33 Echocardiographic Evaluation of the Patient with Pulmonary Hypertension
Pulmonary Artery Pressure and Right Ventricular Afterload
Right Ventricular-Pulmonary Vascular Interaction
Pulmonary Hypertension as a Result of Chronic Respiratory Disease
Pulmonary Hypertension without Pulmonary Vascular Disease
Pulmonary Hypertension Related to Left Heart Disease (Pulmonary Venous Hypertension)
Pulmonary hypertension (PH) is defined as a mean pulmonary artery pressure (mPAP) of more than 25 mm Hg, which typically coincides with a pulmonary artery (PA) systolic pressure (PASP) of more than 40 mm Hg.1,2 An increased mPAP is the hemodynamic consequence of perturbations in pulmonary blood flow (cardiac output [CO]), pulmonary vascular resistance (PVR), and left atrium (LA) pressure, as expressed in the following equation:
Many clinical diagnoses can cause or contribute to PH, including left heart disease with pulmonary venous hypertension (PVH; World Health Organization [WHO] group II), chronic respiratory conditions typically associated with hypoxia (WHO group III), chronic thromboembolic PH (CTEPH; WHO group IV), and miscellaneous/multifactorial causes (WHO group V). In addition, PH may arise from severe pulmonary vasculopathy in the absence of chronic left heart disease, respiratory disease, or prior pulmonary emboli, which is referred to as pulmonary arterial hypertension (PAH; WHO group I).3 Given the highly diverse nature of PH, careful clinical and hemodynamic distinctions need to be made for the appropriate PH diagnosis. This is particularly true with discerning PAH from PVH because PH-specific therapy (i.e., prostacyclin analogues, endothelin receptor antagonists) intended for patients with PAH has either no effect or worsens the condition of a patient with left heart disease (Box 33-1).
Box 33-1 Pulmonary hypertension definitions and WHO Groups
The echocardiographic examination is central to the initial diagnosis of PH, and the serial assessment of PH. Fundamental to the diagnostic assessment is a variety of Doppler imaging–based pulmonary pressure estimations, as outlined in Chapter 32. However, many other ways exist to diagnose, infer, and assess PH and its hemodynamic causes aside from Doppler pressure estimation. Overreliance or exclusive focus on the Doppler pressure estimates and a failure to integrate other salient two-dimensional (2D) and Doppler findings into the overall echocardiographic interpretation greatly increase the risk of false-positive and false-negative diagnoses of PH. Conversely, a more integrated and pathophysiologic approach to the assessment not only enhances the diagnostic accuracy of PH but also allows for a much greater degree of pathophysiologic insight into an individual patient’s PH.
Pulmonary Artery Pressure and Right Ventricular Afterload
The level of pressure elevation does not correlate well with functional limitations or prognosis in PAH.4 Similarly, right ventricular (RV) size, septal position, and RV systolic function vary widely at the same level of mean PAP, depending on the specific hemodynamic cause.5 These observations relate to the fact that PAP is a relatively poor and incomplete measure of RV afterload. Afterload is the force that opposes RV ejection, and thus pulmonary blood flow, and is most completely described by PA input impedance. Input impedance is a composite measure of afterload that includes vascular resistance (PVR = mPAP − LA pressure/pulmonary blood flow), large PA stiffness, and the effects of these two factors on the magnitude and timing of reflected arterial waves arriving at the RV during systole.6
Thus PH can be present with normal pulmonary vessels when there is a high flow state or an elevated LA pressure, even when the pulmonary vessels themselves are normal. Conversely, abnormal changes in the pulmonary vessels themselves, or pulmonary vascular disease (PVD), may be present with only modest elevations in pulmonary pressure. Increased RV afterload is present in PH associated with PVD and is comparatively low in PH without PVD. PVR accounts for approximately 80% of total afterload in most clinical circumstances.6 Increased PAP does increase afterload indirectly via increased PA stiffening, but to a relatively small degree. For example, in a patient with a large atrial septal defect but normal PVR, the RV can eject 15 L of flow through the lung (Qp), despite severe PH, and maintain normal RV systolic function. In this same patient, however, if the PVR increases from 2 mm Hg/L/min to 8 mm Hg/L/min, the Qp falls dramatically and frank RV dysfunction manifests.
