“If I could touch anything in the world right now, it would be your heart. I want to take that piece of you and keep it with me.”
The technology of three-dimensional (3D) printing, also referred to as “rapid prototyping,” “additive manufacturing,” and “stereolithography,” refers to the process of converting a 3D computerized model into an actual physical object. This technology became a reality in 1986 as a result of the innovative thinking of inventor Charles Hull (US patent no. 4575330). Currently the “entry-level” 3D printing machines on the market can lay down layers of material (plastic, metal, etc) onto a platform until a 3D model is complete. More advanced algorithms can use a laser as the power source to bind powdered materials, ranging from nylon to steel, to create a solid structure. Inkjet technology has also revolutionized 3D printing; materials are jetted through print heads while being cured by an ultraviolet light into a 3D object. Three-dimensional printing has been successfully used in medicine to make prosthetic limbs, custom hearing aids, and dental fixtures and is now being used to create more complex structures, such as cardiac models in patients with congenital heart disease. In this issue of JASE , Olivieri et al . describe their experience with creating 3D printed models from echocardiographic volumetric data sets in a series of nine patients, eight with ventricular septal defects (VSDs) and one with periprosthetic valvular leaks. In this editorial, we offer an overview of how technological explorations in 3D printing as carried out by Olivieri et al . will be fundamental to our understanding of the third dimension, elevating our impressions from textured flat-panel colored perspectives to real-time exploration of complex cardiac surface architecture, with the potential to guide personalized care of patients with structural heart disease.
Why is Three-Dimensional Printing Superior to Viewing Three-Dimensional Images on a Two-Dimensional Flat Screen?
Although multiple different 3D imaging modalities are currently available, one must question the effectiveness of most of these techniques, which project a 3D structure on a two-dimensional (2D) screen. Three-dimensional echocardiography, for example, allows us to create a volumetric data set and then manipulate and crop it from different perspectives to focus on the area of interest. Can one truly consider such a structure to be effectively communicating to the observer in three dimensions? Previous research into the ability of “mental rotation,” or visualizing a 3D structure and then being able to imagine it from different perspectives, tells us that the answer is probably no. It has been demonstrated that examining a 3D structure in an actual 3D format, which can be held, is more useful in relaying anatomy than simply trying to understand the structure from 2D images. In addition, individuals vary in the strength of their visuospatial skills, so that 2D images presented to a group of colleagues may not be interpreted in the same way by all. The use of 3D printed cardiac models eliminates this possibility and leaves no aspect of the spatial relationships to the imagination, which can be invaluable in patients with complex anatomy.
Three-Dimensional Printing of Cardiac Models: Will it Be for Everyone?
The majority of work pertaining to creating cardiac models using 3D printing involves computed tomographic (CT) or magnetic resonance imaging (MRI) source data sets. The ability to create models from echocardiographic data brings forth a new level of accessibility. The steps in creating a 3D virtual model for the purpose of rapid prototyping start with the acquisition of the 3D imaging data set. Ideally, there is good blood pool to myocardial contrast. Olivieri et al . optimized their 3D echocardiographic images before processing, which is a critical step in both decreasing the time required to create a 3D virtual model and increasing its quality. Segmentation, the process by which we designate the range of gray values that highlights our area of interest, becomes much faster with such a set of images. The designation of the anatomy of interest to be printed is relatively simple and can be easily done either by pediatric cardiologists or sonographers. Although echocardiographic data do not allow us to visualize or print the whole heart in three dimensions, focusing on one part simplifies the process and makes it less time consuming and labor intensive. Once the area of interest is highlighted, the image-processing software creates a 3D structure that can be saved and then printed on a 3D printer to create a physical cardiac replica. Such a work flow brings us closer to the reality of a scenario in which we acquire images in a patient with newly diagnosed congenital heart disease on one day, with the physical replica in hand in time for discussion at a surgical conference the next day.
