimeters). The rationale for this method is based on the recognition that PVR is directly related to pressure changes and inversely related to pulmonary flow. This approach may have utility in distinguishing high PAP due to increased pulmonary blood flow (as occurs in hyperthyroidism, anemia, and obesity) from PH due to elevated PVR. An estimate of PVR may also be valuable for identifying patients with clinically worsening and severe PAH with no change or a decrease in MPAP as a consequence of progressive decrease in RV ejection fraction and stroke volume (PVR = MPAP − pulmonary artery occlusion pressure/cardiac output [CO]). TRV is used in daily practice to determine RV systolic pressure, which is considered equal to SPAP in the absence of pulmonary outflow tract obstruction and/or pulmonic valve stenosis. This is done by calculating the systolic transtricuspid gradient using the modified Bernoulli equation (as simplified by Hatle et al. ) and then adding an assumed or calculated right atrial pressure (RAP). Several studies have shown modest to good correlations between estimated RV systolic pressure and invasively measured pressures ( R = 0.57–0.93), suggesting that technical and biological variability are not negligible. This variability is further reflected in the sensitivity (0.79–1.00) and specificity (0.60–0.98) for diagnosing or ruling out PH. However, to avoid false-positives, it is important to be aware that the resting physiologic range of SPAP is dependent on age and body mass index and may be as high as 40 mm Hg in older (age > 50 years) or obese (body mass index > 30 kg/m 2 ) subjects. The age-related increase in SPAP is more common in patients with diabetes and is likely due to pulmonary artery noncompliance or abnormal left ventricular (LV) diastolic filling pressures occurring with aging and systemic hypertension. An increase in SPAP has a negative impact on survival. Moreover, it should not be overlooked that SPAP is a flow-dependent variable, such as in anemia and hypothyroidism, as a TRV of 3 m/sec is easily achieved in normal subjects at rest after dobutamine infusion.
A few aspects must be kept in mind to ensure accurate estimates of SPAP. Because velocity measurements are angle dependent, TRV should be taken from multiple views (and off axis if necessary), searching for the best envelope and maximal velocity. Additionally, the use of color flow Doppler is recommended to obtain the best alignment between regurgitant flow and the Doppler signal. From the apical position, the transducer must be angled more medially and inferiorly from the mitral valve signal. Although TR is present in >75% of the normal adult population, in case of a trivial regurgitant jet and a suboptimal continuous-wave Doppler spectrum, the injection of contrast agents (agitated saline, sonicated albumin, air-blood-saline mixture) may be required to achieve clear delineation of the jet envelope. Potential overestimation of Doppler velocities should be taken into account because of contrast artifacts. Furthermore, in severe TR with a large color flow regurgitant jet, the peak velocity may not reflect the true RV–right atrial pressure gradient because of early equalization of RV pressure and RAP. Thus, it is recommended to gather technically adequate TR signals and to consider SPAP values in the context of the clinical scenario, searching for other “concordant clinical and echocardiographic signs” of pressure overload ( Table 3 ).
In this regard, the European Society of Cardiology guidelines for the diagnosis and treatment of PH suggest to consider (1) PH unlikely for TRV ≤ 2.8 m/sec, SPAP ≤ 36 mm Hg (assuming RAP of 5 mm Hg), and no additional echocardiographic signs of PH; (2) PH possible for TRV ≤ 2.8 m/sec and SPAP ≤ 36 mm Hg but the presence of additional echocardiographic signs of PH or TRV of 2.9 to 3.4 m/sec and SPAP of 37 to 50 mm Hg with or without additional signs of PH; and (3) PH likely for TRV > 3.4 m/sec and SPAP > 50 mm Hg with or without additional signs of PH.
Echocardiographic Features in Pulmonary Arterial Hypertension
Figure 1 and Table 3 describe echocardiographic features in PAH. Because of chronic RV pressure overload, at the time of diagnosis, most patients present with enlarged right-side chambers, RV hypertrophy, increased interventricular septal thickness, an abnormal interventricular septum/posterior LV wall ratio (>1), and reduced global RV systolic function. Furthermore, the abnormal pressure gradient between the left and right ventricles results in shape distortion and motion of the interventricular septum (“flattening”), which persists throughout the cardiac cycle. As a consequence, the left ventricle appears D-shaped, with reduced diastolic and systolic volumes but preserved global systolic function. Pericardial effusion and mitral valve prolapse have also been described in patients with PAH; the former may be a manifestation of impaired venous and lymphatic drainage secondary to elevated RAP, and the latter is related to a small left ventricle and the possible involvement of valve leaflets affected by associated connective tissue disorders.