The distinction between PAP and RV afterload has considerable practical value in the context of an echocardiographic evaluation of a patient with known or suspected PH. RV afterload, not PAP, is what impairs RV function and leads to the stereotypical triad of RV enlargement, interventricular septal flattening, and RV systolic dysfunction in PH. With this in mind, the clinician should remain highly suspicious for PH related to PVD if RV dilation, dysfunction, and septal bowing are present, even if the Doppler image–estimated PASP is reported as normal. This point cannot be overemphasized. Fisher et al7 reported that more than 80% of subjects with severe PH that was related to an increased PVR and with PASP that was significantly underestimated with Doppler imaging possessed at least one of three features of the RV dysfunction triad on 2D examination. Conversely, if a patient has PH without significant rise in afterload (i.e., normal PVR), as in cases of high output PH or PH related to LA hypertension, RV structure and function remain comparatively normal, with the conspicuous absence of the RV dysfunction triad. Therefore, one can think of the RV as bearing witness to what is occurring within the pulmonary circulation. For these reasons, we consider PH associated with PVD (PH+PVD) separately from PH in the relative absence of PVD (PHøPVD).
Right Ventricular-Pulmonary Vascular Interaction
The RV and the pulmonary circulation function as a coupled unit. In health, a normal pulmonary vasculature couples to an appropriately thin-walled, distensible RV that generates large amounts of blood flow without a resulting high pressure. As PVD develops, the PVR increases and the large arteries stiffen. As a result, the (formerly appropriate) nonmuscular RV is typically incapable of completely matching or of coupling its contractile performance to its new afterload.8,9 This relative RV-PA uncoupling leads to a stereotypical triad of changes that occur at the level of the RV, including RV systolic dysfunction, increased size and altered shape of the RV, and varying degrees of systolic and diastolic bowing of the interventricular septum (Fig. 33-1). This triad of changes forms the basis of the echocardiographic diagnosis of PH+PVD. The degree to which these changes occur is contingent on the degree of RV-PA uncoupling. Thus, at a PVR of 5 Wood units, a patient with depressed RV contractility has far more uncoupling to the vascular load than a patient with an intrinsically normal RV coupled to the same afterload. As such, the RV dysfunction triad helps to adjudicate and assess the physiologic and clinical significance of any given degree of PVD. The greater the RV impairment, the greater the clinical and physiologic significance of the PH+PVD, no matter what the noninvasive (or invasive) pressure may be.
The RV outflow tract (RVOT) is a key anatomic and physiologic interface between the RV and pulmonary vasculature (Fig. 33-2, A). The pulsed-wave Doppler RV outflow velocity curve provides a wealth of physiologic information about RV and pulmonary vascular function. As such, analysis of the Doppler velocity signal represents perhaps the single most important aspect of the echocardiographic examination in a patient with known or suspected PH.
Early work from Kitabatake and associates10 showed that the time to peak velocity, or acceleration time (AcT), of the RV outflow velocity curve provides an estimate of mPAP (Fig. 33-2, C). An average AcT of 137 ± 24 ms was seen in subjects with a mean PA pressure of 19 mm Hg or less, whereas AcTs of 97 ± 20 ms and 65 ± 14 ms were seen in patients with a mPAP of 20 to 39 mm Hg and 40 mm Hg or more, respectively. This method is relatively easy to perform and highly reproducible; and unlike pressure estimates based on tricuspid regurgitation (TR) velocity, Doppler recordings from the RVOT are available in virtually all patients. The AcT can provide useful corroborative information that aids in the accuracy of PH diagnosis; for example, in the presence of borderline PH by TR jet velocity, an AcT of more than 100 ms suggests there is no PH, whereas an AcT of less than 70 ms greatly increases the likelihood of significant PH.
