Arterial distribution (Figures 32.2, 32.13). Note that LAD supplies the anterior two-thirds of the septum, while RCA supplies the inferior one-third of the septum. A normal wall motion is characterized by an appropriate inward endocardial movement but also appropriate myocardial thickening. A segment can be hypokinetic, akinetic, or dyskinetic. Dyskinesis is outward movement of a myocardial wall during systole, when the remaining walls have an inward movement. Dyskinesis is therefore myocardial outpouching in systole, whereas aneurysm is myocardial outpouching in both systole and diastole (see Chapter 2). Figure 32.1(a) Frontal view showing how the parasternal short-axis views cut through the heart (cross-sectional cuts). The first structure encountered is the right ventricle, which is actually the anterior ventricle; the base of the LV and the mitral valve are encountered more posteriorly. Angling the probe more superiorly allows visualization of the aortic valve, the RA and LA, as well as the RV inflow and outflow (aortic short-axis view, dotted lines). Angling the probe more inferiorly allows visualization of the LV body and apex (dashed lines). (b) Parasternal long-axis cuts. The first structure encountered is the RV (more specifically, RVOT), which is actually the anterior ventricle. The LV, LA, and aortic base are encountered more posteriorly, and the interventricular septum is seen in between. Angling the probe more inferiorly (dashed lines) allows visualization of the inferior RV wall and the tricuspid valve, as well as the RA (RV inflow view). Angling the probe more superiorly allows visualization of the RVOT and pulmonic valve (RV outflow view). (c) Apical four-chamber cut. The first structures encountered are the apices of the LV and RV, while the atria are seen in the back. Superior angulation allows visualization of the aortic valve (five-chamber). A 90° rotation would focus on the LV–LA (two-chamber view). Further rotation opens the aortic valve (three-chamber view). Figure 32.2 (a) Diagram of the parasternal short-axis view and various LV segments, with their corresponding arterial supply. (b) Orientation of various echo cuts. Figure 32.3 (a) Parasternal short-axis view at the level of the mitral valve. Each leaflet has three cusps (A1–A3 for the anterior leaflet, and P1–P3 for the posterior leaflet). A3 and P3 are medial, towards the RV; A1 and P1 are lateral. The orientations of various echo cuts through the mitral plane are shown. (b) Echocardiogram showing the parasternal short-axis view (anterior and posterior leaflets, circles). The triangular-shaped RV is partially seen (arrow). Figure 32.4 Diagram of the parasternal short-axis view at the level of the aortic valve (cut closer to the base than Figure 32.3, across the aortic valve, the LA/RA, and RVOT). The non-coronary or posterior cusp is always the cusp looking towards the interatrial septum. LC, left coronary cusp; NC, non-coronary cusp; RC, right coronary cusp. Reproduced with permission from Hanna EB, Quintal R, Jain N. Cardiology: Handbook for Clinicians. Arlington, VA: Scrub Hill Press, 2009. Figure 32.5 Parasternal short-axis view as described in Figure 32.4. A bicuspid aortic valve may be diagnosed on this view, by assessing how the valve opens in systole: a bicuspid valve opens in an oval rather than a “Y” fashion. In diastole, a bicuspid valve may look tricuspid because a fused raphe may be seen between the fused leaflets. In severe AS, the aortic valve barely opens in this view; planimetry of the aortic valve area may be performed (especially by TEE). Rarely, however, the aortic valve may falsely look open if the cut is a bit below or above the valve level, masking severe AS. The left image shows backward (= blue) blood flow across the tricuspid valve (= TR). Reproduced with permission from Hanna EB, Quintal R, Jain N. Cardiology: Handbook for Clinicians. Arlington, VA: Scrub Hill Press, 2009. Figure 32.6 (a) Diagram of the parasternal long-axis view. Concerning the aortic valve cusps: the lower one, close to the LA, is the non-coronary cusp (NC); the upper one, close to RVOT, is the right coronary cusp (RC). The mitral leaflet close to the aorta is the anterior mitral leaflet (A2 cusp); the mitral leaflet close to the posterior wall is the posterior leaflet (P2 cusp). (b) Echocardiogram showing the parasternal long-axis view. Note the coronary sinus in the AV groove (arrow), and note the descending aorta behind it (x). The coronary sinus is anterior to the bright pericardium (line), whereas the descending aorta is posterior to it and is more rigid. From top to bottom, the following structures are seen around the pericardium: coronary sinus → pericardium/pericardial effusion → aorta → pleural effusion. The aorta separates pericardial from pleural effusion. The coronary sinus goes on to drain in the RA. Coronary sinus may enlarge in case of RA dilatation/pulmonary hypertension or persistent left SVC; in the latter case, coronary sinus is severely enlarged > 1 cm. Figure 32.7 (a) Parasternal long-axis view. Measurements are obtained from top to bottom, between delineated points, at an oblique line crossing the mitral leaflet tips and orthogonal to the axis of the LV (line). (b) Aortic measurements. The annulus is a stable structure that is part of the ventricle/outflow tract and does not usually dilate. The aortic diameter at the level of the sinuses of Valsalva (i.e., the aortic dilatations that suspend the aortic cusps) is normally up to 3.7 cm, while the diameter of the proximal ascending aorta and the sinotubular junction (junction of the ascending aorta with the sinuses of Valsalva) is normally up to 3.2 cm. Aortic dilatation may occur at the level of the ascending aorta and sinotubular junction (e.g., HTN), or may involve the sinuses of Valsalva in addition to the ascending aorta (bicuspid aortic valve, Marfan disease). The normal diameter at the sinuses is affected by age and body surface area and should generally be < 1.9 cm/m2 (lower cutoff in younger patients). Reproduced with permission from Hanna EB, Quintal R, Jain N. Cardiology: Handbook for Clinicians. Arlington, VA: Scrub Hill Press, 2009. Figure 32.8 (a) Parasternal RV inflow view, which is obtained by angling the probe inferiorly from the parasternal long-axis view. This view allows visualization of the RV (horizontal bar) and RA (vertical bar). The IVC entrance into the RA is seen, as well as the coronary sinus (CS) beneath it. A rigid Eustachian valve or a filamentous Chiari network separates the two (Eustachian valve is seen here). The RV walls are the anterior (a) and inferior (b) walls, and the two tricuspid leaflets are the anterior (1) and posterior leaflets (2). (b) The posterior leaflet is the smallest leaflet, and for that reason only one view shows it, the RV inflow view (in some patients, it is the aortic short-axis