At the time of definitive diagnosis, most patients with PAH show at least moderate TR, with SPAP ≥ 60 mm Hg. TR is usually caused by tricuspid annular dilation, altered RV geometry, and apical displacement of the tricuspid leaflets. The degree of TR cannot be used as a surrogate for the degree of PAP elevation.
Significant pulmonic valvular regurgitation is common in PAH. Pulsed-wave Doppler interrogation of the RV outflow tract usually reveals an acceleration time of <100 msec, which reflects abnormal MPAP. Finally, as a result of altered RV-LV interaction, LV diastolic dysfunction may be characterized by a marked dependence of LV filling on atrial contraction.
Transesophageal Echocardiography
Transesophageal echocardiography should be considered in the following circumstances: (1) to confirm and assess congenital systemic-to-pulmonary shunts, (2) to assess the severity and contribution of mitral valve disease, (3) to characterize a right-sided intracardiac mass not well visualized with transthoracic echocardiography or other imaging techniques, and (4) to guide interventional procedures, such as balloon atrial septostomy.
Echocardiographic Features in Pulmonary Arterial Hypertension
Figure 1 and Table 3 describe echocardiographic features in PAH. Because of chronic RV pressure overload, at the time of diagnosis, most patients present with enlarged right-side chambers, RV hypertrophy, increased interventricular septal thickness, an abnormal interventricular septum/posterior LV wall ratio (>1), and reduced global RV systolic function. Furthermore, the abnormal pressure gradient between the left and right ventricles results in shape distortion and motion of the interventricular septum (“flattening”), which persists throughout the cardiac cycle. As a consequence, the left ventricle appears D-shaped, with reduced diastolic and systolic volumes but preserved global systolic function. Pericardial effusion and mitral valve prolapse have also been described in patients with PAH; the former may be a manifestation of impaired venous and lymphatic drainage secondary to elevated RAP, and the latter is related to a small left ventricle and the possible involvement of valve leaflets affected by associated connective tissue disorders.
At the time of definitive diagnosis, most patients with PAH show at least moderate TR, with SPAP ≥ 60 mm Hg. TR is usually caused by tricuspid annular dilation, altered RV geometry, and apical displacement of the tricuspid leaflets. The degree of TR cannot be used as a surrogate for the degree of PAP elevation.
Significant pulmonic valvular regurgitation is common in PAH. Pulsed-wave Doppler interrogation of the RV outflow tract usually reveals an acceleration time of <100 msec, which reflects abnormal MPAP. Finally, as a result of altered RV-LV interaction, LV diastolic dysfunction may be characterized by a marked dependence of LV filling on atrial contraction.
Transesophageal Echocardiography
Transesophageal echocardiography should be considered in the following circumstances: (1) to confirm and assess congenital systemic-to-pulmonary shunts, (2) to assess the severity and contribution of mitral valve disease, (3) to characterize a right-sided intracardiac mass not well visualized with transthoracic echocardiography or other imaging techniques, and (4) to guide interventional procedures, such as balloon atrial septostomy.
Exercise-Induced Pulmonary Hypertension: Looking beyond the Scene
The pulmonary circulation is a high-flow, low-pressure, low-resistance system. PVR is approximately one tenth of comparable systemic values. In healthy subjects, moderate exercise induces mild increases in PAP that are linear with CO and decreases in PVR secondary to the dilation of compliant small vessels and/or the recruitment of additional vessels in the upper portion of normal lungs.