In addition to AcT measurement, visual inspection of the shape of the RV outflow velocity signal has also been be used to qualitatively differentiate varying levels of PH. Prior work has shown that a rounded velocity curve with no evidence of Doppler “notching” has been associated with normal PA pressures, and that patients with mild-moderate PH more often have a triangular, or dagger-shaped, curve (often with late-systolic notching) and those with severe PH typically manifest prominent midsystolic notching of the Doppler velocity signal.10 However, these same authors pointed out that only 53% of patients with PH evidenced a notched flow curve, which suggests that pressure is an associate cause but not the actual physiologic cause of this notching pattern.
The actual cause of Doppler notching is early arrival of reflected arterial waves from the pulmonary vasculature that leads to “real-time” impedance to RV ejection.11 This was initially described in the setting of acute and chronic pulmonary emboli, related to proximal vascular obstruction.12,13 However, Torbicki and colleagues12 showed that the time to midsystolic flow deceleration did not differ between patients with CTEPH and idiopathic PAH. Thus, analyses of the RV outflow velocity curve may be applied more broadly for detection of PVD.
Recent work was reported on a cohort of 79 patients (acute and chronic pulmonary embolic excluded) with undifferentiated PH and the hemodynamic differences seen on the basis of differences in the shape of the Doppler velocity curve.5 In contrast to prior work, this study showed that the absence of Doppler notching did not necessarily indicate a lack of PH. Instead, the lack of Doppler notching (see Fig. 33-2, C) was strongly associated with a relative lack of PVD; when PH was present in these patients, it was the result of an increased LA pressure and a relatively normal PVR (PHøPVD). In contrast, in the presence of increased PA stiffness and high PVR, reflected waves return to the RV during systole, impede RV ejection, and cause “notching” of the Doppler profile. A late systolic notch pattern typically coincides with moderate increases in the PVR (i.e., 3 to 6 Wood units; Fig. 33-3, A), most commonly seen in patients with milder degrees of PAH and in patients with chronic left heart disease or respiratory disease with concomitant PVD. A midsystolic notch pattern is especially abnormal and is the typical finding in patients with PAH and CTEPH. A midsystolic notch pattern is associated with an average PVR of more than 8 Wood units (Fig. 33-3, B); and a midsystolic notch pattern is 96% specific for a PVR of more than 5 Wood units. These findings indicate RV outflow velocity mid systolic notching is far more indicative of PVD (high PVR, low compliance) than high pulmonary pressure and help explain why notching is present in some, but not all, patients with PH.
In parallel with its associated RV afterload, the midsystolic notch pattern was associated with more advanced RV systolic dysfunction, lower tricuspid annular plane systolic excursion (TAPSE; Fig. 33-4, B), RV fractional area change, and the ratio of stroke volume index to RV end-diastolic area compared with normal patterns (no notching) and late-systolic notch groups. In contrast, patients with an elevated mPAP but normal pattern typically had either normal RV function or RV dysfunction associated with normal PVR and PA compliance. Taken together, these findings indicate that normal RV function without outflow notching suggests a relatively normal RV-PA interaction. In contrast, RV dysfunction in the presence of a notched outflow velocity curve strongly suggests an afterload-dependent mechanism, and RV dysfunction in the absence of RV outflow notching relates to primary afterload-independent RV dysfunction.
