Background
Continuous-flow left ventricular assist devices (LVADs) have become part of the standard of care for the treatment of advanced heart failure. However, knowledge of normal values for transthoracic echocardiographic examination and measurements in these patients are lacking.
Methods
All transthoracic echocardiographic examinations in 63 consecutive patients, performed 90 and 180 days after surgery with the implantation of a HeartMate II continuous-flow LVAD between February 2007 and January 2010, were retrospectively analyzed. All patients had to be outpatients at 3 and 6 months after surgery and considered stable on LVAD therapy (New York Heart Association class I or II and no need for inotropes, intravenous furosemide, or hospitalization).
Results
End-diastolic and end-systolic diameters and left ventricular mass decreased considerably compared with baseline measurements before LVAD implantation. Mitral inflow deceleration time increased (188 ± 70 vs 132.5 ± 27 msec, P = .009) and left atrial volume (84.1 ± 33 vs 141.7 ± 62 mL, P = .003) and E/e′ ratio decreased (20.3 ± 9 vs 26 ± 11, P = .01), all consistent with decreased left ventricular filling pressure. Estimated right ventricular (RV) and right atrial pressure decreased significantly (34.1 ± 10 vs 51.7 ± 14 mm Hg and 9.5 ± 5 vs 14.4 ± 5 mm Hg, respectively, P < .0001 for both). Quantitatively estimated RV function ( P = .02), RV fractional area change (27.9 ± 10% vs 37.4 ± 10.9%, P < .0001), and the RV index of myocardial performance (0.32 ± 0.1 vs 0.65 ± 0.2 vs 0.32 ± .01, P < .0001) improved, suggesting improved RV efficiency. LVAD therapy resulted in significant decreases in the severity of mitral regurgitation. Tricuspid regurgitation improved in patients who had concurrent tricuspid surgical correction and was unchanged otherwise. Aortic regurgitation severity increased 3 months after LVAD implantation. There were no significant differences in any of the echocardiographic parameters in the 6-month evaluation compared with the 3-month evaluation.
Conclusions
This is the first report of selected typical echocardiographic values in a group of stable patients with normally functioning HeartMate II continuous-flow LVADs. A stable functioning continuous LVAD is associated with evidence of efficient unloading of the left ventricle, improved RV function, significant improvement in mitral regurgitation, improvement in tricuspid regurgitation only in patients undergoing repair, and increased aortic regurgitation. These normal data provide a basis for future echocardiographic studies after LVAD implantation.
Left ventricular (LV) assist devices (LVADs) are designed for mechanical support for patients with severe systolic heart failure. LVAD therapy provides effective long-term circulatory support as a bridge to transplantation or as destination therapy. In most cases, axial flow pumps have replaced pulsatile LVADs because they have been shown to have improved survival and have less device failure. Recently, the HeartMate II continuous-flow assist device (Thoratec Corporation, Pleasanton, CA) was approved for use as a bridge to transplantation or as destination therapy by the US Food and Drug Administration.
Preoperative and postoperative transthoracic echocardiography has a major role in the management of patients with LVADs. Transthoracic echocardiography is frequently used to assist in patient selection for LVAD therapy, evaluation of proper native heart and LVAD function, and troubleshooting for possible device malfunctions. Although continuous LVAD therapy has been in clinical use for a number of years, a comprehensive summary of normal values for the different echocardiographic measurements in these patients has not been reported to date. We report here for the first time echocardiographic parameters, including LV linear dimensions, chamber areas, valvular function, and Doppler evaluation, obtained from a large patient population having undergone successful LVAD implantation with HeartMate II devices, with improved functional outcomes (New York Heart Association [NYHA] class I or II and no need for inotropes, intravenous furosemide, or hospitalization) at 3 and 6 months after LVAD implantation. We also report the influence of a properly functioning LVAD system on the appearance and function of the different chambers, walls, and valves of the native heart.
