Echocardiography is a widely used cardiac imaging modality that is often the first-choice test for assessing cardiac structure and function. It provides a detailed structural assessment of the myocardium, valves, pericardium, and portions of the surrounding vasculature, which includes the thoracic aorta, pulmonary artery, pulmonary veins, inferior vena cava, and superior vena cava. With real-time evaluation, echocardiography provides an assessment of the systolic and diastolic function of the myocardium, as well as a measurement of intracardiac hemodynamics and the severity of valvular disease. Compared to other cardiac imaging modalities, echocardiography is relatively less expensive, does not expose the patient to ionizing radiation, and is generally well tolerated by the patient.
The two primary echocardiographic techniques include transthoracic echocardiography (TTE) and transesophageal echocardiography (TEE). With TTE, the ultrasound probe is placed on the patient’s chest and several views of the heart are obtained from various angles. In TEE, a flexible ultrasound transducer is introduced into the esophagus and can be rotated or flexed at varying levels in the esophagus to allow for imaging of the cardiac structures. Compared to TTE, TEE has better image quality for posterior cardiac structures due to the closer proximity of the transducer to the heart. TEE is especially useful in the evaluation of endocarditis, valvular dysfunction, and interatrial septal defects.1 Since its introduction to clinical medicine, echocardiograpjy has undergone significant technological advances. As a result, echocardiography is an essential imaging tool in clinical medicine.
Echocardiographic images are obtained with an ultrasound transducer which emits sound waves that are transmitted through the internal structures and then reflected back to the transducer to create an image.2 In standard TTE, the available modalities include M mode, two-dimensional (2D), and three-dimensional (3D). M mode is the most basic method which evaluates cardiac structures in a one-dimensional (1D) view along a single-scan line, which places depth on the y-axis and time on the x-axis. This is ideal for making fine linear measurements. M mode also has excellent temporal and spatial resolution and is thus useful to assess motion and timing.2 2D is the most utilized echocardiographic modality, and produces cross-sectional images of the heart by emitting numerous signals that are repeatedly generated to create moving images in real time. The most common standard 2D views are the parasternal long-axis, parasternal short-axis, and the apical and subcostal views (Fig. 27-1). Off-axis imaging may then be necessary to evaluate more complex structures. Newer machines are also able to generate 3D images of the cardiac structures through the use of matrix-array transducers to provide detailed structural assessments.3 Compared to 2D echocardiography, 3D imaging provides more accurate quantification of ventricular volumes (Fig. 27-2), generates unique views of valve structures (Fig. 27-3), and demonstrates improved visualization of spatial relationships between cardiac structures.3
Figure 27-1
Transthoracic echocardiographic images from multiple views. Panels A and B are parasternal long- and short-axis views, respectively. They show the LV in long axis (A) and short axis (B). Panel C is a four-chamber view that is typically obtained from the apex. Panel D is a subcostal view, and similarly shows a four-chamber view of the cardiac chambers. LV, left ventricle; LA, left atrium; RV, right ventricle; RA, right atrium.

Figure 27-3
Three-dimensional transesophageal echocardiography. Panel A shows an enface view of the aortic valve; the three aortic leaflets are shown open in systole. Panel B shows a three-dimensional view of the mitral valve from the atrial side; the anterior and posterior mitral leaflets are visible. NCC, noncoronary cusp; LCC, left coronary cusp; RCC, right coronary cusp.

Another echocardiographic imaging technique that helps to improve image resolution is tissue harmonic imaging. When an ultrasound wave is transmitted through tissues, the signal becomes distorted and generates additional frequencies. These are harmonics of the fundamental frequency, and the energy of these frequencies increases with the distance of propagation.2 In echocardiography, a great deal of the image artifacts is due to scattering at the chest wall, where there is little harmonic frequency energy. Therefore, if the imaging is limited to only the harmonic range, a significant amount of the artifact is eliminated and a higher-quality image is obtained.4 This technique has the greatest benefit in patients who have technically limited studies with poor image quality.4
One of the primary reasons for ordering an echocardiogram is to evaluate the left ventricular systolic function. In 2D echocardiography, the most common method for determining ejection fraction is the use of the biplane method of disks summation (modified Simpson’s rule) (Fig. 27-4).5 In this method, the reader manually traces the endocardial border, with the left ventricular volume calculated as the summation of a stack of elliptical disks filling the space. This approach can be prone to error in cases of poor image quality where the endocardial borders are difficult to delineate, and can become less accurate if foreshortening of the ventricle occurs in the apical views. By making the borders easier to identify, the use of an echo contrast agent improves the accuracy and reproducibility of 2D echocardiography.6 Echocardiographic contrast reduces both inter- and intraobserver variability,6 which has traditionally been one of the disadvantages of echocardiography when compared to other imaging modalities (Fig. 27-5).