view). All other tricuspid valve views show the anterior and septal leaflets (four-chamber, subcostal, and aortic level short-axis views). Figure 32.9 (a) Diagram and (b) echocardiogram of the apical four-chamber view. Aorta and LVOT can be opened up by tilting the transducer up, creating the five-chamber view. The anterior leaflet is seen medially (1), the posterior leaflet is seen laterally (2), the anterior papillary muscle is lateral (1’), the posterior papillary muscle is medial (2’). Note that the valvular leaflets and their respective papillary muscles crisscross in this view. The tips of the papillary muscles define the separation between basal LV and mid-LV; the distal part of the papillary muscles separates mid-LV from apex. Sometimes, LV false tendon, a fibrous/fibromuscular band, is seen extending distally from the septum to the lateral LV wall. RV has coarse apical trabeculations (more coarse than LV); it has ≥3 small papillary muscles. A muscular band where the right bundle is embedded, the moderator band, may sometimes be seen spanning from the septal to the lateral RV wall, similar to the false tendon on the left. The tricuspid valve is more apical than the mitral valve. When both valves are at the same level, endocardial cushion defect is suspected. Advanced note: The anterior cusp (1) is A2 ± A3, the posterior cusp (2) is P1, the most lateral one; see Figure 32.3 to understand how the cusps are cut and the relation between leaflets and papillary muscles. (c) Apical four-chamber view in dilated cardiomyopathy: LV changes from elliptical to spherical. Note the false tendon and the moderator band, and note the descending aorta behind the LA. Figure 32.10 (a) Diagram and (b) echocardiogram of the apical two-chamber view; and (c) echocardiogram of the apical three-chamber view. By opening the aorta with a counterclockwise rotation, the apical two-chamber view becomes the apical three-chamber view, where the anterior and inferior walls are replaced by the septal and posterior walls, respectively. The apical three-chamber view, also called apical long-axis view, is similar to the parasternal long-axis view, except for a different heart orientation (the beam is parallel to the aortic flow and the true apex is intercepted). Depending on the cut, three mitral cusps with two orifices may be seen on the two-chamber view (P3 next to the inferior wall, A2 in the middle, and P1 or A1 next to the anterolateral wall). Use Figure 32.3 for guidance. Reproduced with permission from Hanna EB, Quintal R, Jain N. Cardiology: Handbook for Clinicians. Arlington, VA: Scrub Hill Press, 2009. Figure 32.11(a) Diagram and (b) example of the subcostal view. Figure 32.12 Subcostal view with a medial tilt to visualize the IVC. A large IVC (>2.1 cm), as well as its lack of 50% collapse with inspiration or sniff, signals high RA pressure. IVC is measured at a level just proximal to its junction with hepatic vein (1-2 cm proximal to the RA). Figure 32.13 Arterial distribution of various echo segments on the short-axis view, long-axis view, and apical four-chamber view. Note that the inferior septum (on the short-axis view) and the basal septum (on the four-chamber view) are supplied by the RCA. If the four-chamber cut is angled a bit posteriorly, some of the visualized mid-septum may be supplied by the RCA as well. 2. Assess for LV dilatation and RV dilatation, which are associated with LV and RV systolic dysfunction, respectively. 3. Left ventricular hypertrophy (LVH) is characterized by an increased LV mass > 115 g/m2 in men, or > 95 g/m2 in women. Increased wall thickness, which often underlies LVH, is characterized by an interventricular septal thickness or a posterior wall thickness ≥ 1.1 cm in men, or ≥ 1 cm in women. The wall thickness is severely increased if it is ≥ 1.7 cm in men, or ≥ 1.6 cm in women. LVH is concentric when the walls are thick but the LV is not dilated. LVH is eccentric when the walls are thick and the LV is dilated. Concentric LV remodeling is characterized by thick walls without overall LVH; i.e., the LV mass is normal LV mass is calculated using the LV wall thickness and the LV diameter on the parasternal long-axis view. 4. Left atrial size is the “hemoglobin A1c” of the left heart; if LA size is normal, it is unlikely that there are any major systolic, diastolic, or left valvular issues. A quick way of assessing LA size is by comparing it to the aorta on the long-axis view. LA is enlarged if it is > 1.1× the aortic size. Normal LA end-systolic diameter is < 3.8 cm on the parasternal long-axis view (anteroposterior diameter) and on the apical four-chamber view (septal-lateral diameter); LA enlargement is severe if LA diameter > 5 cm. LA volume should be assessed using the planimetered LA areas on both the four- and two-chamber views (disk summation technique). This is the preferred method for LA size assessment: LA volume is normally < 34 ml/m2 of BSA; LA is severely enlarged if LA volume is > 48 ml/m2. Normally, the septum moves in towards the LV in systole, and relaxes towards the RV in diastole. Abnormal septal motion is characterized by a septum that moves out towards the RV in systole, or at least at one point of systole, leading to ineffective septal contraction; and moves in towards the LV in diastole, compressing the LV. Five differential diagnoses (Figures 32.15–32.18): As opposed to other diagnoses, pericardial processes, whether constriction or tamponade, are characterized by an abnormal septal motion that increases with inspiration and thus varies between beats, i.e., the septal collapse towards the LV in diastole varies with respiration. In other processes, the abnormal septal motion does not vary as much across beats. In addition, characteristic of constrictive pericarditis, a septal bounce may be seen during each diastole, representing an instantaneous change in the RV-to-LV push with instantaneous pressure changes. A septal motion abnormality may also be seen in patients breathing deeply, wherein the RV pushes the septum towards the LV in deep inspiration. Like pericardial processes, this septal position varies with respiration, but septal bounce is not seen. In a tachycardic patient, sorting out the respiratory effect may prove difficult. M-mode imaging is particularly helpful because of its high frame rate. The abnormal postoperative septal motion is related to the fact that, after cardiac surgery, the heart is fixed anteriorly to the thorax (meaning, the RV is fixated). During systole, the whole heart moves toward that fixation site, leading to what looks like septal motion abnormality. In fact, it is an abnormal anterior motion of the whole heart, including the posterolateral wall. Figure 32.14(a) Example of RV enlargement and RV volume overload on the parasternal short-axis view and apical four-chamber view. The interventricular septum