Methods
Patient Population
We retrospectively analyzed data from 63 consecutive patients (52 men, 11 women) who had been supported for ≥90 days with continuous-flow HeartMate II LVADs between February 2007 and January 2010. All subjects were in NYHA functional class I or II at follow-up, with no echocardiographic or clinical evidence of device malfunction, no need for intravenous inotropic support or diuretics, and no other significant illness requiring hospitalization at the time of baseline (90 days after LVAD surgery) or 6-month echocardiographic examination. All 63 patients underwent comprehensive echocardiographic examinations preoperatively and at 90 days after LVAD surgery. Thirty-nine patients underwent follow-up echocardiographic examinations 180 days after surgery.
Echocardiographic Measurements
Two-dimensional transthoracic echocardiography was performed in a standard manner using the Sonos 5500 (Philips Medical Systems, Andover, MA), Sequoia 512 (Siemens Medical Solutions USA, Inc., Mountain View, CA), or Vivid 7 (GE Medical Systems, Milwaukee, WI).
LV diameters and interventricular septal and posterior wall width were measured from the parasternal short-axis view using two-dimensional or two-dimensionally guided M-mode echocardiography of the left ventricle at the papillary muscle level using the parasternal short-axis view from the trailing edge of the septum to the leading edge of the posterior wall, as recommended. Ejection fraction was calculated using the Quinones method, assuming an akinetic apex, and LV mass was calculated using the method proposed by Devereux et al. , using the same LV dimensions made with M-mode or two-dimensional echocardiography as described above.
Valvular regurgitation was qualitatively assessed using color Doppler according to the guidelines of the American Society of Echocardiography (normal or trivial = 1, mild = 2, moderate = 3, and severe = 4). Right ventricular (RV) chamber size and function were measured according to the guidelines of the American Society of Echocardiography. RV size and systolic function were evaluated by measuring RV end-diastolic area (four-chamber view), RV end-systolic area (four-chamber view), and tricuspid annular end-systolic diameter (four-chamber view) and calculating RV fractional area change. RV function was qualitatively graded using a four-point grading system (normal, mild, moderate, or severe) using all apical views and the RV inflow, parasternal long-axis, parasternal short-axis, and subcostal views. Care was taken to obtain a true, nonforeshortened apical or para-apical four-chamber view, oriented to obtain the maximum RV dimension before making the RV estimation. RV function was then assessed using two other methods: two-dimensional lateral tricuspid annular motion and the RV index of myocardial performance (RIMP), as previously described by others ( Figures 1 and 2 , Videos 1 and 2 ; view video clips online). Tricuspid annular diameter was measured at end-systole as well. The RV outflow tract (RVOT) velocity-time integral (VTI) was measured from pulsed-wave Doppler, and RVOT diameter was measured from the parasternal short-axis view at the level of the great arteries. Total RV output (the sum of LVAD output and the flow ejected through the LV outflow tract) was calculated using the formula (RV outflow diameter/2) 2 × π × RV outflow VTI. Mean pulmonary artery pressure was estimated using the modified Bernoulli formula: [4 × (peak systolic tricuspid regurgitation [TR] velocity at end-expiration) 2 ] + right atrial pressure estimated by the inferior vena cava diameter as well as its response to inspiration, as previously described. Measurements of the LVAD components were performed, including the inflow cannula, outflow graft flow velocities, and native aortic valve opening status ( Figures 3–5 ).





Inflow Cannula
The inflow cannula and its orientation within the LV apex were visualized in the apical four-chamber and two-chamber views and sometimes required off-axis imaging as well. Doppler assessment of the inflow cannula was done in the four-chamber and two-chamber views as well, because they are usually aligned with the central axis of a properly positioned inflow cannula. Continuous-wave Doppler was used for measurement of the maximal velocity along the inflow pathway from the ventricle to the LVAD. Maximal systolic and diastolic velocities were recorded using pulsed-wave Doppler or continuous-wave Doppler, and the ratio of systolic to diastolic inflow velocities was calculated ( Figure 3 , Video 3 ; view video clip online).