Figure 27-4
Two-dimensional volume measurement of the left ventricle using the biplane method of discs (modified Simpson’s rule). The endocardial borders are traced in the apical four-chamber (panels A and B) and apical two-chamber (panels C and D) views at end diastole and end systole, in order to calculate the ejection fraction. The papillary muscles should be excluded from the cavity in the tracing.

Figure 27-5
Normal dobutamine stress echocardiography showing the LV in the four-chamber view. Contrast was administered to improve endocardial border visualization. Normal response is seen with increasing doses of dobutamine; wall-motion contractility increases globally, and the LV decreases in size. Panel A, Rest stage; Panel B, Low dose dobutamine; Panel C, Peak dose dobutamine; Panel D, Recovery stage. LV, left ventricle.

Ultrasound images can also be used to assess cardiac blood flow and myocardial movement through the use of Doppler. This is based on the principle that the frequency of the reflected signal emitted from a moving object will be perceived differently depending on whether the object is moving toward or away from the observer.2,7 In echocardiography, the moving objects being tracked are typically red blood cells. The two primary types of spectral Doppler imaging are pulsed-wave (PW) and continuous-wave (CW) Doppler (Fig. 27-6).7 In PW Doppler, discrete intermittent bursts of ultrasound are reflected off the moving red blood cells at a specific depth within the heart. The common applications for this modality include the measurement of cardiac output, assessment of regurgitant volumes, and the evaluation of diastolic function.8 This technique is limited by its inability to accurately detect signals at higher velocities. With CW Doppler on the other hand, continuous ultrasound is emitted from the transducer and continuously analyzed. This allows for the assessment of higher velocities, though at the expense of decreased precision in localization. CW Doppler is best suited for evaluation of high-velocity flow across stenotic or regurgitant valvular orifices.8 The combination of PW and CW can be used to calculate intracardiac and valvular flows and gradients that allow for a comprehensive hemodynamic assessment of the heart. Color-flow Doppler is an additional technique that is based on PW Doppler, and involves color-coding of flow based on velocity and direction. Red represents blood flow toward the transducer, while blue represents blood flowing away. High velocity and turbulent blood flow show a mosaic color pattern, making color-flow Doppler ideal for evaluating flow in abnormal valves (Fig. 27-7).
Figure 27-7
Transthoracic echocardiogram of a patient with ischemic cardiomyopathy and a restricted posterior mitral leaflet. Color Doppler assessment of the mitral valve in multiple views shows severe mitral regurgitation (red arrows). Panel A, parasternal long-axis view; panel B, four-chamber view; panel C, two-chamber view; panel D, three-chamber view. LV, left ventricle; LA, left atrium.

Another application for Doppler echocardiography is tissue Doppler imaging (TDI). In contrast to the previously described traditional Doppler techniques that focus on the high-frequency motion of red blood cells, tissue Doppler assesses the higher-amplitude and lower-velocity signals generated from myocardial movement.9 TDI is particularly useful in the assessment of diastolic function, which develops in the setting of impaired myocardial relaxation and increased LV chamber stiffness (Fig. 27-8).10
During the normal cardiac cycle the apex remains relatively fixed, with the mitral annulus moving toward the apex in systole and away in diastole. The shortening of the left ventricle during systole can be demonstrated by the descent of the annulus, and thus TDI of mitral annular motion provides a good representation of left ventricular systolic function.11 TDI waveforms reflect the systolic velocity (S’), early diastolic velocity (e’), and late diastolic velocity (a’) of the mitral annulus. Combining these measurements with the mitral inflow velocities in early diastole (E) and late diastole (A) provides for an assessment of diastolic function. Specifically, peak E and A velocities, E/A ratio, e’ velocity, and E/e’ ratio are all important measurements in the determination of diastolic dysfunction. Other echocardiographic parameters, including left atrial size, pulmonary vein systolic to diastolic ratio, and peak velocity of tricuspid regurgitation (TR) signal, can also be integrated to help determine the degree of diastolic impairment (Fig. 27-9).10 One of the main weaknesses of TDI is the inability of the technique to differentiate myocardium that is actively contracting from one that is dyskinetic or akinetic, but appears to be contracting because it is tethered to a moving segment. Myocardial deformation imaging, on the other hand, is a newer technique that does not have this limitation. Deformation indices such as strain and strain rate provide quantitative, and thus more objective, measures of regional myocardial performance.