is flattened and pushed toward the LV in diastole (lines). The interatrial septum is also bowing towards the LA (cross). (b) M mode across the lateral tricuspid annulus in 4-chamber view. The longitudinal excursion of this annulus over time is shown, and is called tricuspid annular plane systolic excursion (TAPSE). TAPSE reflects RV shortening/contraction, and is measured by any of the blue lines. It is reduced here (12 mm, normal ≥17 mm). The distance is the same whether the oblique or vertical blue line is used, as distances are only measured vertically on M mode (horizontal plane represents time). Figure 32.15 During systole, in LBBB: (1) the septum moves in towards the LV; (2) then the septum relaxes while the posterior wall moves in; (3) the septum moves in again at the end, not because it is contracting but because the RV is relaxing and pushing it. Thus, the septum moves in twice (1 and 3), while the posterior wall moves in between (2), when the septum is relaxed. The distance between the peak of (1) and the peak of (2) is the septal-to-lateral M-mode delay, an index of dyssynchrony (>130 ms → significant). Figure 32.16 M-mode imaging shows paradoxical septal motion of RV volume overload (outward in systole, inward in diastole). This paradoxical motion is seen in both inspiration and expiration; it may be a bit more prominent in inspiration, but unlike constrictive pericarditis or tamponade, it is not much more prominent. M-mode allows a fine analysis of how various structures move during the cardiac cycle. QRS is used to time events. Figure 32.17 Constrictive pericarditis. Two septal abnormalities and one posterior wall abnormality are seen on M-mode (D corresponds to the septal position in diastole): In RV volume overload, the RV pushes the septum towards the LV in all diastolic cycles with no marked respiratory variation: the abnormal motion is systolo-diastolic, not respiratory. Moreover, only one sharp septal motion towards the LV is seen in diastole. Figure 32.18 Pericardial processes are characterized by septal compression towards the LV in inspiration, and towards the RV in expiration. 1. Mitral valve (Figures 32.19–32.21) Figure 32.19 Posterior mitral leaflet prolapse. In systole, the leaflet prolapses posteriorly to the mitral annular plane (blue line). This leaflet is not just prolapsed but flail, as even its edge is tilted toward the LA. Severe MR is expected, and a torn, flopping chordae may be seen. Figure 32.20 Rheumatic mitral valve. (a) Long-axis view in diastole. See the hockeystick shape of the anterior leaflet (arrow), the tip of which looks attached to the stiff posterior leaflet (line), with no diastolic opening. In fact, both leaflets are tied together by the commissural fusion. Note the severe LA enlargement, due to MS in this case. Also, note the aortic valve calcification (white aortic valve leaflet tips [dot]). Make sure the aortic calcification is not a false impression related to a high echo gain; the fact that the aortic walls appear bright may be a hint to the increased echo gain. (b) Short-axis view. Commissural fusion (arrow) explains the “fish mouth” opening. Figure 32.21 Posterior mitral annular calcifications (MAC) in the long-axis and four-chamber views. These posterior calcifications project at the base of the posterior leaflet on the long-axis view and the base of both the posterior and anterior leaflets on the four-chamber view. Anterior MAC in the long-axis view suggests radiation heart disease. Figure 32.22 Difference in aortic orifice shape between the tricuspid and bicuspid aortic valves (short-axis view). Note that one cusp is larger than the other in bicuspid aortic valve, and hence the aortic opening/closure becomes eccentric; this is evident on the M-mode analysis of the aortic valve. The leaflets open in a domed fashion on a longitudinal view (long-axis view), as they are restricted by the lack of a third commissure. A severely stenotic bicuspid valve may appear to open well on the long-axis view. The stenosis being eccentric, it is three-dimensionally more severe than it may appear on a two-dimensional view. LC, left coronary cusp; NC, non-coronary cusp; RC, right coronary cusp. 1. Color Doppler is performed at the level of each valve to assess regurgitation (see Figures 32.23–32.33) By “eyeballing” the view, regurgitation appears as a color going backward between chambers, opposite to the normal flow (e.g., any flow from LV to LA, RV to RA, or aorta to LV). It is blue (backward) for the mitral and tricuspid valves on TTE. It usually has a higher velocity than the Nyquist limit, which leads to color aliasing (= mixed, mosaic color). Also, when severe, it is usually turbulent, with high variance of velocities (turbulent flow, coded as green color). 2. CW Doppler is performed at the level of each valve to assess forward-flow velocity, and, consequently, valvular stenosis (see Figures 32.34, 32.35, 32.36) Normally, the forward peak velocity across each valve is 1 m/s. An increase in flow velocity corresponds to valvular stenosis. The peak pressure gradient across a valve can be estimated using this equation (modified Bernoulli equation): This is how gradient is estimated across the aortic valve and the severity of a stenosis is assessed. For spectral Doppler assessment, it is important to obtain a view parallel to the flow. 3. PW Doppler is used to see the velocity at one particular point, such as the mitral inflow (E/A), tricuspid inflow, pulmonary vein inflow (systolic, diastolic, atrial waves), and LVOT flow (Figures 32.37, 32.38) PW has a limited capacity to measure high velocities that exceed twice the Nyquist limit (>2 m/s), particularly at greater depths. Two types of velocities are analyzed on CW or PW Doppler: peak velocity and velocity–time integral (VTI).VTI corresponds to the area enclosed by the CW or PW Doppler velocity profile. It is measured in cm (velocity × time) and corresponds to the distance traveled by blood across the interrogated point during one cardiac cycle. 