Education is the path from cocky ignorance to miserable uncertainty. —Mark Twain
The Need for an Adequate Measure of Dyssynchrony
Ventricular dysfunction leading to heart failure can affect children with both congenital and acquired heart disease. Treatment options for both groups remain limited. The role of medical therapy, although established for adult heart failure, remains less certain in children. Although they are widely used, the benefit of β-blockers and angiotensin receptor inhibitors remains a topic of debate in children with heart failure. Cardiac transplantation, although well established in children, carries associated morbidities, and the limited availability of donor organs remains a concern. Therefore, when cardiac resynchronization therapy (CRT) emerged as an effective treatment for adults with heart failure, it sparked considerable interest among pediatric cardiologists.
However, we soon learned from our colleagues caring for adult patients that although CRT was an effective therapy in some adults with systolic heart failure, roughly a third of patients did not benefit when electrocardiographic QRS duration was used to predict response. Similarly, in retrospective pediatric studies, baseline QRS duration was not associated with improvement in ventricular response to CRT. At the same time, the need to predict which patients will respond to CRT seemed even more pressing in children because of the added complexities and risk for complications associated with pacemaker implantation in children. Furthermore, the potential for worsening ventricular function and clinical status after CRT in some patients emphasized the need to choose candidates carefully.
At first it seemed simple. Echocardiography would step in where electrocardiography had fallen short. Echocardiography would directly and reliably quantify wall motion abnormalities, and the heterogeneous timing of contraction between different ventricular segments, in a comprehensive manner that reflected the actual myocardial mechanics. This would reduce our reliance on an indirect, nonspecific, averaged signal of electrical activation that had failed in so many patients. The enthusiasm for echocardiographic measurement of ventricular dyssynchrony was fueled by the increasing availability of newer techniques to quantify regional myocardial motion, velocities, and deformation, such as Doppler tissue imaging (DTI) and two-dimensional speckle-tracking. We could now not only quantify regional myocardial performance but also evaluate its exact timing, thereby allowing us to measure the disparity in the timing of contraction between segments. These methodologies seemed especially applicable to children, as they were independent of assumptions regarding ventricular geometry, which is often grossly abnormal in congenital heart disease. Evolving technologies, including three-dimensional echocardiography, three-dimensional strain, and vector velocity imaging joined the festivities. The array of technologies produced an explosion of multiple dyssynchrony measures and indices, as well as confusion regarding which to use. Some dyssynchrony indices became more accepted, on the basis of convincing single-center results, promoted with confidence in the literature and at professional meetings, and backed by recommendations endorsed by the American Society of Echocardiography. However, this enthusiasm was subsequently dampened by the Predictors of Response to Cardiac Resynchronization Therapy (PROSPECT) and Resynchronization Therapy in Normal QRS (RETHYNQ) trials, and things became a lot murkier in the world of echocardiographic assessment of mechanical dyssynchrony. Despite criticisms that have been raised regarding the methodologies of PROSPECT and RETHYNQ, both trials indicated that commonly used echocardiographic dyssynchrony measures were inadequate to predict response to CRT. These trials forced us to revisit the very terminology of dyssynchrony, its significance, mechanisms, and impact, and how to measure it.
Dyssynchrony in Children
Against this background, those of us caring for children, with both acquired and congenital heart disease, asked and continue to ask, how do these issues apply to our patients? The pediatric heart failure population is far more heterogeneous than the adult heart failure population. Most children receiving CRT have congenital heart disease with widely varying morphologies. Different structural and hemodynamic lesions, the relatively rare occurrence of frank ischemic heart disease, the more common occurrence of right versus left bundle branch block, single-ventricle versus biventricular physiology, systemic versus subpulmonary right ventricles, the presence of heart block, and the presence of pacemakers in many children undergoing CRT are some of the potential factors influencing the assessment of mechanical dyssynchrony and also the potential response to CRT. Therefore, the already very complex problem in adults is even more complicated in the pediatric population with acquired and congenital heart disease. In this issue of the Journal of the American Society of Echocardiography , two studies on the echocardiographic assessment of mechanical dyssynchrony, in two very different pediatric populations, highlight some important issues and difficulties in the assessment of mechanical dyssynchrony in children.