In elite athletes, substantial increases in PAP have been shown to occur during intense exercise as a result of marked increases in pulmonary blood flow along with increases in LV filling pressure. This “physiologic counteraction” may cause an impairment of the integrity of the pulmonary blood-gas barrier (pulmonary capillary “breaking stress”), with the development of exercise-induced pulmonary hemorrhage. Reported upper normal limits of Doppler-derived SPAP during exercise are <40 to 45 mm Hg in normal healthy individuals and <55 to 60 mm Hg in highly trained athletes. It is worth noting that PVR varies from one subject to another. Accordingly, the increase in PAP as a function of CO during exercise is quite variable as well, with reported slopes of PAP as a function of flow ranging from 0.5 to 2.5 mm Hg/L/min. There has been recent suggestion that steep increases in PAP may be a limiting factor for exercise capacity because of persistent limitations in RV output. Competitive athletes who exercise at high levels of CO and PAP because of an intrinsically steep pressure-flow relationship may be exposed in the long term to RV remodeling and subsequent arrhythmias.
A number of investigators have reported subsets of patients with pathologic SPAP responses during exercise and normal SPAP at rest; this is referred to as exercise-induced PH ( Table 4 ). Recently, using invasive maximum cardiopulmonary exercise testing, Tolle et al. carefully phenotyped a large cohort of patients with exercise-induced PAH. In particular, they showed that at maximum exercise, oxygen uptake was lowest in resting PAH, intermediate in exercise-induced PAH, and highest in normal subjects, whereas MPAP and PVR followed the opposite pattern. These findings suggest that exercise-induced PAH is a mild, intermediate physiologic stage of PAH, with greater vasodilator reserve and less severe degrees of pulmonary vascular disease. However, exercise-induced PAH is rarely considered because of the lack of specific symptoms or signs. Exercise Doppler echocardiographic evaluation should be performed in patients with modest changes in exercise capacity with no other identifiable causes, particularly in asymptomatic or mildly symptomatic subjects at high risk for PAH (i.e., first-degree relatives with PAH, carriers of the PAH gene, patients with human immunodeficiency virus infection, and patients with connective tissue diseases). Although exercise Doppler echocardiography has been proved to be a safe and viable screening tool for detecting a pulmonary hypertensive response to exercise in high-risk patients, it remains technically demanding and requires considerable experience. It should also be emphasized that the optimal exercise protocol (treadmill vs supine, upright, or semirecumbent bicycle) has not been established. Importantly, data derived during or after bicycle exercise should not be applied to posttreadmill or recumbent exercise stress echocardiography, because each protocol is characterized by different loading conditions. However, in the assessment of PH, exercise must be dynamic (cycling or walking) rather than resistive (handgrip, weight lifting). Resistive exercise should be avoided because it results in decreased venous return during the Valsalva maneuver and little change in maximal oxygen uptake and CO but increased systemic vascular resistance and an eventual increase in intrathoracic pressure, each of which may contribute to elevated MPAP at any given level of flow. A resistive component at high levels of dynamic exercise may spuriously increase MPAP at given levels of CO. Although the results of MPAP on exercise are often expressed for a given workload, results of exercise hemodynamics might be better expressed in MPAP as a function of flow (e.g., millimeters of mercury per liter) rather than as a function of workload, given that the mechanical efficiency of muscle contraction varies from one subject to another. Recently, Argiento et al. , in a series of 113 healthy volunteers (mean age, 37 ± 13 years; range, 19–63 years; 57 women [50%]) reported exercise flow-corrected upper limits of normal for MPAP of 34 mm Hg at a CO of <10 L/min, 45 mm Hg at a CO of <20 L/min, and 52 mm Hg at a CO of <30 L/min. All subjects underwent exercise echocardiographic testing on a semirecumbent cycle ergometer with workload increments of 20 to 30 W every 2 min until the maximum tolerated before the onset of dyspnea and/or leg pain. Echocardiographic measurements were taken during the last minute of each workload.