4. Tissue Doppler assesses the movement of cardiac structures rather than blood flow (Figures 32.39, 32.40) Tissue Doppler is useful to assess: The global ventricular function may also be simply and routinely assessed using annular tissue Doppler. On the annular Doppler, S’ is the forward systolic movement of the annulus in systole (longitudinal fibers). S’ ≤ 7 cm/s correlates with LV systolic dysfunction. At the tricuspid annulus, S’ < 10 cm/s correlates with RV systolic dysfunction. Volume and flow can be derived from velocity using the continuity equation, which states that blood volume that crosses a cardiac area during one cardiac cycle = area × VTI. Thus: Stroke volume is, thus, equal to: 0.785 × LVOT d2 × LVOT VTI Cardiac output is equal to: stroke volume × heart rate LVOT diameter should be measured at the insertion of the aortic valve leaflets (i.e., at the annulus, not below it), parallel to the leaflets’ plane, in early systole (largest diameter). A falsely low LVOT measurement is a common cause of a falsely low AVA. Capture of TR jet by CW Doppler is necessary for the calculation of PA pressure. While many normal individuals have mild TR that allows this calculation, over 50% of patients, including some patients with severe pulmonary hypertension, do not have an adequate TR jet envelope. According to the Bernoulli equation, the pressure difference between RV and RA in systole equals 4 × VTR 2. Thus, RV systolic pressure = 4 × VTR 2 + RA pressure. In the absence of pulmonic stenosis, RV systolic pressure is equal to PA systolic pressure. RA pressure is assessed on the basis of IVC diameter and inspiratory collapse: or IVC > 2.1 cm with > 50% inspiratory collapse → RA pressure = 5–10 mmHg Figure 32.23 MR, four-chamber view. The blue, backward flow between the LV and the LA is MR. The regurgitation can be graded by measuring the regurgitant (blue) area (arrow) and comparing it to the LA area. If the regurgitant area is > 40% LA area → severe MR (this also applies for TR: jet area > 30% of RA area → severe TR). This provides a quick idea of the severity of MR, but is not very reliable. Increasing the color gain or lowering the Nyquist limit of the backward flow on the color scale (horizontal arrow) increases the regurgitant/turbulent area and makes the MR look more severe. The best Nyquist limit for regurgitation assessment is 50–60 cm/s. To obtain the best color gain, increase the gain until noise is seen in cardiac tissues, then slightly reduce it just until the noise disappears. Reproduced with permission from Hanna EB, Quintal R, Jain N. Cardiology: Handbook for Clinicians. Arlington, VA: Scrub Hill Press, 2009. Figure 32.24 Severe MR on four-chamber TEE view. Severity criteria of MR: (PISA radius)2/2 If PISA radius is 0.9 cm, ERO is ~0.4 cm2. MR is severe if ERO ≥ 0.4 cm2. ERO can be used to calculate the regurgitant volume (= ERO × VTI of the regurgitant flow) and the regurgitant fraction. Regurgitant volume > 60 ml or regurgitant fraction > 50% signifies severe MR. PISA is affected by eccentricity of the jet, but less than MR jet area; another limitation is that the PISA should be a 180° hemisphere. A flattened, squashed PISA has a wider base but a shorter diameter (shorter double arrow); the short diameter underestimates the PISA area as the calculation assumes PISA is round. When MR seems severe but LA is not enlarged, make sure that MR is present throughout systole. What seems like severe MR by color or PISA is moderate if it only encompasses 50% of systole or end-systole (this may occur with mitral valve prolapse and with functional MR). The duration of MR is evaluated by CW Doppler or by color M-mode across the mitral valve. Figure 32.25 MR, long-axis view. The blue flow between LV and LA is MR (arrow). It is eccentric, posteriorly directed, and at least moderate in severity. When MR is eccentric, consider it more severe than it appears (MR that appears mild is likely moderate). An eccentric jet that turns around the left atrial wall in a circular way is severe MR (Coanda effect). A well-visualized PISA hemisphere (arrowhead) despite a Nyquist limit of 69 cm/s is concerning for severe MR. A posteriorly directed MR usually implies either anterior leaflet prolapse or posterior leaflet tethering from inferior akinesis. Figure 32.26 Systolic flow reversal of pulmonary venous flow in a patient with severe MR. Normally, S flow has the same direction as D flow. Figure 32.27 CW Doppler across the mitral valve on an apical four-chamber view. The flow is directed backward (arrow) from the LV to the LA, and projects below the baseline on CW Doppler. (a) The flow is dense (white) but not as white as the forward flow, and thus is probably moderate MR. (b) Acute or decompensated severe MR may lead to a late indentation of the CW signal, related to a large V wave and decreased LV–LA pressure gradient at end-systole. This is called the V-wave cutoff sign, and leads to an early-peaking, triangular MR shape. On the apical views, because of similarities in direction of AS and MR jets and because of beam width artifact, AS Doppler interrogation may capture MR jet, creating the false impression of severe AS velocity. Unlike AS, MR jet starts immediately at MV closure, immediately after the mitral inflow A wave, at the peak of R wave on the ECG (white line). The timing of the two jets and a back-and-forth sweeping of the transducer help differentiate the two. Figure 32.28 Severe TR seen from the short-axis view (aortic valve level) (arrow). The same criteria described under Figure 32.24 can be used for TR to assess severity. To estimate ERO, set the backward flow aliasing limit at 28 cm/s (instead of 40). Also, annular dilatation ≥ 3.5 cm correlates with severe functional TR. Use the same vena contracta and ERO values as in MR, use jet area > 30% RA area, and look for hepatic vein flow reversal and RA enlargement. Reproduced with permission from Hanna EB, Quintal R, Jain N. Cardiology: Handbook for Clinicians. Arlington, VA: Scrub Hill Press, 2009. Figure 32.29 TR. CW Doppler across the tricuspid valve on a four-chamber view (see that the line swept is on the right side, arrow). TR looks very dense (white) on CW Doppler, which often means large-volume moderate or severe regurgitation. Unlike the density of the regurgitation, the peak velocity does not correlate with the severity of the regurgitation. This peak velocity correlates with the pressure gradient between the two chambers (here: RV to RA), and hence PA pressure. Severe pulmonary hypertension may occur with mild, non-dense TR, yet the velocity would be high (>4 m/s); conversely, severe, dense TR may be seen with a low TR velocity when PA pressure is not elevated (e.g., primary TR). Figure 32.30 Aortic insufficiency (AI). Long-axis view shows blue–backward flow from the aorta to the LV, i.e., AI. Assess AI severity by looking at: In (a), AI is moderate; in (b), AI is mild. Figure 32.31 AI. The width of the AI jet may also be assessed on the aortic short-axis view. Here, look for a central blue or mixed-color “flame” in diastole (arrow), which corresponds to AI jet. AI jet area that is > 60% of the whole aortic circle area implies severe AI. AI jet may look falsely enlarged if the cut is well below the regurgitant orifice, or falsely reduced if AI is eccentric. In order to obtain the accurate AI width, the transducer may be angled up until the AI flow is lost; the AI that is seen just before the flow is lost corresponds to the jet width. The latter maneuver is best performed on the TEE’s aortic short-axis view. Reproduced with permission from Hanna EB, Quintal R, Jain N. Cardiology: Handbook for Clinicians. Arlington, VA: Scrub Hill Press, 2009. Figure 