It has been more than 25 years since Hegrenaes and Hatle and Currie et al revolutionized the diagnostic approach to aortic stenosis by demonstrating an excellent correlation between aortic valve systolic gradients determined noninvasively, using continuous wave Doppler and the modified Bernoulli equation, with invasively measured gradients. In 1989, Burstow et al proved a similar correlation between noninvasively and invasively determined systolic gradients across aortic valve prostheses. In each of these investigations a key component of continuous wave Doppler methodology was aligning the Doppler beam as close to parallel as possible with the direction of the systolic jet through the aortic valve. This requires interrogation of the aortic valve from multiple transducer positions, including apical, right parasternal, right supraclavicular, and suprasternal notch.
Because the gradient across stenotic aortic valves depends not only on the degree of orifice narrowing but also on the flow across the valve, the assessment of aortic valve stenosis is incomplete without measurement of the aortic valve area (AVA). In 1985, Skjaerpe et al, and later Oh et al, demonstrated clinically acceptable correlation between aortic valve areas determined noninvasively with echocardiography and Doppler, using the continuity equation, and valve areas determined invasively, using the Gorlin equation. In 1990, Rothbart et al demonstrated a similar correlation between these two methods for aortic bioprostheses.
For assessment of AVA the now familiar continuity principle is utilized. As a review, let us first consider volume flow through the left ventricular outflow tract (LVOT) during systole. If we were able to view the aortic annulus just before valve opening we would see it occupied by a disc of blood. With valve opening and ejection more discs would appear at the annulus—an increasing number per unit time during blood flow acceleration and a decreasing number per unit time with flow deceleration. If we could save all the discs that had appeared at the annulus during ejection and pile them up we would have a cylinder. The area of this cylinder is the LVOT area at the aortic annulus. The number of discs in the pile is determined by the LVOT velocity profile, and if we integrate this velocity profile, calculating the LVOT time velocity integral (TVI), we obtain the length of our cylinder. The volume of this cylinder, which is the stroke volume, is the product of its area and length: the LVOT area times the LVOT TVI.
Similarly, if we start our observation with the smaller disc of blood occupying the narrowed aortic valve area just before ejection, and collected all the discs passing through during ejection, we could make a pile of discs whose area is the aortic valve area and whose length is the TVI of the velocity profile through the narrowed orifice. The continuity principle requires that the volume of blood traveling through the left ventricular outflow tract during ejection is the same as the volume of blood traveling through the narrowed valve orifice. In order for the stroke volume to pass through the narrowed aortic orifice during ejection it has to accelerate, and hence with significant stenosis the velocity profile through the valve has a much higher peak than the LVOT profile, and therefore, a much larger TVI. This means that our second cylinder is long and narrow compared to our LVOT cylinder. Despite the difference in shape, the volume of this second cylinder, obtained by multiplying the aortic valve area times the aortic valve TVI, is also the stroke volume.
Since the volumes of these two cylinders are equal, we can equate their mathematical expressions: the AVA times the aortic valve TVI is equal to the LVOT area times the LVOT TVI. Solving for AVA, we divide the product of the LVOT area and TVI (the stroke volume) by the aortic valve TVI. As blood speeds through a narrowed valve orifice, frictional forces at the edge of the orifice lead to contraction of the jet. The jet is smallest just beyond the orifice, at the vena contracta, where pressure is lowest and velocity is highest. Importantly, since we obtain the aortic valve velocity by continuous wave Doppler, which provides us with the highest velocity along its path, the velocity profile that we obtain when directing our beam through the valve is actually the velocity profile of the vena contracta, just beyond the valve orifice. Hence the area that we calculate with this method is the area of the vena contracta. This is why, for both native and prosthetic aortic valves, we calculate an effective orifice area (EOA), which is slightly smaller than the valve geometric orifice area.
To calculate the numerator of the valve area formula we must first calculate the LVOT area by measuring its diameter. With native aortic valves the LVOT diameter is measured just below the valve and with most aortic valve prostheses the LVOT diameter is measured just below the sewing ring. In each case the measurement is carried out from a parasternal long axis view that includes the LVOT, the valve, and the proximal ascending aorta. The transducer is manipulated for slight medial and lateral scanning until the largest diameter is identified and the image is then frozen in systole.