Study | Associated disease | n | Age (y) | Height (cm) | Weight (kg) | Exercise protocol | RAP estimate (mm Hg) | Baseline SPAP (mm Hg) | Peak SPAP (mm Hg) |
---|---|---|---|---|---|---|---|---|---|
Himelman et al. (1989) | COPD | 36 (15 female) | 32–80 | Supine bicycle (10 or 25 W/2 incr) | From IVC | 46 ± 20 (ctrl 22 ± 4) | 83 ± 30 (ctrl 31 ± 7) | ||
Oelberg et al. (1998) | Asymptomatic ASD | 10 (4 women) | 52.9 ± 11.2 | 167 ± 7 | 82 ± 20 | Upright bicycle (10 W/2 incr) | From IVC | 31 ± 8 (ctrl 17 ± 8) | 51 ± 10 (ctrl 19 ± 8) |
Grünig et al. (2000) | HAPE-S | 9 men | 45 ± 8 | 182 ± 8 | 82 ± 9 | Supine bicycle (25 W/2 incr) | Fixed value (5 mm Hg) | 28 ± 4 (ctrl 27 ± 4) | 55 ± 11 (ctrl 36 ± 3) |
Grünig et al. (2000) | Relatives of IPAH cases | 52 | Supine bicycle (25 W/2 incr) | Fixed value | 24 ± 4 (NR); 23 ± 3 (AR) | 37 ± 3 (NR); 56 ± 11(AR) | |||
Collins et al. (2006) | Scleroderma | 51 (49 women) | 53.9 ± 12.0 | Treadmill (5.9 ± 1.9 METs) | Fixed value (10 mm Hg) | 24 ± 8 | 38 ± 12 | ||
Alkotob et al. (2006) | Scleroderma | 65 (56 women) | 51 ± 12 | Treadmill (1 to 13.4 METs) | Fixed value (5 mm Hg) | 25 ± 8 | 39 ± 8 | ||
Kiencke et al. (2008) | HAPE-S | 10 | 33 ± 2 | Supine bicycle | 19 ± 4 (ctrl 17 ± 3) | 23 ± 6 (ctrl 11 ± 5) | |||