32.32 AI on three-chamber apical view. This view is not accurate for measurement of the jet width area and vena contracta. Being parallel to the flow, it is excellent for recording of Doppler flow and measurement of AS gradient or AI pressure half-time, but it is not accurate for width measurement. When assessing thickness of a structure or flow, it is best to be orthogonal to the structure or flow. Note AI’s PISA, which is the spherical jet on the side of the aorta. Figure 32.33 AI spectral Doppler assessment on an apical five-chamber view. The flow between the aorta and the LV is directed upward (along the direction of the arrow). The slope of this regurgitant flow depends on how closely the LV and aortic pressures approximate during diastole, and thus how severe and acute AI is. The steeper the slope, the more severe and quick is the rise of LV diastolic pressure. This is called pressure half-time (PHT), which is the time needed for the pressure gradient to decrease in half. If PHT < 250 ms → steep slope → severe AI. Note, however, that a chronic severe but compensated AI with compensated LV may have PHT > 250 ms. PHT correlates more with acuity and decompensation of AI than its severity. PHT of AI is different from PHT of MS, where a steeper slope implies less severe MS. Also, the density of this regurgitant flow correlates with severity (the whiter it is in comparison to the forward flow, the larger the AI volume and the more severe the regurgitation). In this case, note that the patient has AI with PHT < 250 ms but also significant AS, with a peak forward velocity of 4 m/s. AI exaggerates the AS velocity (increased stroke volume), while AS may exaggerate AI severity by the PHT method, as it impairs LV compliance. Figure 32.34 AS. CW Doppler across the aortic valve on the apical five-chamber view. The flow directed down is the aortic flow in systole (along the direction of the arrow). The normal peak velocity is 1 m/s. Velocity > 2 m/s signals AS; velocity ≥ 4 m/s implies severe AS, which is the case here. The peak pressure gradient may be calculated from peak velocity using the modified Bernoulli equation (= 4 V2). The mean pressure gradient integrates all the gradients underneath the velocity envelope. Echo may underestimate the pressure gradient if the interrogation angle is not parallel to the flow, or if the jet envelope is incomplete (may need to increase the gain settings). Search for the best envelope and the highest gradient in multiple views (apical five-chamber, apical three-chamber, right parasternal, and suprasternal views). Caution: increased CW velocity is not necessarily AS, because CW samples the highest velocity along the whole line swept and not only the aortic valve. It may be LVOT obstruction, where the velocity is increased across the LVOT rather than the aortic valve. In this case, the PW velocity is increased across the LVOT, whereas the localized aortic PW velocity is not increased (PW localizes the site of obstruction, even though it may not be able to record the exact velocity). Also, in LVOT obstruction, the gradient peaks late and the CW velocity has a late-peaking dagger shape. In low-output states, the gradient may be low despite severe AS. Severe AS is diagnosed by using the dimensionless index (LVOT PW velocity divided by aortic valve CW velocity). Either VTI or peak velocity may be used. The index is normally ~1, and an index < 0.25 implies severe AS with AVA<25% of normal. If the index is >0.25, AS is likely non-severe, even if AVA measures <1 cm2 (–>false LVOT diameter measurement). In AF, the velocity and the gradient decrease after the short R–R cycles (as opposed to MS). For AVA calculation, use LVOT VTI and aortic valve VTI obtained after the same R–R cycle lengths. For peak/mean gradient estimation, control the rate and average the gradients from several beats; however, since AF reduces cardiac output, AS severity is better gauged by the highest gradient (long R-R) than by averaging down gradients. AS gradient is often underestimated, not overestimated. AS gradient may rarely be overestimated when AS and MR jets are confused on the apical five-chamber or three-chamber view. MR jet starts exactly at the peak of R wave, whereas AS jet starts a bit later. Figure 32.35 Severely increased velocity on aortic Doppler. Several features suggest LVOT obstruction rather than AS: (1) the velocity has a late-peaking dagger shape (tilted line), and is “skinnier” than the aortic velocity seen in Figure 32.34; (2) on color Doppler, the aliasing (arrow) occurs below the aortic valve level (double arrow). To confirm LVOT obstruction, PW Doppler may be swept throughout the LVOT and the aortic valve. PW Doppler cannot record velocities over 1.5–2 m/s, but will indicate that the velocity is increased across the LVOT rather than the aortic valve. Exact assessment of the velocity with CW ensues. Figure 32.36 MS assessment. (a) CW Doppler across the mitral valve (MV) on the apical four-chamber view. The flow is directed from the LA (which is down on this view) to the LV (up) along the direction of the arrow. A mean gradient > 5 mmHg at rest, or 10–15 mmHg with exercise, corresponds to severe MS. Passive leg raising should be performed to assess stress gradient. CW, not PW, should be used to capture the gradient. The downslope of the rapid diastolic filling (E wave, first wave) may be used to estimate MV area: a slow downslope means that LA pressure does not equalize with LV pressure even in late diastole (no diastasis), which corresponds to severe MS. The pressure half-time is the time it takes the pressure gradient to decrease in half [i.e., time it takes E velocity to decrease by 30%], and is long in severe MS. MV area = 220 divided by pressure half-time. However, for the same valve area, LA and LV pressures more readily equalize in case of increased LV diastolic pressure (AI, severe LVH), which makes the downslope look steeper → MV area will be seemingly larger and MS will be seemingly less severe. High E wave may merely result from MR or HF and when MAC coincides, this high E may be falsely considered high gradient and MS. Unlike MS, E wave of the latter cases has a sharp downslope. (b) If E wave has a “ski slope” shape, (i.e., initially steep then slow downslope), use the slow downslope portion to calculate the pressure half-time. An associated MR or high output state increases the early E-wave velocity and the overall gradient, but the later E slope remains unchanged. In AF, the pressure gradient varies between different beats (↑ with short R–R cycles, as LA emptying decreases), but the shape and slope of the CW envelope remain unchanged. Mean gradient increases as in any tachycardia (or exercise), but pressure half-time and MV area remain unchanged. PHT = pressure half-time. Figure 32.37 PW Doppler at the level of the mitral valve. During