Thomas et al. studied seven mechanical dyssynchrony measures in 60 healthy children and in 11 children with dilated cardiomyopathy (DCM). The dyssynchrony measures included commonly used DTI measures such as the septal-lateral delay, peak velocity difference, and standard deviation of time to peak velocity in 12 segments in systole and in diastole. The investigators found that dyssynchrony measures were correlated with age only weakly or not at all. Moreover, even weak correlations became apparent only after correcting the dyssynchrony measure for heart rate. The investigators found that “traditional” DTI measures diagnosed dyssynchrony in 57% to 85% of normal children when using adult cutoff values, similar to the proportion found in the small sample of patients with DCM. Conversely, the standard deviation of time to peak diastolic velocity in diastole identified only 3% of normal children as having dyssynchrony. Thomas et al. also measured the cross-correlation delay (XCD) in systole, in diastole, and throughout the entire cardiac cycle, which previously has been proposed by this group as a measure of dyssynchrony in adults. As opposed to the more widely used DTI dyssynchrony measures, which assess the timing of a single point during the cardiac cycle (e.g., time to onset or peak of the systolic tissue velocity), the XCD measures the relative shift in velocity curves between segments through the cardiac cycle or a portion of the cycle, such as systole or diastole. If two regions show similar motion and velocities, their velocity curves can be superimposed one on the other, yielding high cross-correlation. If regions are dyssynchronous and move at varying times, the velocity curve from one region will be time shifted compared to curves from other regions, and the cross-correlation will be low. Accordingly, the normalized correlation coefficient ranges from +1 (reflecting perfect synchrony) to −1 (reflecting absolute dyssynchrony). In comparison with the more common DTI measures, XCD showed less dyssynchrony in normal children compared with those with DCM and therefore may be potentially more useful.
One may question the purpose of measuring dyssynchrony in a healthy population, as these individuals would not receive CRT even if dyssynchrony were found. Likewise, the study was not designed to define normal reference values, which may then be used to define the presence of dyssynchrony in other populations. In adult studies, dyssynchrony cut points to predict response to CRT have been derived in the target cardiomyopathy population rather than in a normal reference population. The 11 children with DCM included in Thomas et al. ‘s study constitute a very small group, and mechanical dyssynchrony has previously been found to be prevalent in this population. Still, beyond the relatively novel application of XCD, the study highlights several important problems in assessing mechanical dyssynchrony in children, and it raises several questions.
First, are methodologies and cutoff values used to assess mechanical dyssynchrony in adult patients applicable in children? Even though dyssynchrony parameters were worse in patients with DCM than in controls, suggesting that they may be able to discriminate normal from abnormal, it seems unlikely that a dyssynchrony cutoff value that identifies the majority of normal children as having dyssynchrony would be useful and/or sufficiently predictive of response to CRT in a pediatric heart failure population. Although this does not automatically mean that the methodology itself is not useful (that is the subject of additional discussion), Thomas et al. correctly conclude that these values would need to be used with extreme caution in children.
Second, should dyssynchrony measures be corrected for heart rate in children? In contrast to adult assessment of mechanical dyssynchrony, in which absolute cut points are used, it seems intuitive that this cannot be the case in children of different ages and with varying heart rates. For example, when a standard deviation is calculated from shorter time intervals (as occurs with faster heart rates), the standard deviation value will be lower, complicating the use of an absolute cutoff in children. Larger studies have investigated the relation between cardiac timing intervals, heart rate, and age in children. Still, the optimal method to correct the dyssynchrony measure for heart rate remains poorly defined. If Bazett’s method is used (by dividing the dyssynchrony measure by the square root of the R-R interval), as in Thomas et al. ‘s study, the resulting value will increase when the heart rate is <60 beats/min. Should Z scores should be developed for timing intervals or dyssynchrony indices, as has been done with other quantitative parameters to address the influence of childhood growth? Perhaps so; however, even then, it can be debated whether Z scores should be based on age or body size, and how to account for heart rate, which is the predominant factor influencing timing intervals. For example, a 2-year-old child taking a β-blocker may have a slower heart rate than an untreated 5-year-old with ventricular dysfunction and tachycardia. Furthermore, even if one were able to define dyssynchrony on the basis of Z scores, this would not automatically translate into an improved ability to predict response to CRT. As will be subsequently discussed, a single value, (be it a Z score, a threshold, or an XCD) does not adequately define the pattern and mechanism of dyssynchrony and therefore may not adequately predict response to CRT.
Thomas et al. ‘s study suggests that the XCD methodology may be advantageous compared with single-point measurements. Experience has shown that dyssynchrony measures based on time to peak (or time to onset) intervals have low reproducibility. This problem may be especially prominent in pediatric DCM, in which the wall is thin and the amplitude of motion is low, heart rates are fast, and DTI curves can be “noisy.” Furthermore, velocity curves from different regions in the heart normally have different patterns, which may lead to false identification of a “delay” between peaks, even though the segments are synchronous. Therefore, analyzing wall motion continuously over a longer segment of the cardiac cycle, rather than at a single point, seems inherently attractive. Conversely, as previously mentioned, a statistical correlation, even if it shows imperfect superimposition of curves, does not address the pattern and mechanism of dyssynchrony, which is emerging as an important factor in predicting response to CRT. In this regard, XCD considered in isolation may be inadequate to define clinically meaningful dyssynchrony.
