The typical appearance of cardiac amyloidosis using standard echocardiographic techniques is usually a late finding only in patients with relatively advanced stages of the disease. Early noninvasive identification of cardiac amyloidosis is of growing clinical importance. Newer echocardiographic techniques, including tissue Doppler imaging and deformation imaging (strain rate imaging and 2-dimensional speckle tracking), are powerful tools for quantifying regional myocardial motion and deformation. Using these advanced techniques, early functional impairment in cardiac amyloidosis may be detectable when the results of standard echocardiography are still normal or inconclusive. This review provides a comprehensive overview of the different echocardiographic approaches for the assessment of systolic and diastolic function in patients with cardiac amyloidosis. Special attention is paid to regional myocardial function assessed by tissue Doppler imaging, strain rate imaging, and 2-dimensional speckle-tracking imaging.
In this review, we comprehensively evaluate the echocardiographic approach in the assessment of systolic and diastolic function in patients with cardiac amyloidosis (CA). Special attention is paid to regional myocardial function assessed by tissue Doppler imaging (TDI), strain rate imaging (SRI), and 2-dimensional (2D) speckle-tracking imaging (STI).
Standard Echocardiographic Findings
Conventional M-mode and 2D echocardiographic features associated with CA include concentric left ventricular (LV) and right ventricular (RV) thickening, normal LV cavity size, dilated atria, and pericardial effusion. The myocardial texture often features a distinct “granular sparkling” for diagnosing CA with sensitivity of 87% and specificity of 81% ( Figure 1 ). The LV ejection fraction and fractional shortening in CA remain normal or nearly normal up to the late stage of the disease. Diastolic dysfunction appears early in CA and can be evaluated using standard and Doppler echocardiography. However, most studies of the role of conventional echocardiography in amyloidosis included patients in advanced stages, but it is difficult by this technique to detect early cardiac involvement. With the help of newer echocardiographic modalities such as TDI and SRI, early diagnosis might be feasible by the detection of subclinical myocardial dysfunction in patients with CA.
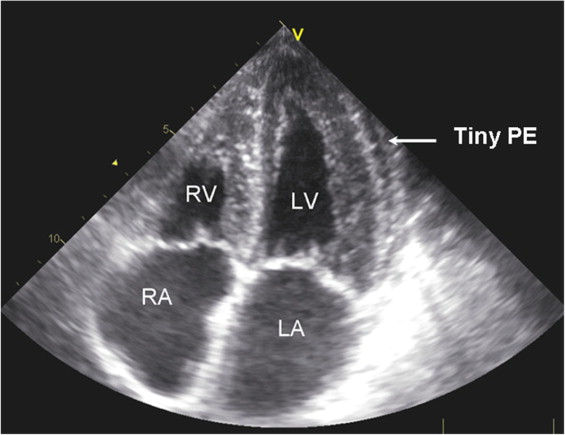
How to Assess Regional Myocardial Function
TDI provides quantification of regional myocardial function by the assessment of myocardial segmental motion and deformation. Tissue Doppler velocity can be acquired through pulsed Doppler, M-mode Doppler, or 2D color Doppler. The main limitation of tissue velocity data is the influence of cardiac overall motion and adjacent myocardial tethering. Deformation imaging can overcome these issues. Myocardial deformation can be demonstrated by strain rate and strain parameters derived from TDI as well as 2D STI. Strain rate has been confirmed to be more related to myocardial contractility, while strain is related to overall stroke volume. Motion and deformation of regional myocardium consist of 3 components: longitudinal, radial, and circumferential ( Figure 2 ). The use of tissue Doppler–derived SRI has remained limited because of angle dependency, substantial intraobserver and interobserver variability, and noise interference. STI is a promising new echocardiographic technique based on frame-to-frame tissue tracking from 2D grayscale images. Segmental motion and deformation can be analyzed quantitatively using STI, which is much less angle dependent than conventional TDI techniques. Currently, STI has been proposed to be applied in clinical routine echocardiography rather than TDI, because of more rapid tracking and semiautomatic segmentation. Related key concepts in respect of ultrasonic techniques are listed in Table 1 .