Pulmonary Vascular Disease
Pulmonary Arterial Hypertension
Pulmonary arterial hypertension is the prototype of PH+PVD and can be familial or idiopathic (formerly referred to as primary PH) or associated with various predisposing conditions, including connective tissue disease (i.e., scleroderma), congenital systemic to pulmonary shunts, HIV infection, toxin-mediated pulmonary vascular injury, and other conditions.3 Patients with PAH, by definition, have a mPAP of more than 25 mm Hg and an LA pressure of 15 mm Hg or less and universally have a PVR of more than 3 mm Hg/L/min (average PVR at index diagnosis, 8 to 10 mm Hg/L/min).3,14,15 In addition to these hemodynamic criteria, a diagnosis of PAH requires the exclusion of chronic left heart disease, respiratory disease, and chronic pulmonary emboli. All approved forms of PH-specific therapy are particularly intended for patients with PAH. Importantly, the response to PH therapies and patient prognosis are linked to the relative stage of the disease at the time therapy is implemented.4 This places a premium on early detection of PAH and, thus, on the importance of the echocardiographic examination, as this is one of the first noninvasive tests performed in the workup of a patient with suspected PAH. Echocardiography is a highly sensitive test for the detection of the physiology that underlies PAH, so long as the study is interpreted in an integrated manner, with due attention to RV size, shape, and function and other Doppler features that are nearly universally present in these patients. A normal or mildly elevated PASP Doppler estimate should not dissuade a suspected diagnosis of PAH when the other 2D and Doppler features of PAH are present.
In 1973, Goodman, Harrison, and Popp16 reported the salient 2D echocardiographic findings of idiopathic PAH. All patients had RV dilation, and 50% had an RV : left ventricle (LV) dimension ratio of 1 or more. Patients commonly showed paradoxical septal motion, where the interventricular septum moved away from the center of the LV cavity in systole (Fig. 33-5). Aside from being a common echocardiographic feature in PAH, the relative loss of inward septal motion can account for a mild loss of LV systolic function in PAH, and thus, a low-normal or mildly reduced LV function can occur in PAH on this basis. Bossone and co-workers17 published a larger observation series with idiopathic PAH and showed that 96% of patients had a Doppler-estimated pressure of more than 60 mm Hg at diagnosis; however, the overall correlation between Doppler and invasive pressure estimates was relatively low (r = 0.31). Ninety-eight percent of subjects had RV enlargement, and 75% of subjects had qualitative evidence of RV systolic dysfunction. Systolic flattening of the interventricular septum occurred in 90% of subjects. Moderate or severe TR occurred in 80% of subjects, whereas mild to moderate mitral regurgitation was present in only one patient. A small or moderate-sized pericardial effusion was also noted in about 16% of subjects.17

Figure 33-5 M-mode imaging from the parasternal position in a patient with severe pulmonary arterial hypertension.
In PAH, at least 70% of subjects manifest a transmitral Doppler E wave velocity to A wave velocity ratio (E : A ratio) of less than 1.0, consistent with grade I LV diastolic dysfunction. All subjects in this study had normal LA pressure with right heart catheterization. In fact, grade I diastolic dysfunction in the vast majority of cases indicates normal LA pressure, reflecting the necessary redistribution of LV filling into late diastole that must occur when LV relaxation is impaired in the context of a normal LV filling pressure.17,18 In clinical practice, the presence of any or all of the triad of findings of RV dysfunction, accompanied by grade I diastolic dysfunction, is a strong indicator of severe PH in the context of a normal LA pressure and high PVR. Arkles and associates5 recently showed that a notched RV outflow velocity curve was seen in 100% of patients with an incident diagnosis of PAH, which coincides with prior data that show that 94% of subjects with PAH have an AcT of the RV outflow velocity curve less than 100 ms.17 In our experience, the combination of a midsystolic notch RV outflow pattern with an E : A ratio of less than 1.0 is a highly sensitive and specific predictor of a markedly elevated PVR and a normal LA pressure.