Outflow Cannula
Interrogation of the outflow cannula was performed from the high left parasternal long-axis view, which shows the end-to-side anastomosis of the outflow cannula to the mid ascending aorta, and the right parasternal view, which shows the long axis of the outflow cannula traversing from the pump toward the right aspect of the ascending aorta. Color flow, pulsed-wave, and continuous-wave Doppler were used to evaluate flow patterns of the outflow cannula. To measure flow velocity, the pulsed-wave sample volume was positioned ≥1 cm proximal to the aortic anastomosis, and the flow was recorded away from or toward the transducer, depending on the transducer position. For the right parasternal view, the patient was positioned on his or her right side, and the image was recorded with the transducer immediately rightward to the sternum ( Figure 3 , Videos 4–7 ; view video clips online).
The product of a pulsed-wave spectral Doppler VTI from the outflow cannula and its cross-sectional area equals the flow volume created by the pump. This technique is well known and was recently validated with the HeartMate II in a well-conducted in vitro study. The same method was applied here to calculate the LVAD minute flow rate ( Figure 5 ). LVAD outflow cannula diameter was measured in the right parasternal view, and outflow VTI was measured using the right parasternal view or the high left parasternal view, depending on which view afforded the lowest Doppler angle of interrogation. In some patients, the outflow cannula diameter could not be measured because of variations in imaging plane. We examined whether the pump’s flow volume could be estimated using a simplified method using the product of a pulsed-wave spectral Doppler VTI from the outflow cannula and an outflow cross-sectional area calculated assuming a diameter of 16 mm (which is the actual manufactured measurement) or any other constant.
LVAD Settings
We routinely use an echocardiographically guided “ramped speed study” to set the optimal pump speed before discharge. We generally use a fixed speed setting that falls midway between the minimum and maximum speeds on the basis of the surgeon’s preference and changes in ventricular dimensions, the position of the interatrial septum and interventricular septum, and the frequency of aortic valve opening ( Figure 4 ).
Tricuspid Repair and Replacement
Candidates for LVAD implantation are evaluated using echocardiography to assess native TR. Those with at least moderate TR (jet area/right atrial area ≥20% or vena contracta >4.5 mm) need to undergo tricuspid valve repair or replacement. For tricuspid valve repair, the valve was inspected for pathology. If TR was associated with annular dilatation, a flexible annuloplasty ring (either complete or incomplete) was implanted to tighten the tricuspid annulus. If TR was related to entrapped leaflets from defibrillator or pacemaker leads, the responsible portion of the leads was freed from the leaflets and tucked into the commissure between the septal and posterior leaflets within a modified Kay annuloplasty stitch. If uncorrectable TR pathology was noted (too many adhesions, significant valvular tenting, severe TR such that repair was not likely to hold), we proceeded with tricuspid valve replacement using a tissue valve.
Aortic Valve Stitch
Candidates for LVAD implantation were evaluated using echocardiography to assess for native aortic valve insufficiency. Those with aortic insufficiency due to significant structural defects in the aortic valve underwent aortic valve replacement or patch closure of the valve. However, the majority of patients with aortic insufficiency were corrected with a simple coaptation stitch placed at the central portion of the aortic cusps, as previously described. The ascending aorta was cross-clamped to arrest the heart. A lateral aortotomy incision was made, long enough to complete the anastomosis between the outflow graft and the aorta. An aortic root retractor was placed, and the native aortic valve was inspected through the incision. The cusps of the native aortic valve were carefully examined to make sure that no other structural problems could be causing the aortic insufficiency. If the central aortic insufficiency was due to poor coaptation, a simple coaptation stitch using pledget-supported 5-0 Prolene sutures at the center easily mended this problem. Using this approach, the aortic valve was still able to open for ejection, even though the effective orifice area of the aortic valve was diminished. After placement of the coaptation stitch, the anastomosis between the outflow graft and the aorta was completed in the usual fashion.
Interobserver and Intraobserver Variability
Interobserver variability was assessed by comparing the readings made by another independent echocardiographer in 15 randomly selected patients. Intraobserver variability was determined by having the first observer who measured the data in all patients remeasure the velocities in 15 patients ≥3 month apart. The degrees of interobserver and intraobserver variability were determined using the Bland-Altman method and the within-subjects coefficient of variation. The within-subjects coefficient of variation (calculated as ratio of the standard deviation of the measurement difference to the mean value of all measurements) provides a scale-free, unitless estimate of variation expressed as a percentage. We measured the intraobserver and interobserver reproducibility for the inflow cannula velocity, the outflow cannula velocity, and the outflow cannula VTI, and the variations in measurements were expressed as ratios.