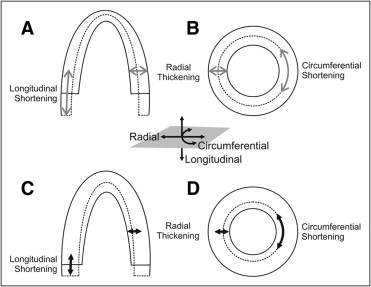
Basic Concept | |
---|---|
Tissue Doppler | Applying a low-pass filter (high-velocity rejection) to acquire high-amplitude and low-velocity signals of the myocardial tissue for the determination of ventricular motion (tissue velocity and displacement) |
Deformation | Referring changes in the shape or size of the myocardium, consisting of longitudinal shortening and lengthening, circumferential shortening and lengthening, and radial thickening and thinning; there are interactions among these 3 directional deformations, because myocardium is not compressible |
Strain | Reflecting the percentage change in myocardial length in comparison to its original length (strain = [L − L 0 ]/L 0 ) |
Strain rate | Presenting the speed at which deformation occurs, demonstrated as velocity gradient (strain rate = strain/Δt) |
How to Assess Regional Myocardial Function
TDI provides quantification of regional myocardial function by the assessment of myocardial segmental motion and deformation. Tissue Doppler velocity can be acquired through pulsed Doppler, M-mode Doppler, or 2D color Doppler. The main limitation of tissue velocity data is the influence of cardiac overall motion and adjacent myocardial tethering. Deformation imaging can overcome these issues. Myocardial deformation can be demonstrated by strain rate and strain parameters derived from TDI as well as 2D STI. Strain rate has been confirmed to be more related to myocardial contractility, while strain is related to overall stroke volume. Motion and deformation of regional myocardium consist of 3 components: longitudinal, radial, and circumferential ( Figure 2 ). The use of tissue Doppler–derived SRI has remained limited because of angle dependency, substantial intraobserver and interobserver variability, and noise interference. STI is a promising new echocardiographic technique based on frame-to-frame tissue tracking from 2D grayscale images. Segmental motion and deformation can be analyzed quantitatively using STI, which is much less angle dependent than conventional TDI techniques. Currently, STI has been proposed to be applied in clinical routine echocardiography rather than TDI, because of more rapid tracking and semiautomatic segmentation. Related key concepts in respect of ultrasonic techniques are listed in Table 1 .
Basic Concept | |
---|---|
Tissue Doppler | Applying a low-pass filter (high-velocity rejection) to acquire high-amplitude and low-velocity signals of the myocardial tissue for the determination of ventricular motion (tissue velocity and displacement) |
Deformation | Referring changes in the shape or size of the myocardium, consisting of longitudinal shortening and lengthening, circumferential shortening and lengthening, and radial thickening and thinning; there are interactions among these 3 directional deformations, because myocardium is not compressible |
Strain | Reflecting the percentage change in myocardial length in comparison to its original length (strain = [L − L 0 ]/L 0 ) |
Strain rate | Presenting the speed at which deformation occurs, demonstrated as velocity gradient (strain rate = strain/Δt) |
Left Ventricular Systolic Dysfunction
LV global systolic function remains normal until the late stage of the disease in patients with CA. However, despite a normal LV ejection fraction and fractional shortening, longitudinal systolic function may be altered very early during disease progression. Thus, newer imaging techniques for the assessment of longitudinal function, such as TDI, SRI, and STI, have been suggested for the echocardiographic evaluation of patients with CA. The recent progress in evaluating regional myocardial function using motion-based (TDI) and deformation-based (SRI and STI) techniques is summarized in the following discussion to characterize typical patterns in patients with CA ( Table 2 ).
Parameter | Subclinical Stage | Typical Stage | End Stage |
---|---|---|---|
Systolic function | |||
SEP | |||
IVSD | — | ↑ | ↑↑ |
EF | — | — | ↓ |
FS | — | — | ↓ |
MAD | — | ↓ | ↓↓ |
TAPSE | — | — | ↓ |
TDI | |||
Systolic velocity | |||
Apical level | — | — | — |
Middle level | — | — | ↓↓ |
Basal level | — | ↓ | ↓↓ |
SRI | |||
Longitudinal deformation | |||
Apical level | — | — | ↓ |
Middle level | ↓ | ↓↓ | ↓↓ |
Basal level | ↓ | ↓↓ | ↓↓ |
Radial deformation | — | — | ↓ |
2D STI | |||
Longitudinal deformation | |||
Apical level | — | — | ↓ |
Middle level | ↓ | ↓↓ | ↓↓ |
Basal level | ↓ | ↓↓ | ↓↓ |
Global deformation | ↓ | ↓↓ | ↓↓ |
Radial deformation | — | ↓ | ↓↓ |
Circumferential deformation | — | ↓ | ↓↓ |
Diastolic function | |||
LV filling pattern | + | ++/+++ | +++ |
Peak early diastolic velocity by pulsed-wave Doppler | — | ↓ | ↓↓ |
Peak early diastolic strain rate | — | ↓ | ↓↓ |
Global ventricular function | |||
Tei index | ↑ | ↑↑ | ↑↑ |
Tissue Doppler imaging
As in normal controls, tissue velocities in patients with CA present a similar base-to-apex gradient in all LV walls, with the highest tissue velocities at the base of each wall and progressive decreases of these velocities as the sample volume is moved toward the apex. Despite this gradient, peak systolic tissue velocities at the base and middle ventricle were significantly lower in advanced CA patients (presenting with congestive heart failure) compared to early asymptomatic patients. In contrast, peak systolic tissue velocity did not differ between the non-CA and non–congestive heart failure groups in these studies.