There are certainly challenges in making this technology accessible to all programs that would like to use it. The ideal scenario would involve predefined protocols for image acquisition, automated postprocessing, and subsequent creation of a 3D physical model on a cost- and time-efficient printer. Currently and unfortunately, there are no published recommendations on parameters that should be used during 3D image acquisition, for any modality. In addition, thin mobile structures, such as valves and the subvalvar apparatus, continue to be challenging to recreate. For patients with congenital heart disease, the automation of the segmentation process promises to be a challenge. With such a wide variation in cardiac anatomy for each lesion, it seems almost impossible for software to identify relevant structures for surgical planning, assign appropriate values for segmentation, and crop the virtual 3D model in a manner to best display the anatomy of interest. In their “Conclusions” section, Olivieri et al . refer to the technology used to create these models as “highly accessible.” It is difficult at this stage for this technology to be considered readily available. The cost of both the image postprocessing software and the 3D printer put the process even further from the reach of every program that may wish to use it. In addition, it remains unclear which patient populations will benefit most from these models. Although the report by Olivieri et al . includes mainly cases of patients with VSDs, it may be more intuitive to study patients with more complex intracardiac anatomy, in whom the models would add more spatial information. Nevertheless, the report by Olivieri et al . should be considered symbolic of the progress and burgeoning interest in creating 3D cardiac models from routine echocardiographic data sets.
Touching Three Dimensions to Explore Structural Heart Disease
The utility of this technology in multiple areas of medicine is increasingly being noted in the literature. The prospects for applications in transcatheter interventional procedures and presurgical planning for complex cardiac cases are particularly exciting ( Table 1 ). One of the earliest works, by Binder et al ., used a multiplane transesophageal echocardiographic probe to recreate 3D echocardiographic data sets at a time when the process was far from automated. Subsequent work by Gilon et al ., also using echocardiographic data sets and stereolithographic technology, demonstrated that the 3D aortic valve shape in aortic stenosis contributes to the lesions’ hemodynamic effects in addition to the limiting valve orifice.
Study | Purpose | Subjects | Principal findings |
---|---|---|---|
Binder et al . | To assess feasibility, accuracy, and clinical potential of creating hard sterolithographic models from echocardiographic data | Patients with mitral valve disease (stenosis, prolapse, annular dilation, leaflet restriction) and phantoms to test accuracy | Stereolithographic modeling of echocardiographic images is feasible and provides tangible polyacrylic models that are true to scale, shape, and volume. |
Gilon et al . | To study the hemodynamic impact of the valve geometry in aortic stenosis using 3D sterolithographic physical models | Patients with aortic stenosis | Three-dimensional valve shape is an important determinant of pressure loss in patients with aortic stenosis, with smaller effective areas and higher pressure gradients for flatter valves. |
Sodian et al . | To explore the use of RP for surgical planning and intraoperative orientation | Patients requiring resternotomy for aortic valve replacement with previous CABG | These replicas are helpful in choosing treatment strategies and for intraoperative orientation in patients with previous CABG. |
Sodian et al . | To demonstrate the use of a 3D printed aortic arch model for planning and simulation of occlusion of a pseudoaneurysm | Patient with prior ascending aorta and arch replacement with an aortic arch pseudoaneurysm | The printed model allowed fabrication of an occluder device in a patient with a pseudoaneurysm. |
Schmauss et al . | To evaluate the potential effects of 3D models for surgical planning and simulation of transcatheter intervention | Patient with aortic stenosis and porcelain aorta | The model was useful to demonstrate to the authors that a transcatheter valve should be positioned deep into the aortic annulus. |
Schmauss et al . | To explore using RP for surgical planning, decision making and intraoperative orientation | Patient with a right ventricular primary cardiac tumor | The models allowed identification of the position and expansion of the tumor and to plan surgical intervention. |
In patients with complex congenital heart disease and complex intracardiac relationships ( Table 2 ), 3D printing removes the potential for misinterpretation that may occur between the presentation of images in two dimensions and the mental reconstruction that is undertaken to understand the relationships in three dimensions. For example, 3D printing has been used to demonstrate the location of major aortopulmonary collateral arteries in patients with tetralogy of Fallot and pulmonary atresia and to guide sternotomy in adults who have undergone coronary bypass surgery and subsequently required aortic valve replacement at a later date. These two scenarios, in addition to the patient cases presented by Olivieri et al ., demonstrate the use of 3D models for the purpose of directly visualizing anatomic relationships in a physical replica.