Statistical Analysis
Data are presented as mean ± SD, as percentages, or as medians with first and third quartiles, as appropriate. Comparisons between patients before and after LVAD implantation were performed using the paired Student’s t test or Wilcoxon’s signed-rank test, as appropriate. To analyze potential factors that influence the progression in aortic regurgitation (AR) (dichotomized into worse or stable AR by comparing AR grade at the baseline, 3 month and 6 month evaluations), univariate analyses on the basis of logistic regression were performed on various parameters (clinical, hemodynamic, and echocardiographic). All P values were two sided, and values < .05 were considered statistically significant. All data were analyzed using JMP version 8.0 (SAS Institute Inc., Cary, NC).
Results
The demographic, clinical, and hemodynamic characteristics of all patients are listed in Table 1 . Most patients were men aged >60 years with ischemic cardiomyopathy. All patients had NYHA class IIIB or IV symptoms, with hemodynamic parameters consistent with severe heart failure, a mean LV ejection fraction of 19.4 ± 7.2%, mean peak oxygen consumption of 10.6 ± 2.2 mL/kg/min, and a mean cardiac index of 1.9 ± 0.5 L/min/m 2 .
Characteristic | Value |
---|---|
Clinical, surgical, and demographic | |
Age (y) | 63.8 ± 13.0 |
Men | 82.5% |
Previous sternotomy | 47.6% |
TV repair/replacement ∗ | 38.1% |
Aortic repair (Park’s stitch) ∗ | 7.9% |
NYHA class IV | 52.4% |
Destination | 61.9% |
Ischemic | 53.9% |
Presence of LBBB | 10.8% |
Hemodynamic variables | |
Heart rate (beats/min) | 77.6 ± 15.6 |
Systolic blood pressure (mm Hg) | 98.1 ± 11.5 |
Diastolic blood pressure (mm Hg) | 63.3 ± 8.1 |
Mean RA pressure (mm Hg) | 14.9 ± 6.5 |
Mean PA pressure (mm Hg) | 36.6 ± 9.6 |
RV dP/dt | 471.1 ± 213.1 |
Mean wedge pressure (mm Hg) | 23.7 ± 6.5 |
Cardiac index (L/min/m 2 ) | 1.8 ± 0.5 |
SVR (Wood units) | 18.3 ± 8.4 |
Hemoglobin (g/dL) | 12.2 ± 1.9 |
Platelet count (×10 3 /μL) | 170.8 ± 69.6 |
INR | 1.5 ± 0.7 |
Bilirubin (mg/dL) | 1.2 ± 0.7 |
Albumin (g/dL) | 3.7 ± 0.5 |
BUN (mg/dL) | 31.8 ± 16.4 |
Creatinine (mg/dL) | 1.5 ± 0.5 |
BNP (pg/mL) | 1,901 ± 1,619 |
Linear LV dimensions, chamber function, valvular function, and Doppler evaluation obtained from patients at preoperative baseline and at 3 months after LVAD surgery are presented in Table 2 . LV dimensions changed significantly with continuous LVAD support. LV end-diastolic and end-systolic diameters decreased considerably (−15.8 ± 12.9% and −20.8 ± 18.8%, respectively, P < .0001). Septal wall and posterior wall thickness increased, but the difference did not reach statistical significance ( Table 2 , Figure 1 ). The calculated LV mass decreased (−19.4 ± 25.2%, P < .0001), and the mean LV ejection fraction improved (19.4 ± 7.2% vs 26.2 ± 12.4%, P < .0001).