The LVOT diameter is measured using landmarks that are most likely to provide reproducibility. For native aortic valves the LVOT diameter is measured by placing one caliper anteriorly, at the junction of the right aortic cusp with the ventricular septum and a second caliper at the junction of the posterior cusp with the anterior mitral leaflet. For prostheses with sewing rings, the LVOT diameter is measured by placing one cursor at the junction of the anterior sewing ring with the ventricular septum and the second cursor at the junction of the of the posterior sewing ring with the anterior mitral leaflet. Rothbart et al demonstrated that this measurement is highly feasible and that this method using the measured LVOT diameter for aortic prostheses yields results that correlate better with invasive measurement of valve area when compared to the method that substitutes the size of the prosthesis for the measured LVOT diameter. Therefore, the method utilizing the measured LVOT diameter is recommended.
After measuring the LVOT diameter we need to measure the LVOT TVI to complete the numerator of the valve area equation. While the LVOT velocity profile should ideally be obtained at the same level used for measurement of the LVOT diameter, this is not usually entirely possible for stenotic native aortic valves and for many aortic valve prostheses, because of the zone of flow convergence that forms just below these valves. In the approximately circular area just proximal to the valve, flow convergence results in large velocities centrally and lower velocities toward the periphery. As Skjaerpe et al elucidated in the original manuscript on calculation of aortic valve area by echocardiography and Doppler, the continuity principle requires that the velocity profile that is used to calculate the LVOT TVI must be the spatial average velocity within the circular area. In order to obtain such a profile we have to drop the pulsed wave sample volume from just below the valve to a position just below the zone of flow convergence—usually 0.5 to 1.0 cm below the cusps. Correct positioning is often accomplished by placing the sample volume just below the valve cusps and then creeping it lower until the peak velocity abruptly decreases, correlating with a position below the convergence zone. While use of this sample volume position means that we are measuring the LVOT velocity profile in a slightly different position from which we measure the diameter, this method has held up when compared to invasive measurements of EOA, and thus is recommended.
The denominator for the AVA calculation, the aortic valve TVI, is acquired at the same time that the velocity profile, obtained from the continuous wave Doppler transducer position yielding the highest velocity, is traced to determine the mean gradient. With this trace the ultrasound machine not only calculates the mean gradient, using the Bernoulli equation, but also performs simple integration of the velocity spectrum, thereby yielding the aortic valve TVI.
In this issue of the Journal , Shames et al detail the unique pulsed wave sample volume positioning that is required to correctly calculate the EOA of the SAPIEN valve (Edwards Lifesciences, Irvine, CA). This valve is one of the first examples of a new generation of aortic valve prostheses that have been designed for transfemoral or transapical implantation. It consists of bovine cusps mounted inside a stent. When implanted, the stent extends below the cusps, into the LVOT.
This design leads to velocity acceleration both within the stent and through the cusps. In order to calculate the EOA of the SAPIEN valve the LVOT stroke volume is measured immediately below the lower border of the stent. The diameter is measured at this location and the LVOT velocity must be measured using a pulsed wave Doppler sample volume positioned in this same location (the authors found no zone of central flow convergence in this location). This requires methodology that differs from that discussed above. If the sample volume is mistakenly positioned below the valve cusps and carefully moved lower until the velocity decreases, it will still be within the stent, in a zone of increased velocity.
Shames et al document that use of this incorrect sample volume position leads to calculation of a falsely large (often supra-physiologic) stroke volume and hence a falsely large EOA. Furthermore, unless the correct sample position is consistently identified during interrogation of SAPIEN prostheses, there is a risk that the calculated EOA will erroneously vary from one exam to another on the same patient. In this manuscript we are given two very useful pointers for avoiding incorrect sample volume positioning. First, full screen imaging of the SAPIEN valve should be used to verify sample volume positioning just below the stent, before switching to the spectral Doppler mode. Secondly, while the patient is still available for additional examination, the stroke volume index should be calculated from the measured LVOT diameter and TVI, to be certain that it falls within reasonable range for the patient’s left ventricular size and ejection fraction. The authors also recommend that, although the velocity profile within the stent should not be used to calculate the EOA, this velocity profile should be measured and recorded with each examination, as a means of surveillance for in-stent, pre-cusp stenosis.
We have entered the exciting era of transcutaneous aortic valve implantation. As each new valve becomes available for such implantation we will continue to adhere to the continuity principle for calculating EOA, equating the stroke volume just below the prosthesis with the stroke volume through the prosthesis. However, this investigation by Shames et al highlights the necessity of familiarizing ourselves with each new prosthesis design so that, when necessary, we can appropriately alter the methods by which we acquire the measurements that we plug into the continuity equation.