Figure 27-9
2D, Doppler, and tissue Doppler transthoracic echocardiographic views used in combination, for diastolic evaluation. Panel A shows a dilated left atrium. Mitral inflow evaluation by pulsed Doppler (panel B) shows an E/A >2. Panel C shows depressed septal tissue Doppler velocity, and Panel D shows a pulmonary vein inflow pattern suggestive of advanced diastolic disease (diastolic predominance and prominent atrial flow reversal). The E/’e = 27.5. In combination, this is diagnostic of restrictive diastolic dysfunction (grade 3) with elevated filling pressures. LA, left atrium; vol, volume.

Simply put, strain represents the percentage change in the length of a myocardial fiber during contraction relative to its original dimension, and strain rate is its temporal derivative (1/second). Thus, these strain-derived parameters allow assessment of the extent of myocardial thickening in addition to its timing. Because these methods evaluate each myocardial segment individually, they are relatively less susceptible to tethering and translational motion.
Strain and strain rate may be derived from TDI, which requires the direction of wall motion to be along the scan line of the ultrasound, and therefore is angle-dependent.12 Alternatively, speckle-tracking echocardiography, which tracks the unique speckle patterns of the myocardium, is not dependent on the Doppler angle. This allows for strain analysis in multiple different planes, including longitudinal, circumferential, radial, and rotational strain.12,13
In addition to these advances in 2D echocardiography, 3D imaging also allows for a high-quality assessment of systolic function. 3D echocardiography does not require geometric assumptions about left ventricular shape, and therefore provides a more accurate measure of left ventricular volumes compared to standard 2D imaging, especially in patients with asymmetric ventricles or with wall-motion abnormalities after a myocardial infarction (MI).14 3D echocardiography has been shown to provide comparable volume measurements to radionuclide angiography,15 which has long been one of the preferred noninvasive methods for estimating left ventricular ejection fraction. Similarly, when compared to standard 2D imaging, 3D echocardiography demonstrates a greater correlation to the volume measurements obtained with cardiac magnetic resonance imaging (CMR),16 which is generally considered to be the gold standard for measuring ejection fraction. With 3D echocardiography becoming more widely available, it appears to be a reasonable alternative to CMR imaging for accurate and noninvasive assessment of left ventricular systolic function in patients with optimal image quality.
Stress echocardiography (SE) is primarily used to evaluate for myocardial ischemia through the assessment of regional wall-motion abnormalities. It can also be used as a prognostic tool in patients with known CAD, to predict outcomes after coronary revascularization, and to assess the severity of valvular heart disease (VHD). While stress testing has traditionally involved patients undergoing aerobic exercise, pharmacologic stress agents can also elicit a similar cardiovascular response in patients unable to participate in a usual exercise protocol.
In the normal response to either a physical or inotropic stress, the left ventricle demonstrates increased endocardial excursion and myocardial thickening, which subsequently leads to an increase in ejection fraction and reduced cavity size at end systole. As a result, the myocardial oxygen demand and uptake increases with stress. This uptake is influenced by several factors, including heart rate, contractility, and left ventricular wall stress. Coronary blood flow increases in a linear fashion alongside myocardial oxygen uptake, up to a fivefold higher flow than at baseline.17 In patients who have obstructive CAD, however, coronary blood flow is unable to satisfy the metabolic demands of the myocardium during stress. This results in myocardial ischemia from a regional mismatch between the myocardial oxygen demand and the reduced supply from arterial stenosis. In SE, this is generally represented by new or worsening wall-motion abnormalities, as well as increasing left ventricular cavity size with stress and decreased ejection fraction.