Study | Purpose | Subjects | Principal findings |
---|---|---|---|
Ngan et al . | To assess utility and accuracy of solid anatomic models for surgical planning | Patients with pulmonary atresia, VSD, and MAPCAs | Three-dimensional printed anatomic models of the pulmonary vasculature from CTA data accurately represented MAPCAs and were found by the surgeons to be very useful in visualizing the vascular anatomy. |
Noecker et al . | To demonstrate the utility of 3D cardiac modeling for medical device development | Pediatric patients with and without CHD | Three-dimensional models of pediatric cardiac and chest anatomy provided enhanced understanding and tactile representation of complex anatomy, which was found to be particularly useful during the development and placement of medical devices. |
Sodian et al . | To explore the impact of using RP techniques for surgical planning and intraoperative orientation | Patients with complex congenital heart disease | Stereolithographic replicas are helpful for choosing treatment strategies, surgical planning of corrections, and intraoperative orientation, as demonstrated in a patient with aberrant retroesophageal left subclavian artery and right aortic arch and a patient with a VSD. |
Schievano et al . | To determine if MRI data could be used to create rigid models which accurately represent the right ventricular outflow tract and pulmonary trunk for PPVI | Patients requiring PPVI | Using 3D RP models resulted in more accurate selection of patients for PPVI than did using magnetic resonance images. |
Greil et al . | To demonstrate the creation of 3D physical models using high-resolution MDCT and MRI | Patients with congenital heart disease including tetralogy of Fallot, D-TGA, and DORV | High-resolution MDCT or MRI 3D data sets can be accurately reconstructed using laser sinter technique. Teaching, research, and preoperative planning may be facilitated in the future using this technique. |
Jacobs et al . | To create anatomically correct RP models to facilitate planning and execution of the surgery | Patients with complex congenital heart disease and altered geometry of the atria and ventricles | The use of the 3D rapid prototyping model during resection of ventricular aneurysm and malignant cardiac tumors may facilitate the surgical procedure because of better planning and improved orientation. |
Mottl-Link et al . | To improve spatial imagination for planning of surgical intervention | Patient with L-TGA, pulmonary atresia, atrial and VSDs, and dextrocardia | A 3D printed model in a patient with transposition of the great arteries, pulmonary atresia, atrial septal defect, and VSD were helpful in preoperative in surgical planning. |