Steen et al. (2008) | Scleroderma | 54 (51 women) | Treadmill (85% pred max HR) | Fixed value (10 mm Hg) | 34.5 ± 11.5 | 51.4 | |||
Grünig et al. (2009) | Relatives of IPAH patients | 291 (125 women) | 37 ± 16 | 169 ± 9 | 69 ± 15 | Supine bicycle (25 W/2 incr) | From IVC | 20.7 ± 5.4 (ctrl 20.4 ± 5.3) | 39.5 ± 5.6 (ctrl 35.5 ± 5.4) |
Reichenberger et al. (2009) | Scleroderma | 33 (31 women) | 54 ± 11 | Supine bicycle (30 W/2 incr) | From IVC | 23 ± 8 | 40 ± 11 | ||
Möller et al. (2010) | ASD and VSD | 44 (25 female) | 17.5 ± 3.3 | 167 ± 8.8 | 59 ± 11 | Supine bicycle (25 W/2 incr) | From IVC | 20.7 ± 5.3 (ctrl 21.8 ± 3.6) | 37 (24–76) (ctrl 39 [17–63]) |
Kovacs et al. (2010) | Connective tissue disease | 52 (42 women) | 54 ± 11 | 167 ± 8 | 69 ± 12 | Supine bicycle (25W/2 incr) | From IVC | 27 ± 5 ∗ ; 23 ± 3 † ; 23 ± 3 ‡ | 55 ± 10 ∗ ; 29 ± 8 † ; 30 ± 7 ‡ |
D’Alto et al. (2011) | Systemic sclerosis | 172 (155 women) | 51.8 ± 21.5 | 163 ± 9 | 66 ± 14 | Supine bicycle (25W/2 incr) | From IVC | 26.2 ± 5.3 (ctrl 20.6 ± 3.7) | 36.9 ± 8.7 (ctrl 25.9 ± 3.3) |
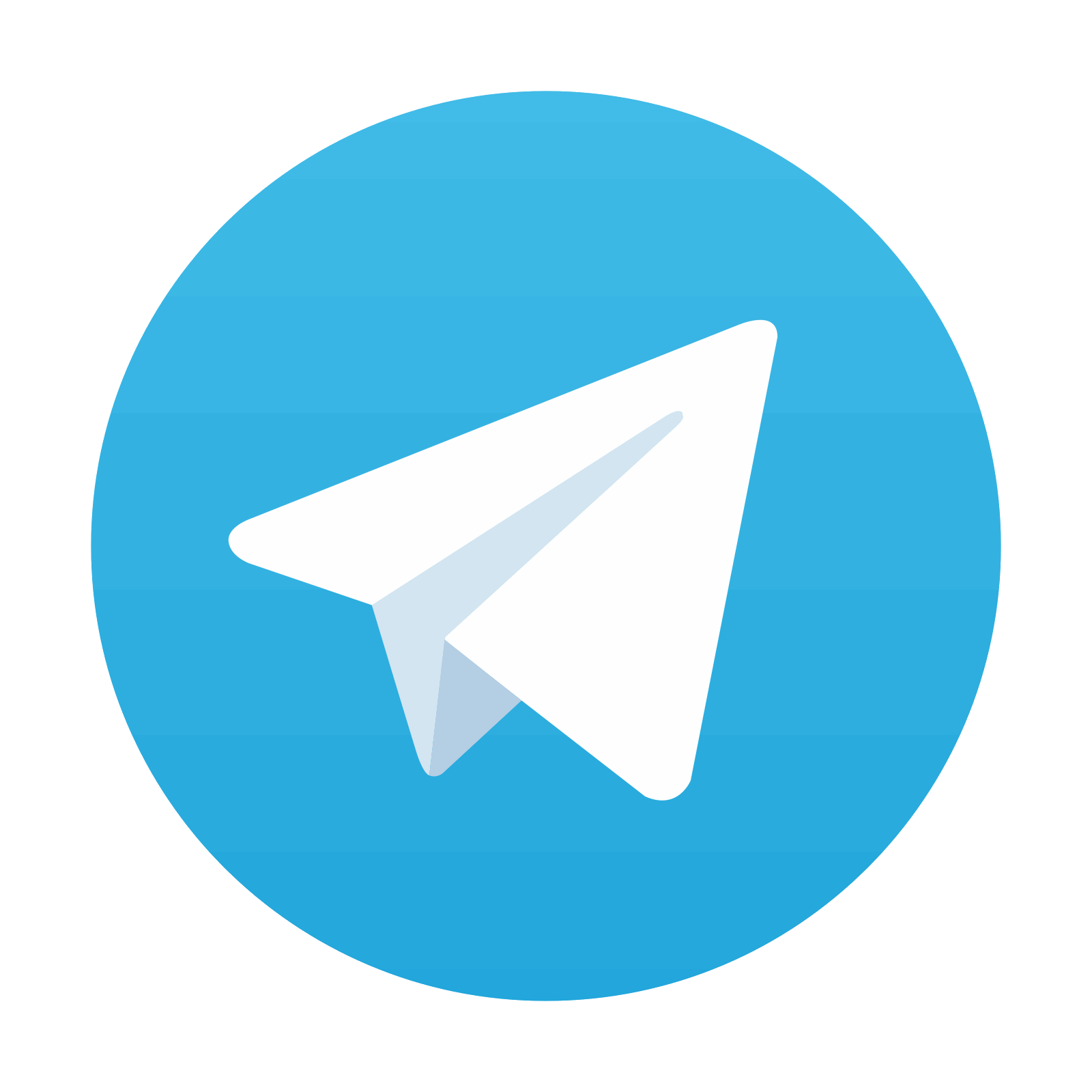
Stay updated, free articles. Join our Telegram channel

Full access? Get Clinical Tree
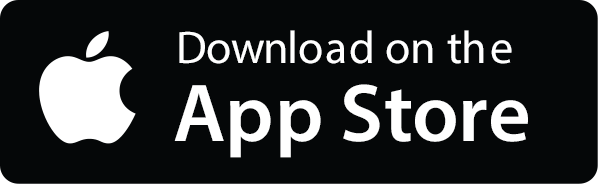
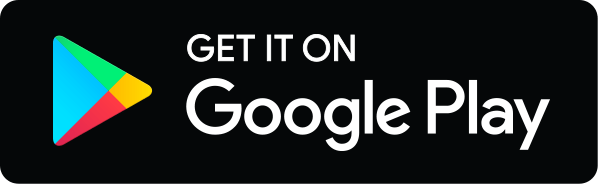