Prior to initiating the stress portion of the examination, baseline echocardiographic images are obtained with the patient at rest. Standard M-mode and 2D imaging is performed to assess all segments of the left ventricle. An overall assessment of other cardiac structures is also performed. Evaluation of the left ventricle using harmonic imaging can improve endocardial border definition, which is critical for the assessment of wall motion.18 Typically the parasternal long- and short-axis views are obtained, as well as at least two and preferably three apical views. The baseline images should be evaluated for any resting abnormalities that may be indicative of a prior infarct. Other cardiac structures, such as the valves, pericardium, and aortic root, can also be evaluated for any pathology or contraindications to stress testing.
To assess for myocardial ischemia with echocardiographic stress testing, several modalities are available, including exercise, pharmacologic, and pacing. When patients are physically able to exercise, this is the preferred method for stress testing as it provides additional information regarding exercise capacity, which has been shown to be a predictor of mortality.19 With standard protocols, baseline echo images are obtained at rest prior to the patient exercising, and then again at peak exercise or immediately after peak exercise.
Exercise SE is most commonly performed using a treadmill and less often a supine bicycle. In both modalities, the patient gradually increases exercise intensity throughout the duration of test. The Bruce protocol, which is the most common treadmill protocol in use, involves incremental increases in treadmill speed and grade in 3-minute intervals. Both bicycle and treadmill exercises allow for the determination of exercise capacity and peak metabolic equivalents (METs) achieved. In treadmill SE, the patient is moved quickly from the treadmill to the imaging bed after the termination of exercise to obtain post-exercise images (Fig. 27-10). In order to obtain the most accurate evaluation for ischemia, post-exercise images need to be captured within the first minute of cessation of exercise. Even so, during this time period, the transient echocardiographic findings indicative of ischemia may already be resolving, which can reduce the sensitivity of the test. With supine bicycle testing, echo images are obtained during exercise, which allows capture of exercise images at a higher heart rate. When comparing supine bicycle echocardiography to traditional treadmill stress testing with the capture of immediate post-exercise images, supine bicycle stress testing has a higher sensitivity for detecting ischemia.20
Figure 27-10
A normal exercise-stress echocardiographic test is shown. Baseline echocardiographic views are obtained at rest showing normal wall motion. This is followed by obtaining peak images after maximal exercise on a treadmill. Peak images show normal response with hypercontractile wall motion and decreased left ventricular cavity size. Panel A, three-chamber view; panel B, parasternal long-axis view; panel C, four-chamber view; panel D, two-chamber view.

For patients who are unable to exercise, pharmacologic stress testing is an available alternative. The most commonly used agent is dobutamine, with graded infusions uptitrated in 3-minute increments starting from 5 or 10 μg/kg/min up to a peak dose of 40 μg/kg/min, as discussed in greater detail in Chapter 8 (Fig. 27-11).21 This increases the myocardial oxygen consumption by increasing inotropic state, heart rate, and blood pressure.22 The goal is to increase the heart rate to at least 85% of the age-predicted maximum, which is the target of both the exercise and pharmacologic protocols.
Figure 27-11
A normal dobutamine stress echocardiographic test is shown. All images are at end systole. As the dobutamine is infused, the myocardial contractility increases and the left ventricular cavity size decreases until the target heart rate is achieved. Recovery images are obtained after the infusion is stopped and the heart rate recovers back to baseline. Panel A, four-chamber view; panel B, two-chamber view; panel C, short-axis view; panels D, parasternal long-axis view.