Indices of right heart size, ventricular interdependence, and depressed RV function have been correlated with disease severity and patient outcome in patients with PAH.4,19 In addition, studies have consistently shown that the presence and size of a pericardial effusion predict poor outcome in patients with PAH.20 Larger effusion size correlates with greater degrees of exercise intolerance, right atrium (RA) dilation, greater interventricular septal displacement, and more severe TR than in patients without an effusion.19,21,22 The mechanism of the pericardial effusion in PAH most likely relates to slow progressive accumulation of intrapericardial fluid as a result of longstanding RA hypertension from decompensated right heart function. When the effusion is of moderate size or greater, the temptation is to intervene with percutaneous or surgical drainage. However, great caution should be exercised in this regard because drainage of pericardial effusions in patients with PAH has been associated with very poor outcomes, with death occurring within 12 to 24 hours of the procedure.23 Importantly, the pericardial effusion is a marker of, but not the cause of, the right heart decompensation in this context. Thus, before pericardial drainage is considered, a right heart catheterization should be performed; if markedly elevated PVR, high RA pressure, and depressed cardiac index are found, the effusion is highly likely to be the direct result of afterload-induced RV failure. In the authors’ experience, pericardial effusions in PAH almost invariably decrease in size or resolve over days or weeks as the RV failure is treated with pulmonary vasodilation therapy and diuresis.
Eysmann and colleagues24 showed that a peak pulmonic flow velocity of more than 60 cm/s was predictive of a drop in PVR of more than 30% on acute vasodilator testing; no patient with a peak velocity of less than 60 cm/sec had a condition that was vasoreactive. Likewise, decreased LV and LA area, increasing RV : LV area ratio, and the degree of leftward septal bowing are also associated with an increased risk of systemic hypotension in response to calcium channel blocker therapy, likely reflecting the inability to sufficiently recruit RV stroke volume to overcome the drop in systemic vascular resistance.25
Other studies have focused on more direct assessment of RV systolic function. Tei et al26 showed that the mean RV myocardial performance index (MPI) of patients with idiopathic PAH (0.83) was higher than in healthy controls (0.28) and that patients with an RV MPI of more than 0.83 had a less than 10% event-free survival rate at 5 years, versus more than 70% in those with an RV MPI of more than 0.83 (see Fig. 32-10).27 A prospective study in patients with idiopathic PAH and PAH associated with connective tissue disease showed that a TAPSE of less than 1.8 cm was