Vranicar et al . | To assess accuracy of 3D stereolithographic models created in patients with great vessels anomalies including coarctation of the aorta and vascular rings | Patients with anomalies of the great vessels | Three-dimensional printed models for patients with aortic coarctation and vascular rings were useful in planning transcatheter and surgical interventions. |
Sodian et al . | To demonstrate the utility of 3D physical replicas in presurgical planning | Single-ventricle patients with failing ventricles requiring transplantation | Three-dimensional printed models were useful for perioperative planning and intraoperative visualization in patients with failing single ventricles undergoing cardiac transplantation. |
Shiraishi et al . | To assess if a stereolithographic modelling technique can be applied for anatomical diagnosis and surgical simulation | Patients with complex congenital heart disease | Rubber-like urethane cardiac biomodels allowed surgeons to practice and actually suture and cut the flexible material. |
Schmauss et al . | To present a single-center experience with a wide variety of cases using this technology in complex pediatric and adult cardiac cases | Patients with congenital heart disease and adults with structural heart disease | This group demonstrated its center’s experience and the feasibility of creating 3D models in a series of patients with congenital and structural heart defects in preparation for either surgical or transcatheter interventions. |
Ryan et al . | To demonstrate a new approach to identify location of MAPCAs using RP that may preclude cardiac catheterization | Patient with tetralogy of Fallot, pulmonary atresia, and MAPCAs | Use of a 3D printed model allowed preoperative planning in a patient with tetralogy of Fallot, pulmonary atresia, and MAPCAs without cardiac catheterization. |
Olivieri et al . | To demonstrate utility of a 3D printed model to assist in presurgical and interventional planning | Patient with D-TGA who had undergone a Mustard repair | The insight provided by 3D modeling may help increase procedural efficiency, decrease radiation exposure, and mitigate procedural complications. |
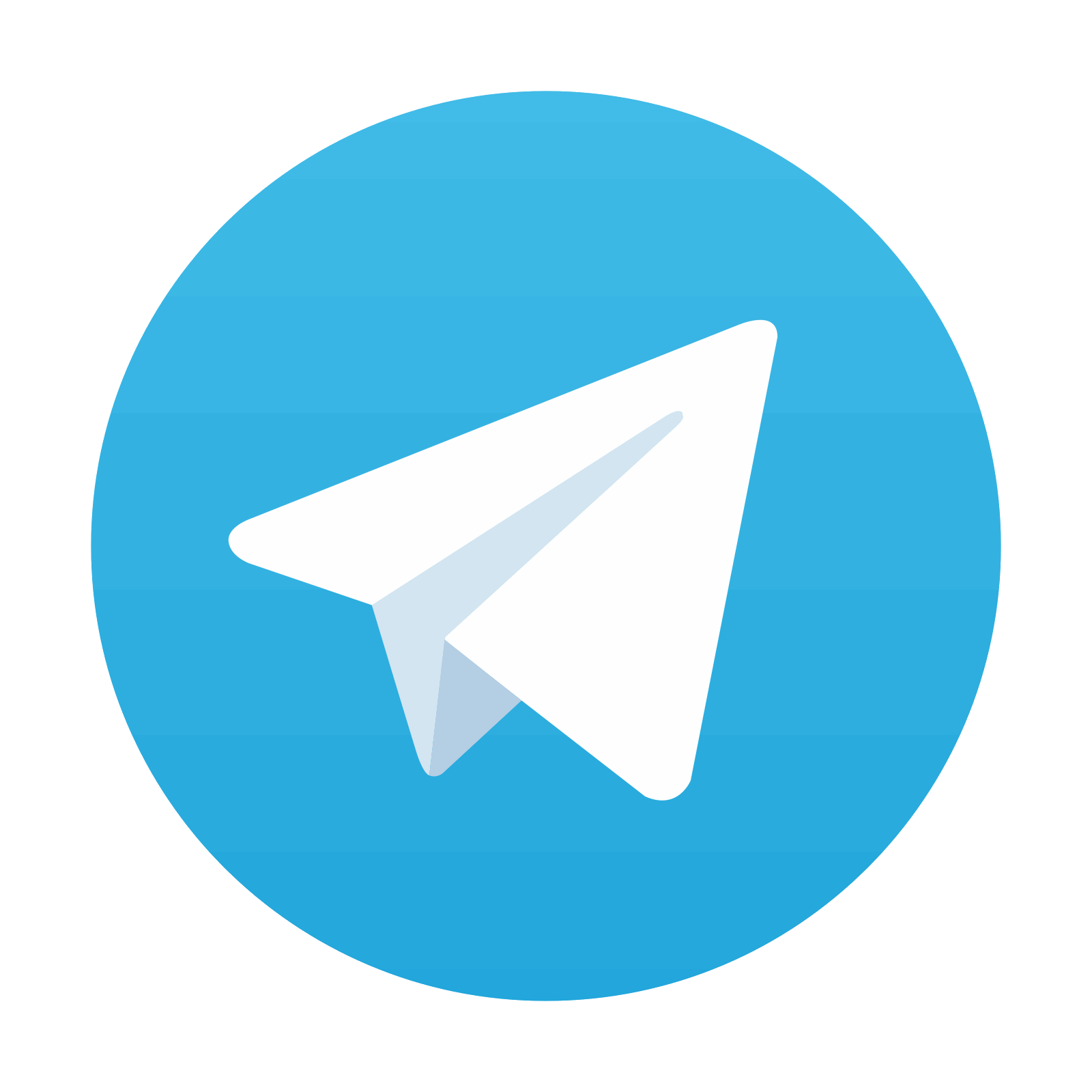
Stay updated, free articles. Join our Telegram channel

Full access? Get Clinical Tree
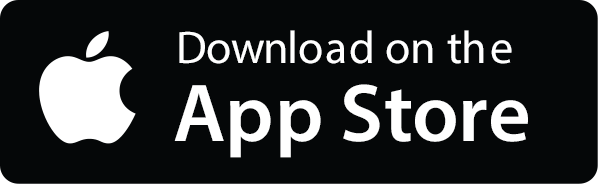
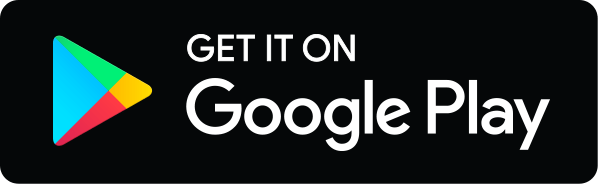