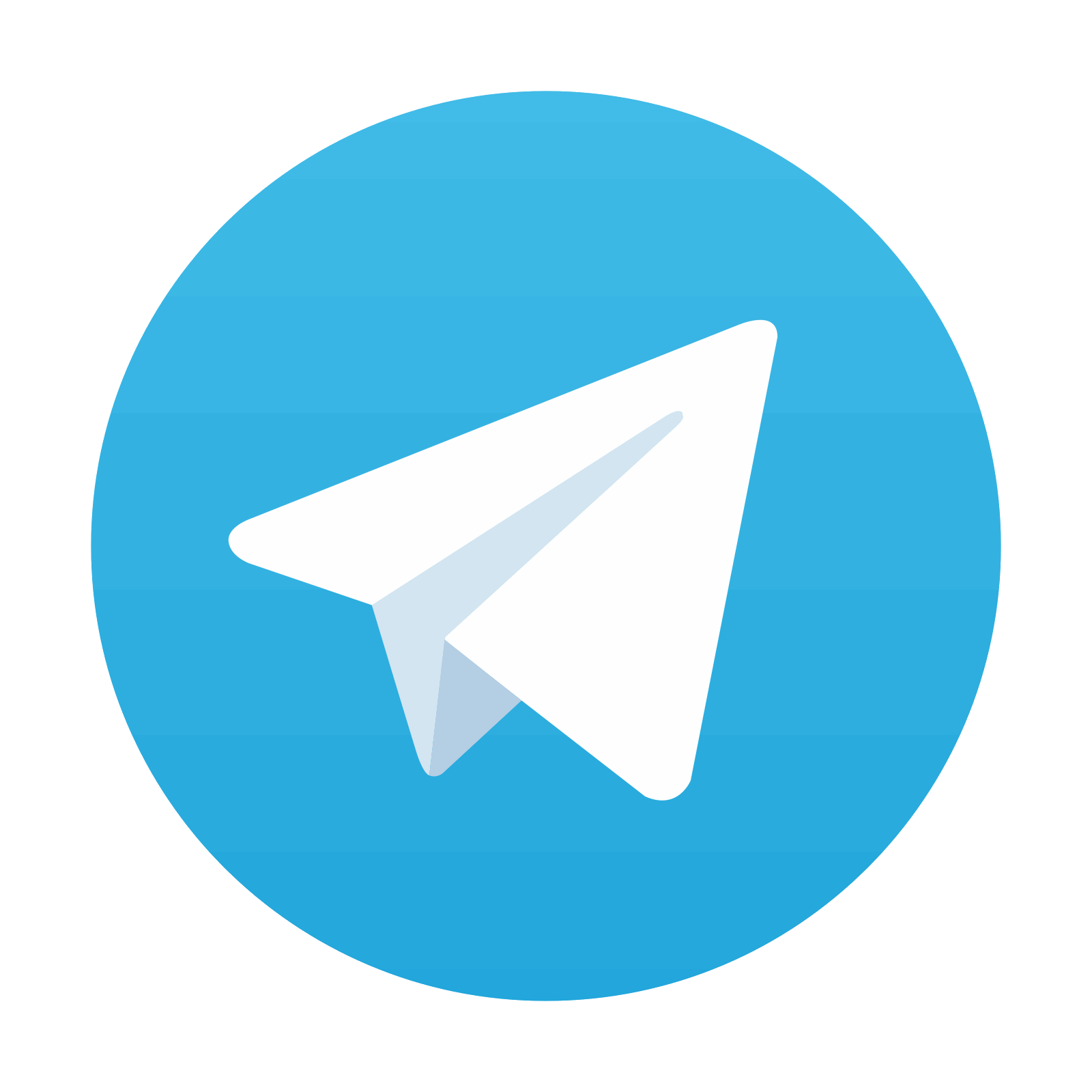
Stay updated, free articles. Join our Telegram channel

Full access? Get Clinical Tree
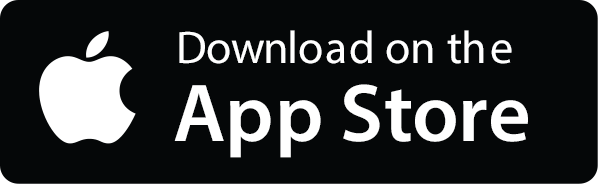
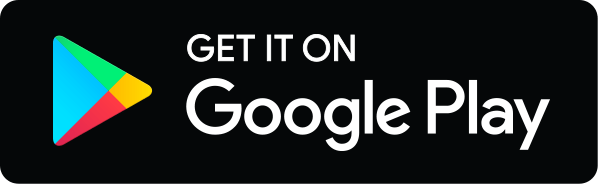