Thomas et al. ‘s study highlights an additional important topic. To date, we have focused on assessment of systolic dyssynchrony, with little attention to diastole. However, patients with DCM have both systolic and diastolic dysfunction, and children with DCM have diastolic dyssynchrony that may be associated with adverse outcomes. Furthermore, systolic abnormalities may lead to diastolic abnormalities (and vice versa), and, as implied by Thomas et al. ‘s study, dyssynchrony may exist throughout the cardiac cycle. These postulates are not unreasonable, as abnormal electrical depolarization can lead to abnormal repolarization; moreover, mechanical systolic-diastolic coupling through torsion, recoil, and other mechanisms is well recognized. Some years ago, our group attempted to address this issue with rather cumbersome DTI measurements in systole and diastole, as well as combining systole and diastole to evaluate the entire duration of the cardiac cycle. XCD does this more elegantly than the methodology we proposed and with higher potential for clinical application. Nonetheless, in Thomas et al. ‘s study, the relation between systolic and diastolic XCDs was weak. The mechanisms driving systolic dyssynchrony may be independent from those driving diastolic dyssynchrony. This topic is difficult to study, as both mechanical dyssynchrony and diastolic dysfunction are difficult to diagnose in children.
The complexity of evaluating mechanical dyssynchrony in children is further emphasized in the study Gokhale et al. , who investigated QRS duration and mechanical dyssynchrony in 26 children with hypoplastic left heart syndrome (HLHS) after the Fontan operation. As in Thomas et al. ‘s study, Gokhale et al. used multiple techniques to measure dyssynchrony, including DTI (the difference in the interval from QRS to the onset of the s′ wave between the hypoplastic left ventricle and the dominant right ventricle), time to peak strain, time to peak systolic strain rate, and the standard deviations of times to peak strain and strain rate between segments. The associations of these indices with an array of right ventricular (RV) functional parameters (including DTI s′ wave, DTI RV myocardial performance index, global strain, global strain rate, and RV fractional area change) were investigated. The study found that QRS duration, and some mechanical dyssynchrony indices, correlated weakly with several RV functional indices.
Children with HLHS are certainly a worthwhile population to study. Among those with congenital disease, patients with HLHS are a high-risk group, and RV function is a key determinant of outcomes. It is worthwhile to note, however, that most mortality in HLHS occurs around stage 1 of surgical palliation (e.g., the Norwood procedure) and between stage 1 and stage 2 of surgical palliation. In Gokhale et al. ‘s study, only patients after the Fontan operation (stage 3 of surgical palliation) were included, and therefore these patients are a lower risk subset. Moreover, diastolic dysfunction and abnormal ventricular-vascular coupling may affect Fontan patients more than systolic dysfunction. Additionally, it is difficult to comment on the severity of RV dysfunction in Gokhale et al. ‘s study population, even though some parameters were lower than published normative data from subpulmonary right ventricles. This illustrates one difficulty in investigating dyssynchrony and function in congenital heart disease: should RV parameters of function and dyssynchrony in HLHS be compared with normative RV data? Is the single systemic right ventricle more like a left ventricle or a right ventricle, or dissimilar to either? Can there be “normative” data for the systemic right ventricle, which is an intrinsically abnormal condition?
Are Gokhale et al. ‘s findings new? Mechanical dyssynchrony has previously been described in the Fontan population by angiography and echocardiography. Specifically in patients with HLHS, both a wide QRS interval and mechanical dyssynchrony have previously been described in association with interstage RV dysfunction. However, there is a lack of data demonstrating that reversal or alleviation of either electrical or mechanical dyssynchrony leads to improved ventricular function in this population. The reasons for mechanical dyssynchrony in HLHS remain poorly defined. Altered loading conditions between the palliative stages may influence disparate contraction. In addition, regional variations in wall stress, abnormal segmental interaction, ischemia, and perhaps fibrosis may all potentially influence regional heterogeneity in the timing of segmental contraction. Which of these reflects contractile disparity and which are related to mechanical dyssynchrony remains unclear. Prinzen et al. differentiated between dyssynchrony , used for the timing of contraction, as previously described for the timing of onset or peak shortening, and discoordination , defined as opposing shortening and stretch within the ventricle. Gokhale et al. ‘s study suggests that electrical activation abnormalities contribute to regional contractile delay, as there was a relation between QRS duration and time to peak regional strain. This relation has been elusive to characterize in the pediatric population in general, and prior studies in children with HLHS have not found a clear relation between parameters of mechanical dyssynchrony and QRS duration. Of note, the mean QRS duration in Gokhale et al. ‘s study was 96 msec, which is not particularly prolonged for the age range of the study population. Nonetheless, the relation between electrical activation and mechanical abnormalities suggests that in some patients with HLHS, there may be potential for reversal of mechanical abnormalities with pacing. We still do not have a clear idea of how to select these patients or if QRS morphology, beyond its duration alone, is important.