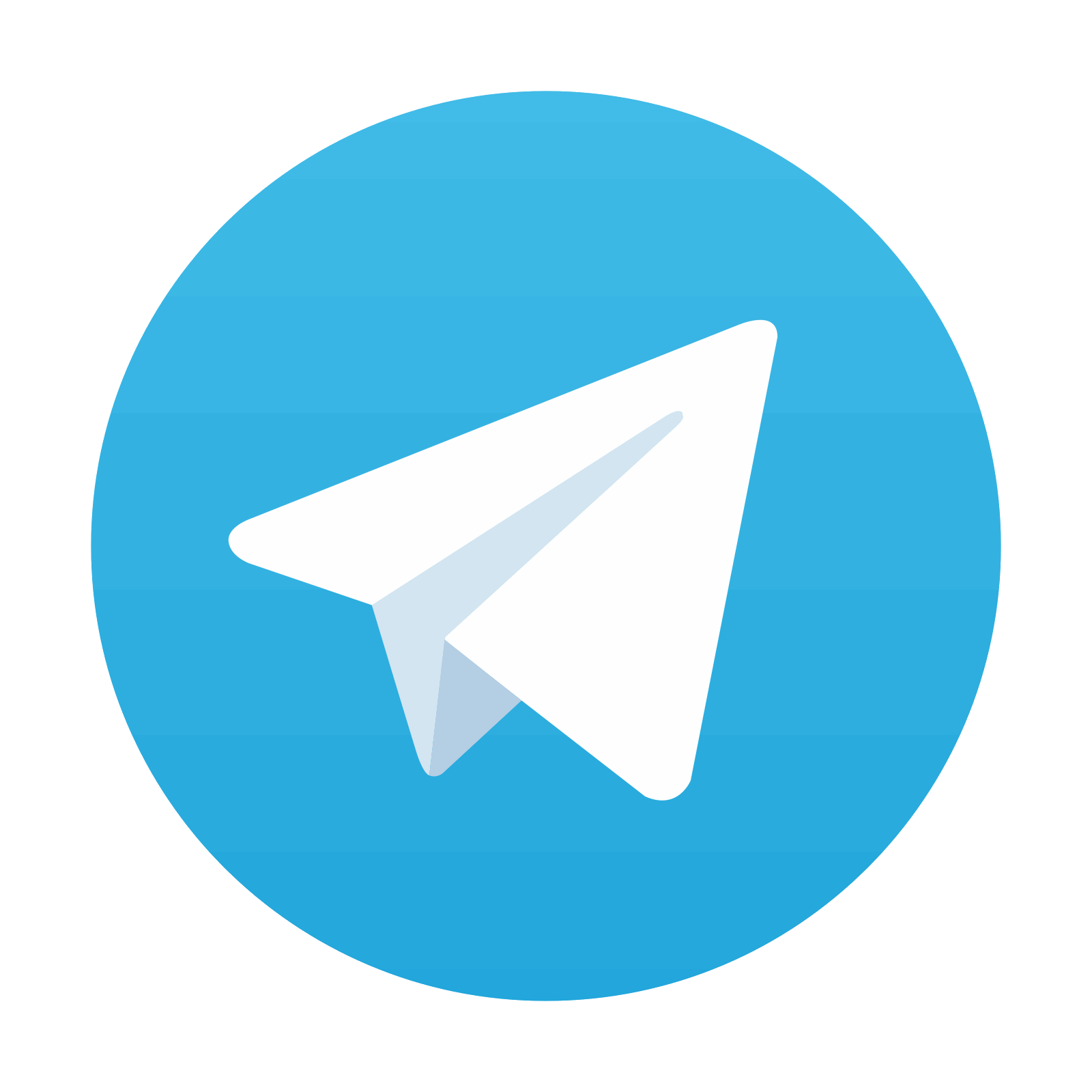
Stay updated, free articles. Join our Telegram channel

Full access? Get Clinical Tree
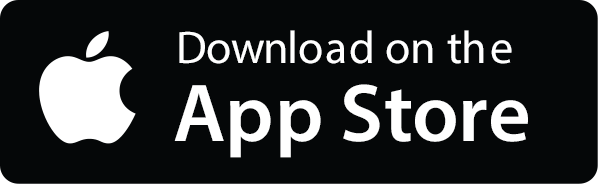
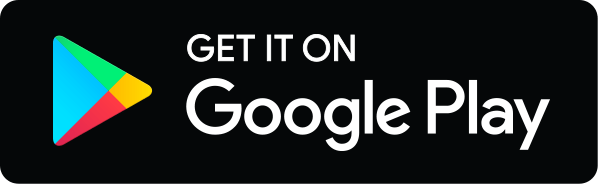