associated with lower invasively derived stroke volume index, higher RA pressure, greater RV dilation, right to left heart disproportion, and septal bowing and a higher incidence rate of pericardial effusion. Two-year survival rate estimates were 88% and 50% for patients with a TAPSE of 1.8 cm or more and less than 1.8 cm, respectively.28 The prognostic value of TAPSE persisted after adjustment for several echocardiographic and invasive hemodynamic variables of known prognostic significance. The peak systolic tissue Doppler velocity is another practical measure of RV longitudinal function and is strongly correlated with TAPSE (see Fig. 32-10).
The typical changes in RV size, function, and septal position have been shown to improve in response to varying therapies. After 4 months of therapy with bosentan in patients with PAH, repeat echocardiographic examination showed improved RV MPI, reduced RV end-systolic area and RV : LV diastolic area ratio, improved early diastolic LV filling, and decreased pericardial effusion scores.29 Similarly, after 3 months of intravenous epoprostenol infusion, RV size decreased, as did peak TR velocity and the noninvasive PVR estimate.30
Preliminary data from the authors’ group have shown that after 6 months of PH-specific therapy, 78% of patients had increased TAPSE of more than 0.2 cm (mean, +0.5 cm), in association with significant improvements in 6-minute walk distance (median, +115 m), B-type natriuretic peptide levels (−210 pg/mL), and New York Heart Association functional class (−1.0 functional class). In those patients who reached a TAPSE of 2.0 cm or greater at 6 months, no deaths or right heart failure admissions were seen, as compared with two deaths and four right heart failure hospitalizations in those with a TAPSE of less than 2.0 cm on follow-up examination.31 The increase in TAPSE in response to therapy is consistent with recent data that show that in PAH, PH-specific therapy leads to relatively selective improvement in longitudinal RV shortening, which suggests that long-axis shortening of the RV may represent the most afterload-responsive element of RV systolic function.32
Acute Pulmonary Embolism
Acute pulmonary embolism and especially large proximal emboli lead to a sudden increase in the pulmonary vascular impedance without a marked rise in the PAP. This relates to the fact that the normal RV is typically incapable of generating a sufficient stroke volume to maintain a PASP of more than 60 mm Hg (mean pressure, 40 mm Hg) when suddenly uncoupled from a normal pulmonary vascular bed.33,34 As a result, RV failure and cardiogenic shock may ensue despite a mean PA pressure between 20 and 40 mm Hg.35 It follows that the degree of RV dysfunction predicts the extent of perfusion defects with ventilation/perfusion scan.36 In contrast, small subsegmental pulmonary emboli that are not associated