Strain rate and strain derived from TDI
In patients with advanced primary amyloidosis, studies of myocardial deformation quantified by TDI-derived SRI showed that systolic strain rate and strain at the basal septal segments were significantly decreased. When focusing on different directions of systolic heart function, it could be shown that although radial function was still within the normal range, longitudinal strain rate and strain were markedly reduced ( Figure 3 ). Moreover, serial investigations revealed that systolic strain rate and strain at the base and middle ventricle were significantly decreased also in asymptomatic patients with increased wall thickness. Other investigators found that systolic strain rate and strain in the basal and middle LV segments in patients with advanced CA and hypertrophy were significantly lower compared to patients with CA with normal wall thickness. Furthermore, peak longitudinal systolic strain rate and strain were decreased in all 16 LV segments of patients with CA presenting any abnormality on standard echocardiography. Interestingly, radial posterior systolic strain rate and strain were not impaired in these CA patients with LV hypertrophy. These observations support the notion that impairment of longitudinal systolic function plays a major role in CA and might be present even before congestive heart failure occurs and sometimes even in subjects with normal results on standard echocardiography. Furthermore, multivariate survival analysis identified independent predictors of clinical outcome in patients with amyloidosis, and longitudinal peak systolic basal septal strain ≤−7.5% was associated with adverse outcomes.
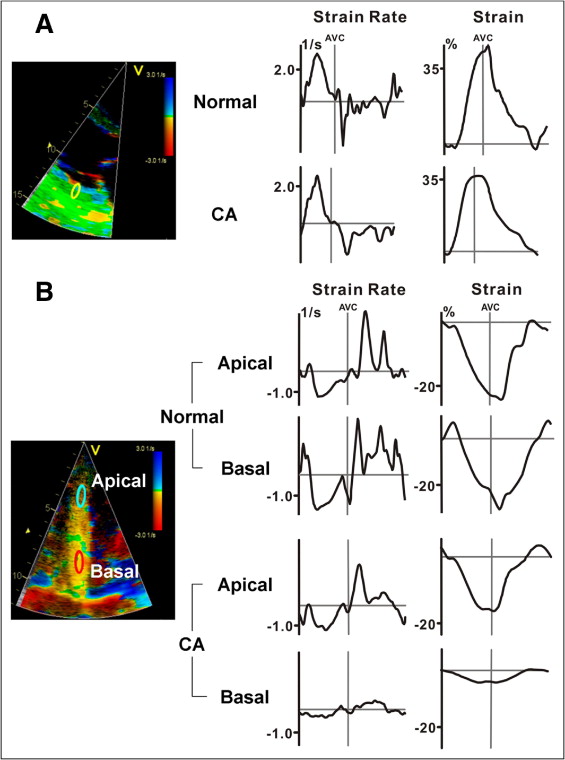
Strain rate and strain derived from 2D STI
There are only few published studies reporting on functional abnormalities in patients with CA by STI ( Figure 4 ). Sun et al reported that global longitudinal deformation detected by STI was significantly lower in patients with CA compared to healthy controls but also to subjects with LV hypertrophy caused by hypertrophic cardiomyopathy or hypertensive heart disease. In patients with CA, some segments displayed delayed peak strain, suggesting failure of the synchronized contraction pattern that is characteristic for the pathology of these patients.
Furthermore, Kusunose et al investigated 10 patients with biopsy-proved CA using 2D STI. Peak systolic circumferential and radial strain rate and strain were calculated in the inner (endocardial half), outer (epicardial half), and transmural myocardial walls from short-axis images of the middle left ventricle. Circumferential and radial strain and strain rate of inner, outer, and transmural myocardium were all lower compared to patients with hypertension. Furthermore, segments positive for late enhancement on cardiac magnetic resonance tomography (suggesting myocardial fibrosis) showed significantly reduced radial strain and strain rate especially of the inner layer. This might be important, because these data suggest that myocardial fibrosis seems to be responsible for the markedly reduced longitudinal but also the mildly impaired radial function.
Pathomechanisms for systolic dysfunction
The underlying pathomechanisms of systolic dysfunction associated with CA remain widely unknown. Brenner et al reported that human light-chain proteins specifically alter the cellular redox state in isolated cardiomyocytes, resulting in direct impairment of cardiomyocyte contractile function and calcium handling. These direct toxic effects of the proteins induce myocardial hypertrophy, which additionally leads to impaired LV function. As the disease progresses, more amyloid deposits lead to atrophy and apoptosis of cardiomyocytes and a remodeling toward collagen formation and subsequent fibrosis. In these advanced disease stages, the impaired systolic and diastolic function is responsible for the severe symptoms with congestive heart failure.