Variable | Before LVAD implantation | 3 months after LVAD implantation | P ∗ | 6 months after LVAD implantation |
---|---|---|---|---|
LV parameters | ||||
LV diastolic diameter (mm) | 68.3 ± 9.1 | 56.7 ± 11.4 | <.0001 | 57.4 ± 12.6 |
LV systolic diameter (mm) | 61.7 ± 9.0 | 47.8 ± 13.0 | <.0001 | 49.0 ± 13.9 |
Posterior wall thickness (mm) | 10.0 ± 1.8 | 10.1 ± 2.0 | .46 | 10.6 ± 1.5 |
Interventricular septal wall thickness (mm) | 10.3 ± 2.1 | 10.8 ± 3.3 | .15 | 10.8 ± 2.1 |
Ejection fraction (%) | 19.4 ± 7.2 | 26.2 ± 12.4 | .0004 | 27.7 ± 14.7 |
LV mass (g) | 383.6 ± 113.8 | 295.9 ± 118.9 | <.0001 | 314.8 ± 134.4 |
Diastolic parameters | ||||
LA volume index (mL/m 2 ) | 69.3 ± 30.0 | 42.5 ± 15.9 | .009 | |
E-wave velocity (m/sec) | 0.98 ± 0.35 | 1.0 ± 1.6 | .9 | 0.8 ± 0.2 |
E-wave deceleration time (msec) | 132.5 ± 27.0 | 188.0 ± 70.1 | .009 | 166.3 ± 48.4 |
Tissue Doppler e′ (septal wall) | 0.04 ± 0.01 | 0.04 ± 0.01 | .9 | |
E/e′ ratio | 26.0 ± 11.5 | 20.3 ± 9.5 | .01 | 13.9 ± 7.9 |
RV parameters | ||||
RV end-diastolic area (cm 2 ) | 32.0 ± 8.6 | 29.3 ± 6.4 | .0002 ‡ | |
RV end-systolic area (cm 2 ) | 23.2 ± 7.2 | 18.6 ± 5.6 | <.0001 ‡ | |
RV fractional area change (%) | 27.9 ± 10 | 37.4 ± 10.9 | <.0001 ‡ | |
Tricuspid annular systolic diameter (cm) | 3.6 ± 0.6 | 3.3 ± 0.5 | <.0001 ‡ | |
Estimated RA pressure (mm Hg) | 14.4 ± 5.1 | 9.5 ± 5.1 | <.0001 | 8.6 ± 4.9 |
Estimated pulmonary pressure (mm Hg) | 51.7 ± 14.3 | 34.1 ± 10.2 | <.0001 | 33.5 ± 10.6 |
RIMP | 0.65 ± 0.23 | 0.32 ± 0.16 | <.0001 | 0.44 ± 0.27 |
TV lateral annular velocity (m/sec) | 0.08 ± 0.02 | 0.08 ± 0.03 | .4 | 0.07 ± 0.01 |
RV functional grade † | 3 (2.5–4) | 3 (2–3.5) | .02 | |
Valvular parameters | ||||
AR grade † | 0 (0–0) | 0 (0–1) | .004 | 1 (1–1) |
AR VC (mm) | 0.38 ± 0.9 | 2.0 ± 1.6 | <.0001 | 1.7 ± 0.9 |
MR grade † | 2 (0–4) | 0 (0–1) | <.0001 | 0 (0–1) |
MR VC (mm) | 3.1 ± 2.4 | 2.1 ± 1.9 | .04 | |
TR grade † | 2 (0.5–4) | 1 (0–2) | <.0001 | 1.5 (1–2) |
TR VC (mm) | 3.8 ± 2.5 | 2.6 ± 1.7 | .001 | |
PR grade † | 0 (0–1) | 0 (0–0) | .003 | 1 (0–1) |
LVAD parameters | ||||
Inflow cannula velocity systolic (cm/sec) | 76.2 ± 37.2 | |||
Inflow cannula velocity diastolic (cm/sec) | 24.6 ± 13.5 | |||
LVAD inflow systolic to diastolic ratio | 3.4 ± 1.9 | |||
Outflow cannula velocity (cm/sec) | 104.7 ± 37.1 | |||
Outflow cannula VTI (cm) | 40.7 ± 11.1 | |||
Calculated total output (L/min) | 5.8 ± 1.6 | |||
Calculated LVAD output (L/min) | 5.1 ± 1.2 |
∗ Before versus 3 months after LVAD implantation.