with a marked rise in PA impedance do not lead to abnormal echocardiographic findings, highlighting the fact that echocardiography cannot rule out an acute pulmonary embolism.37 The relative degree of PH helps establish the chronicity of the embolic event, as the average transtricuspid flow velocity in acute proximal pulmonary emboli (3.0 m/s) is significantly lower than in patients with subacute pulmonary embolism (4.2 m/s).34 In contrast, patients with acute pulmonary embolism with a PASP of more than 50 mm Hg at the time of diagnosis are at increased risk of persistent PH at 1-year follow-up.38
From a physiologic viewpoint, acute pulmonary embolism is an important and often dramatic clinical example that underscores the concept that PAP is a poor measure of RV afterload. RV dilation is almost universally present in the setting of a large pulmonary embolism, as the normally compliant RV rapidly distends in response to the increased vascular load. However, unlike in PAH or other forms of chronic PVD, RV hypertrophy is conspicuously absent. Another common finding in acute pulmonary embolism is McConnell’s sign (Fig. 33-6), which is visually appreciated as RV dysfunction along the RV base and mid segments, with a hinge point or “buckling” of the RV free wall near the RV apex.39 This relative sparing of RV apical wall motion likely is caused by tethering of the RV apex by a normal or hyperdynamic LV across the interventricular septum. Other studies have shown that the RV ejection fraction, RV stroke volume measured with pulsed Doppler imaging, and TAPSE are reduced in the setting of an acute pulmonary embolism.40 A characteristic Doppler finding in acute pulmonary embolism is the “60/60 sign,” which refers to a markedly shortened AcT (less than 60 ms) in the RV outflow velocity curve, because of the increased PA impedence, combined with a PASP of less than 60 mm Hg.41 Similarly, midsystolic notching of RV outflow is a common finding in acute pulmonary embolism, relating to early arrival of reflected pressure waves from proximal vascular obstruction.
Another important finding to look for on the initial examination is right-sided heart thrombi and thrombi-in-transit, which complicate approximately 4% of cases with acute pulmonary emboli (Fig. 33-7).42 Thrombi may be visualized within the RA and ventricle and occasionally are seen straddling the interatrial septum. Right heart thrombi are a high-risk feature, typically associated with prior massive pulmonary embolism, severe RV dysfunction, and high in-hospital mortality.42 In such cases, thrombolysis or catheter-based versus surgical embolectomy is often warranted.42,43
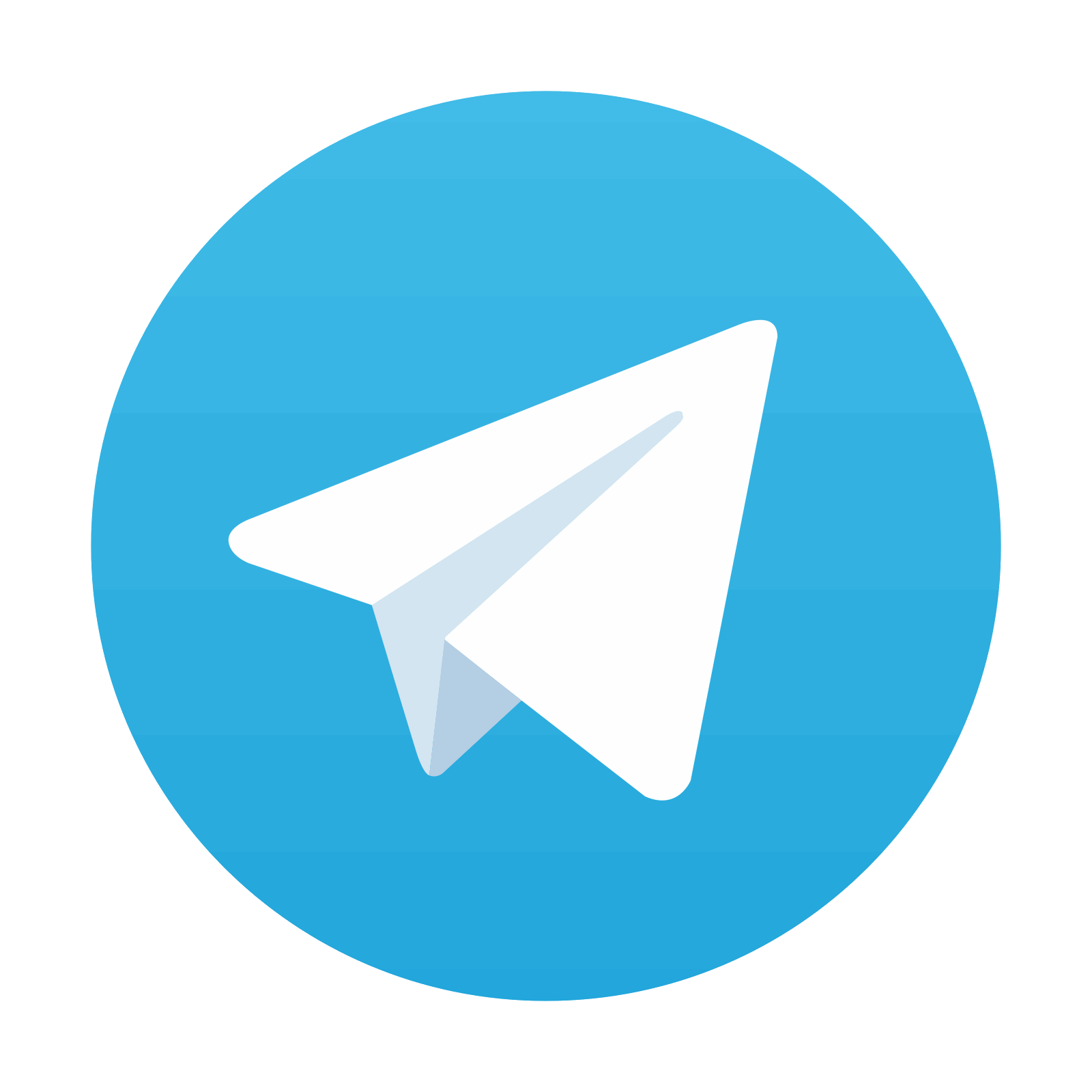
Stay updated, free articles. Join our Telegram channel

Full access? Get Clinical Tree
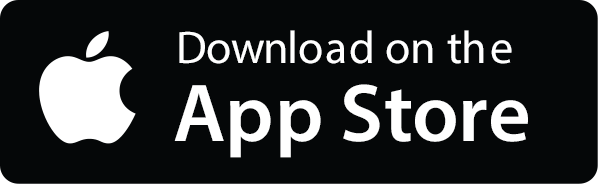
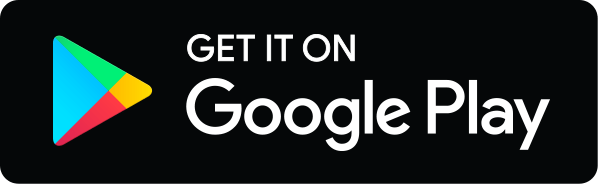