diastole, forward flow is recorded across the MV: E is the rapid diastolic filling, A is the filling related to atrial contraction. E occurs after T wave, while A occurs after P wave and almost coincides with QRS. Figure 32.38 PW Doppler across the pulmonary veins on a four-chamber view, pulmonary veins being behind the LA (cursor). S and D represent, as it is seen, forward systolic and diastolic flow toward the LA. A wave corresponds to atrial systole, when LA pressure increases and prevents forward blood flow: A is a reversed flow wave. Normally, S is slightly > D or slightly < D. A severely reduced S wave implies elevated LA pressure. The hepatic venous flow on the subcostal view looks similar to the pulmonary venous flow (S, D, A), except that S and D waves are below the baseline, whereas A wave is above the baseline. Figure 32.39 Myocardial tissue Doppler at the level of the mitral annulus. The cursor is placed on the myocardial lateral or medial annulus. E’ and A’ waves occur in diastole, on the downward negative side (diastolic recoil). In systole, a forward positive motion S’ occurs. Lateral E’ annular velocity is usually higher than medial E’. Measure both lateral and medial E’, and calculate E/E’ for each E’, and for the average E’. The cursor should be positioned within 0.5 cm of the leaflet insertion site and should be parallel to the plane of cardiac motion. In myocardial dysfunction (systolic or diastolic), IVCT and IVRT increase, leading to an increase of the following ratio: (IVCT + IVRT)/ejection time (ejection time corresponds to S’ time). This ratio, called myocardial performance index or Tei index, may be calculated using myocardial annular Doppler and is normally < 0.4. Reproduced with permission from Hanna EB, Quintal R, Jain N. Cardiology: Handbook for Clinicians. Arlington, VA: Scrub Hill Press, 2009. Figure 32.40 2D tissue strain imaging of the LV on a two-chamber view. Strain between two myocardial points = % change in distance between the two points = (distancebetween these points in systole – distancein diastole)/distancein diastole. Normal regional strain values are –20 to –12; abnormal values are –8 to +20. The red color corresponds to normal strain, while the blue/purple corresponds to abnormal strain. In this figure, the antero-apex is blue and therefore dysfunctional. The time display (right image) shows the peak systolic strain of each segment and the strain curve throughout systole then diastole; the segment moving in the opposite direction to the baseline is a dyskinetic segment (apex). Courtesy of Carmen Ilie and Fred Helmcke, who provided this image. Table 32.1 Severe valvular regurgitation. This supports severe MR, but dense flow may also be seen with moderate MR Important supportive features Important supportive feature RA/IVC size is always increased in severe chronic TR RV is always enlarged in chronic severe PR. In a patient with significant PR, RV enlargement without any other cause suggests severe PR Table 32.2 Severe valvular stenosis: severe AS, including low-gradient AS, and severe MS. LVOT velocity may be > 1 m/s when severe AS is associated with moderate AI or high-output states (anemia, fever), cases in which DI remains < 0.25; or when severe AS is associated with significant septal bulge and subaortic obstruction. It may be falsely elevated if the LVOT cursor is placed too close to the aortic valve or the septal bulge, in the aliasing Differential of paradoxical low-gradient AS with normal EF (AVA ≤ 1 cm2 with low gradient < 40 mmHg): AS is likely severe if AVA≤ 0.8 cm2 with mean gradient >30 mmHg; or if DI ≤0.25. Doppler assesses the transmitral gradient very accurately, as it is easy to align the cursor with the transmitral flow. Caveats: The estimation of MVA using one of 4 methods (mitral inflow pressure half-time, continuity equation, PISA method, and planimetry) is subject to measurement errors. Pressure half-time may falsely ↓ and MVA may falsely ↑ in patients with LV dysfunction (HTN, elderly) a AVA = (0.785 × LVOT diameter2) × LVOT velocity/aortic valve velocity. LVOT is assumed to be circular, but it is in fact elliptical and underestimated. LVOT diameter should be measured at the insertion of the aortic valve leaflets (= annulus), rather than 5-10 mm below it (LVOT is largest and most circular at the annulus). LVOT is measured in the long-axis view, in early systole (largest diameter, inner edge to inner edge). In the presence of protruding annular calcification, LVOT measurement is extended beyond the outer edge of the calcification. LVOT velocity is obtained by positioning the pulsed Doppler ~5 mm proximal to the stenotic valve (not too close to the valve). Avoid catching the high-velocity convergence proximal to the aortic valve. In case of septal bulge, place the cursor further down, away from the bulge. Either VTI or peak velocity may be used. M-mode echocardiography is derived from 2D echo (see Figures 32.41, 32.42, 32.43; also Figures 32.15–32.18). M-mode graphically displays the movement of cardiac structures along one line swept by the probe. It can assess valvular opening, chamber size, and subtle abnormalities of cardiac motion (such as RV compression by pericardial effusion). It has a very high temporal resolution and rapid sampling rate (1000 frames/s vs 30/s for 2D) that allows recording of subtle motion and timing of cardiac events. A pericardial effusion is small if < 1 cm, moderate if 1–2 cm, and large if > 2 cm. The effusion is measured as the summation of the anterior and posterior dimensions at end-diastole, i.e., when it appears smallest. A small effusion is usually localized posteriorly, while a large effusion is usually circumferential. A swinging heart, i.e., a heart that changes position in a phasic manner, may be seen with large effusions. An echo-free space that is present only anteriorly suggests an epicardial fat pad rather than a pericardial effusion; unless loculated, a pericardial effusion usually gravitates and predominates posteriorly in the supine position, or is circumferential. IVC plethora has a sensitivity of 97% for tamponade, but a specificity of only 40%, as it may occur with any right heart failure. On hepatic vein Doppler, a flat D wave (Y descent) corresponds to impeded right-sided diastolic filling and thus tamponade. Respiratory E variations are the earliest and least specific pre-tamponade findings. They are followed by progressive RA/RV collapse. Tamponade is a clinical diagnosis. The echocardiographic signs appear earlier than clinical tamponade and pulsus paradoxus; they suggest hemodynamic abnormalities that are the substrate for tamponade, but on their own they do not establish the diagnosis of tamponade. Tamponade may occur as a result of a localized effusion compressing one particular chamber, such as RV, LV, LA, RA, or pulmonary veins, as after cardiac