† Graded visually as described in the “Methods” section.
‡ Measured in the 50 patients with nonforeshortened four-chamber views.
Evaluation of indices of LV filling pressure showed significant prolongation of mitral inflow deceleration time of E-wave velocity, significant decrease in left atrial volume index, and a significant decrease in E/e′ ratio ( Table 2 ), all supportive of decreased LV filling pressure at 3 months after LVAD implantation. Interestingly, in those with left bundle branch block, the E/e′ ratio did not decrease and even increased slightly (5 ± 3% vs −38 ± 10%, P = .005).
The calculated RV pressure decreased significantly (−30.7 ± 26.2%, P < .0001), as did the estimated right atrial pressure ( Table 2 ). The quantitative estimation of RV function improved, RV end-diastolic and end-systolic areas decreased (32.0 ± 8.6 vs 29.3 ± 6.4 cm 2 , P = .0002, and 23.2 ± 7.2 vs 18.6 ± 5.6 cm 2 , P < .0001, respectively), RV fractional area change improved (27.9 ± 10% vs 37.4 ± 10.9%, P < .0001), and RIMP decreased significantly (0.69 ± 0.28 vs 0.34 ± 0.19, P < .0001) with LVAD therapy, suggesting improved RV function ( Figures 1 and 2 ). Interestingly, longitudinal contraction (lateral tricuspid annular motion velocity) did not change after LVAD implantation ( Table 2 ).
Follow-up measurements were available in 39 patients at 6 months after LVAD implantation ( Table 2 ). There were no significant differences in any of the echocardiographic variables compared with the measurements obtained at 3 months.
We assessed the effect of LVAD support on the severity of valvular regurgitation. LVAD therapy resulted in a significant decrease in the severity of mitral regurgitation (MR).