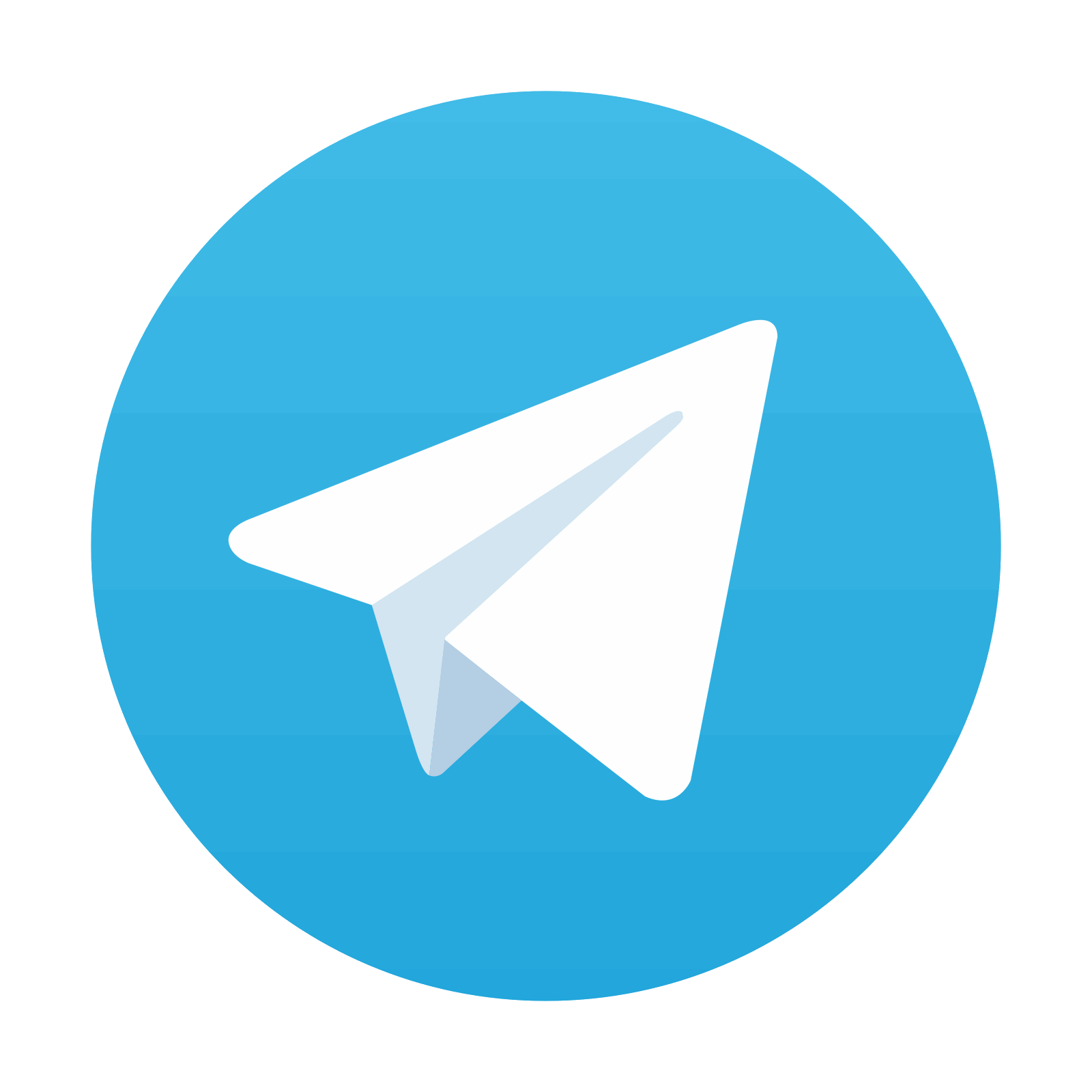
Stay updated, free articles. Join our Telegram channel

Full access? Get Clinical Tree
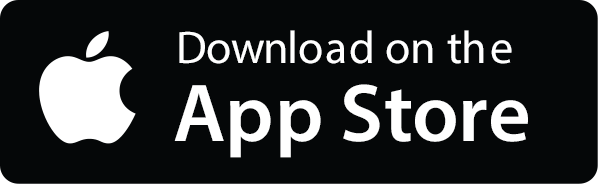
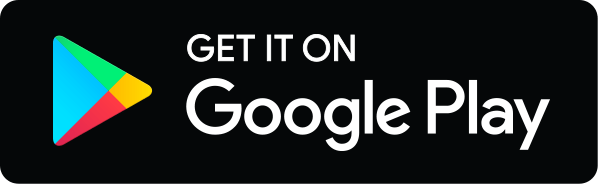