surgery. This is more difficult to diagnose, and only some of the tamponade echocardiographic signs are seen. TEE may be more helpful in showing the localized effusion and cardiac chamber compression (e.g., isolated pulmonary venous compression). Figure 32.41 M-mode across the mitral valve on the parasternal long-axis view. The first structure intercepted, at the top, is the RVOT wall (white line); the second structure is the RV cavity (dark); the third structure is the septum; the fourth structure is the anterior leaflet of the MV (closes in systole and opens in diastole), and the fifth structure is the posterior mitral leaflet. The anterior mitral leaflet has two waves in diastole: E (rapid filling) and A, similar to the mitral inflow Doppler. Examples of disease states: By moving the interrogation line towards the mid LV, the systolic movement of the septum and the LV posterior wall can be assessed (see Figures 32.15–32.18). Figure 32.42 M-mode across the aortic valve on the long-axis view. The first structures encountered are the RVOT wall and cavity. Then the aortic walls (rather than LV walls) are encountered and, inside them, the aortic valve. The aortic valve opens well here (open box in systole [arrow]). Disease states: Reproduced with permission from Hanna EB, Quintal R, Jain N. Cardiology: Handbook for Clinicians. Arlington, VA: Scrub Hill Press, 2009. Figure 32.43 Examples of M-mode across the mitral valve.(a) Posterior mitral leaflet prolapse. See how the posterior leaflet moves posteriorly in mid-to-late systole, creating a “gap” in the mitral closure, and thus MR (arrows). (b) SAM of the anterior leaflet in HOCM, with the anterior leaflet touching the septum in systole, creating a gap away from the posterior leaflet. (c) Flat EF slope in diastole suggests MS (mitral valve is steadily open throughout diastole, with no diastasis). Also, the posterior leaflet is pulled towards the anterior leaflet (commissural fusion) (arrow). Ant, anterior mitral leaflet; Post, posterior mitral leaflet. Figure 32.44 Diffuse pericardial effusion on long-axis view, identified as a black band above the RV (upper arrow) and a black band posterior to the LV. In this case, posterior to the LV, there are two black bands separated by the pericardium: pericardial effusion and pleural effusion (lower arrows). Differentiate pleural from pericardial effusion: the pericardial effusion is anterior to the descending aorta (X), whereas the pleural effusion extends behind the aorta. The coronary sinus is in the AV groove, anterior to the pericardium (dot). Reproduced with permission from Hanna EB, Quintal R, Jain N. Cardiology: Handbook for Clinicians. Arlington, VA: Scrub Hill Press, 2009. Figure 32.45 Pericardial effusion on multiple views. In a supine position, a free-flowing effusion gravitates and predominates over the posterior aspect of the LV. The posterior aspect is the one seen on the long-axis view (posterolateral aspect) and the four-chamber view. This has to be distinguished from the effusion at the inferior/diaphragmatic aspect of the LV, which is more anterior and is the one seen on the subcostal view (used for subcostal pericardiocentesis). Figure 32.46 (a) Pericardial effusion (stars) and pleural effusion (bar). The latter is behind the level of the descending aorta. RVOT is compressed in diastole, at a time when the mitral valve is open (RVOT diastolic indentation is marked by arrow). (b) RVOT collapse in early diastole on M-mode. Always time events to the ECG; systole starts at the peak of R wave and occupies the ST–T segment, whereas diastole starts beyond the T wave. Events may also be timed to the mitral opening on M-mode. After the systolic dip, the RVOT should be expanding outward in diastole (as in the solid line), rather than pushed inward (dashed line). The presence of two dips, a systolic dip and a diastolic dip soon after the RV starts to expand out, is characteristic of tamponade. For the diastolic dip to be diagnostic, the M-mode has to cut the RVOT in an orthogonal fashion. D, diastole; S, systole. (see Chapter 5, Figure 5.2) Diastolic E flow and E/A ratio-Diastolic E flow is affected by: (1) LA pressure, (2) LV relaxation, which is impaired in both diastolic dysfunction and systolic dysfunction, and (3) heart rate and PR interval. Impaired LV relaxation reduces E velocity; however, severe hypovolemia with low left-sided filling pressure may reduce E and E/A ratio even in the absence of a relaxation problem. Prolonged PR interval and sinus tachycardia reduce E and E/A ratio and may be associated with E–A fusion without any relaxation problem. On the other hand, high left-sided filling pressures but also high elastic recoil in normal young patients may elevate E velocity. One echocardiographic parameter correlates solely with LV relaxation and is thus reduced in any LV dysfunction, systolic or diastolic, regardless of filling pressure: mitral annular recoil velocity (E’). Therefore:
32
Echocardiography
1. GENERAL ECHOCARDIOGRAPHY
I. The five major echocardiographic views and the myocardial wall segments
II. Global echo assessment of cardiac function and structure
A. Global assessment of myocardial function
1. Overall assessment of LV function
B. Paradoxical septal motion
C. Valvular structure assessment
2. Aortic valve
III. Doppler and assessment of valvular regurgitation and stenosis
A. Types
B. Routine Doppler interrogations
C. Routine Doppler calculations
1. Volume and flow calculations
2. Aortic valve area (AVA) calculation using the continuity equation
3. Calculation of the systolic PA pressure using the TR jet
4. Assessment of RA pressure
IV. Summary of features characterizing severe valvular regurgitation and stenosis (see Tables 32.1, 32.2)
Mitral regurgitation
Pros: area <20% makes severe MR very unlikely
Cons: eccentricity reduces jet area; low color gain and high Nyquist falsely reduce the jet area. Conversely, inappropriately high color gain and low Nyquist limit falsely increase the jet area.
Pros: accurate, even in eccentric MR. Vena contracta < 3 mm makes severe MR unlikely.
Cons: does not reliably distinguish moderate from severe MR. Also, should be measured in a view orthogonal to the regurgitation (parasternal long-axis), not an apical view, which tends to falsely widen the vena contracta. It is not accurate if multiple jets are present.
Cons: may underestimate MR if jet is eccentric (but better than jet area in this case), if multiple jets are present, or if PISA convergence is squashed rather than round (crescentic PISA), as in functional MR with dilated LV; thus, 0.3 cm2 is a better ERO cutoff in functional MR. It may overestimate MR if convergence is not a full hemisphere.
Pros: very sensitive, regardless of eccentricity. A lower E velocity or E/A reversal ~ excludes severe MR.
Cons: not specific. E velocity increases with high LA pressure and AF.