A common practice at the time of LVAD implantation consists of tricuspid valve repair or replacement, whenever TR is more than moderate. To better understand the effect of these interventions on regurgitation severity, we separated the patients into those who underwent or did not undergo tricuspid intervention during the initial LVAD surgery. TR severity improved significantly in patients in whom tricuspid valve intervention was performed but was unchanged in those without any tricuspid valve intervention ( Table 3 ). Our data show that TR did not improve in patients in whom the interventricular septum was deviated to the left ( Table 3 ).
Before implantation | After implantation | Before implantation | After implantation | Before implantation | After implantation | Before implantation | After implantation | |
---|---|---|---|---|---|---|---|---|
No TR surgery ( n = 40) | TR surgery ( n = 23) | IVS to left ( n = 5) | IVS to right ( n = 58) | |||||
TR VC (mm) | 2.8 ± 2.0 (NS) | 2.5 ± 1.6 (NS) | 5.5 ± 2.3 ∗ | 2.6 ± 1.9 ∗ | 4.7 ± 2.6 (NS) | 4.7 ± 1.9 (NS) | 3.7 ± 2.5 ‡ | 2.4 ± 1.6 ‡ |
No AR stitch ( n = 58) | AR stitch ( n = 5) | Aortic valve opening ( n = 23) | Aortic valve closed ( n = 40) | |||||
AR VC (mm) | 0.34 ± 0.82 ∗ | 2.0 ± 1.6 ∗ | 2.3 ± 1.8 (NS) | 1.2 ± 0.9 (NS) | 0.33 ± 0.6 † | 1.7 ± 1.4 † | 0.6 ± 1.2 ∗ | 2.1 ± 1.6 ∗ |
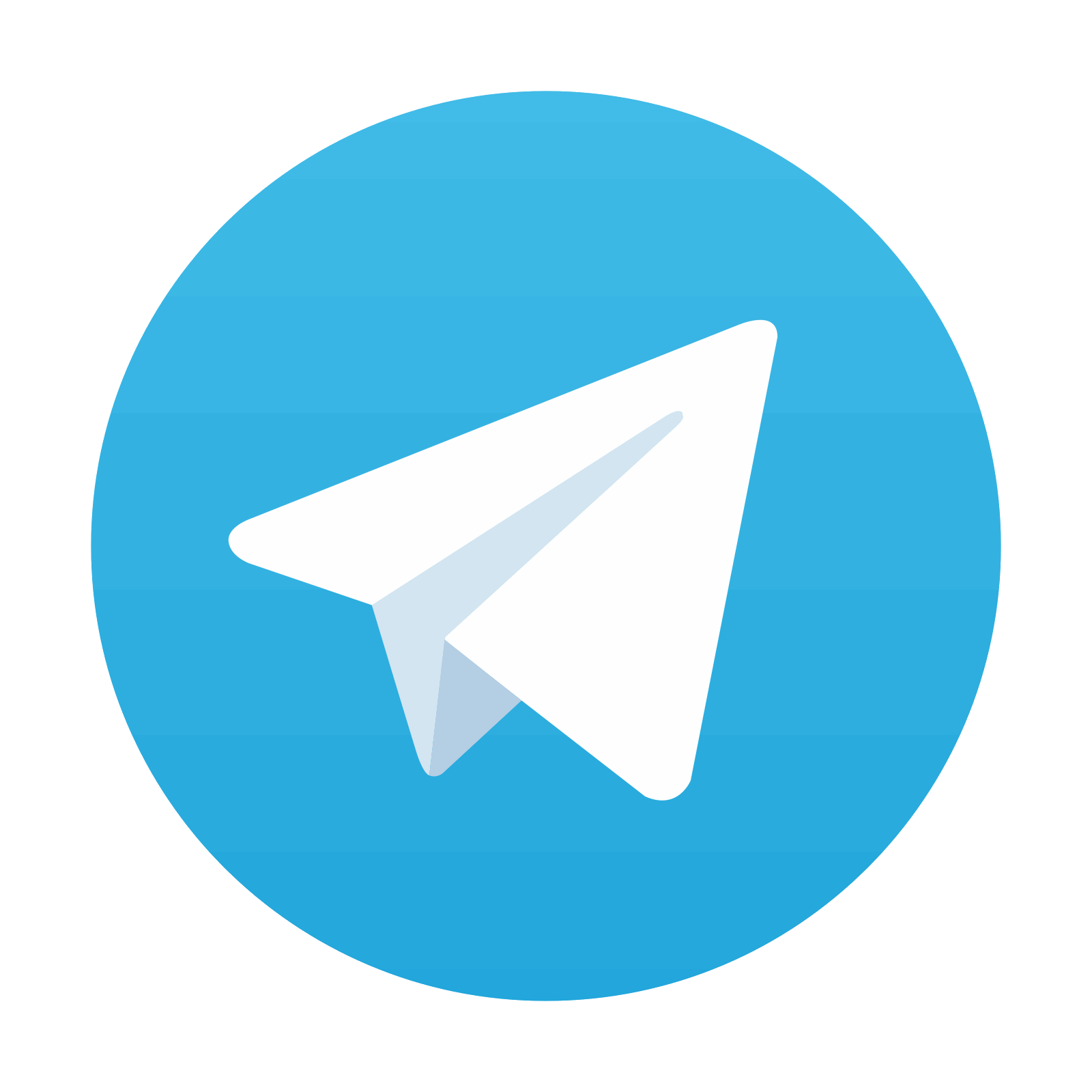
Stay updated, free articles. Join our Telegram channel

Full access? Get Clinical Tree
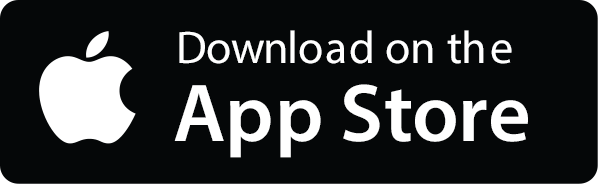
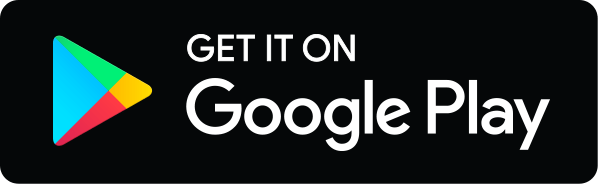