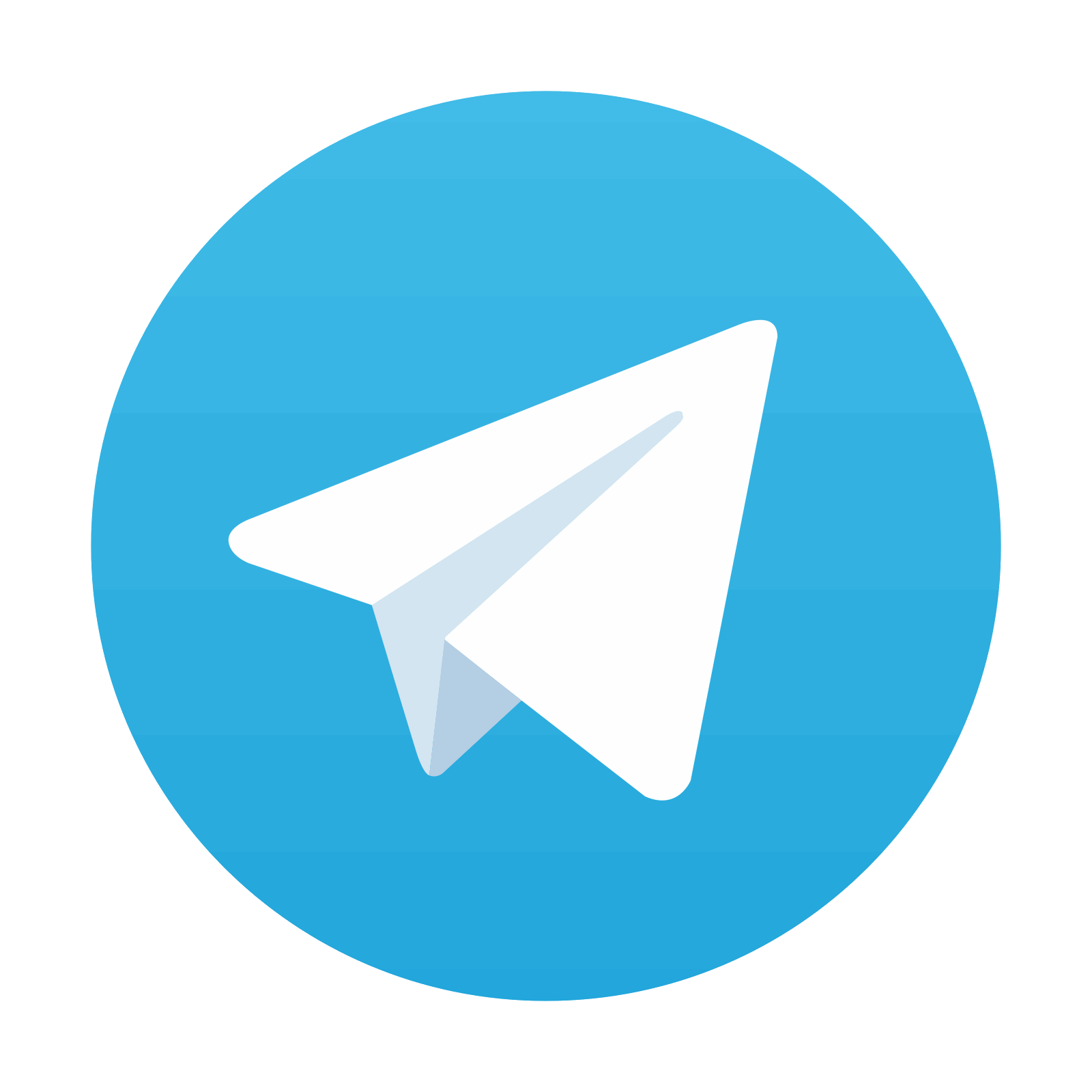
Stay updated, free articles. Join our Telegram channel

Full access? Get Clinical Tree
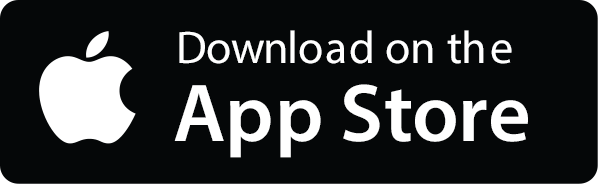
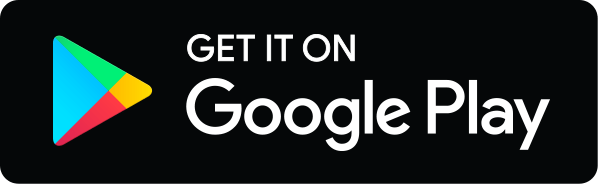