Cons:
Aortic insufficiency
AI jet width ≥ 60% of LVOT diameter (long-axis view)
AI jet cross-sectional area ≥ 60% of LVOT area (short-axis view) at a Nyquist limit of 50–60 cm/s
Note: AI jet size is different from vena contracta. AI jet size is the AI size just below the vena contracta, as the jet starts to expand (within 1 cm of the aortic valve)
Cons: eccentric AI, such as bicuspid AI, may be underestimated, because the jet abuts the mitral valve or the septum and shrinks in velocity (color). Color doppler correlates with velocity more than volume; the regurgitant volume may be high, yet color may abate quickly. This is even more so when assessed in a 2D plane where it is narrowest (in the long-axis view). Conversely, AI may be overestimated when the measurement is performed too low below the vena contracta, or in the apical views
Cons: the rounded flow convergence is more difficult to measure in AI than in MR, as it is shadowed by the thick +/- calcified aortic leaflets.
Cons: depends on LV compliance and LVEDP. PHT may be > 250 ms in chronic, compensated severe AI. PHT may be < 250 ms in moderate AI with decompensated LV dysfunction and high LVEDP (e.g., severe HTN). Also, vasodilators may reduce diastolic aortic pressure and thus, PHT.
PHT > 500 ms ~ excludes severe AI
Tricuspid regurgitation (same type of features and limitations as MR)
Pulmonic regurgitation
Aortic stenosis
Peak velocity ≥ 4 m/s, mean gradient ≥ 40 mmHg, and AVA ≤ 1 cm2 by continuity equation a
Typically, in severe AS:
Mitral stenosis (see Section VIII.C)
V. M-mode echocardiography
VI. Pericardial effusion
A. Size (see Figures 32.44, 32.45)
B. IVC plethora
C. Pre-tamponade echocardiographic signs (see Figure 32.46)
VII. Echocardiographic determination of LV filling pressure and diastolic function
A. Main parameters
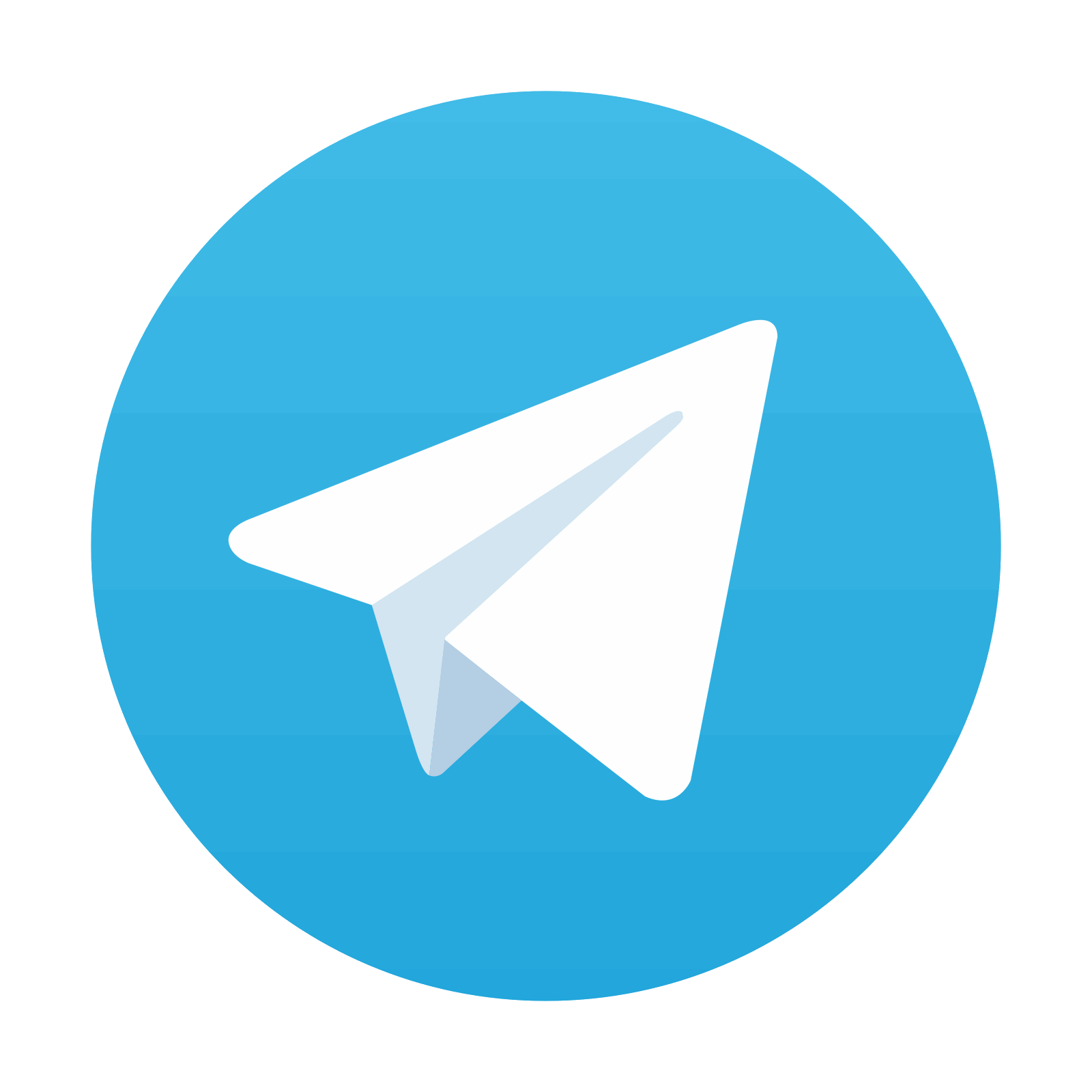
Stay updated, free articles. Join our Telegram channel

Full access? Get Clinical Tree
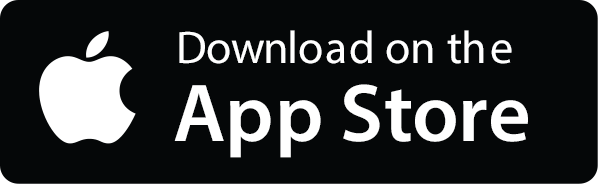
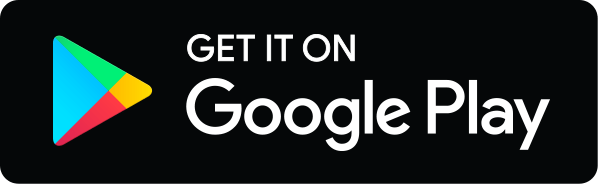