Background
Right ventricular (RV) failure is a key determinant of mortality in children with pulmonary arterial hypertension (PAH). RV stroke work (RVSW) can be estimated as the product of RV systolic pressure and stroke volume. The authors have shown that RVSW predicts adverse outcomes in this population when derived from hemodynamic data; noninvasive assessment of RVSW may be advantageous but has not been assessed. There are few data validating noninvasive versus invasive measurements in children with PAH. The aim of this study was to compare echocardiographically derived RVSW with RVSW determined from hemodynamic data.
Methods
This was a retrospective study, including subjects with idiopathic PAH and minor or repaired congenital heart disease. Forty-nine subjects were included, in whom cardiac catheterization and echocardiography were performed within 1 month. Fourteen additional patients were included in a separate cohort, in whom catheterization and echocardiography were performed simultaneously. Catheterization-derived RVSW was calculated as RV systolic pressure × (cardiac output/heart rate). Echocardiographically derived RVSW was calculated as 4 × (peak tricuspid regurgitant jet velocity) 2 × (pulmonary valve area × velocity-time integral). Statistics included the intraclass correlation coefficient and Bland-Altman analysis.
Results
Echocardiographically derived RVSW was linearly correlated with invasively derived RVSW ( r = 0.74, P < .0001, intraclass correlation coefficient = 0.76). Bland-Altman analysis showed adequate agreement. Echocardiographically derived RV work was related to indexed pulmonary vascular resistance ( r = 0.43, P = .002), tricuspid annular plane systolic excursion ( r = 0.41, P = .004), and RV wall thickness ( r = 0.62, P < .0001).
Conclusions
The authors demonstrate that RV work, a potential novel index of RV function, can be estimated noninvasively and is related to pulmonary hemodynamics and other indices of RV performance.
Children with pulmonary arterial hypertension (PAH) are at risk for right ventricular (RV) failure, the primary cause of morbidity and mortality in this population, because of increased afterload. Traditionally, RV afterload has been thought of as pulmonary vascular resistance (PVR) indexed to body surface area. However, PVR does not adequately account for all components of pulmonary hemodynamics, nor does it quantify the degree to which the right ventricle has compensated to the high afterload. This may be the reason that associations between PVR and clinical outcomes are relatively weak. In general, PVR represents the static component of RV afterload, while pulmonary artery capacitance accounts for the pulsatile component. Capacitance is computed as RV stroke volume divided by pulse pressure and is a predictor of clinical outcomes in both adults and children with PAH. Other echocardiographic indices of RV systolic performance, such as tricuspid annular plane systolic excursion (TAPSE) and the myocardial performance index (MPI), have shown promise as predictors of outcome in adults with PAH. Prior studies have shown that children with PAH have significantly lower TAPSE and higher (more abnormal) MPI.
RV stroke work (RVSW), which incorporates stroke volume and systolic pressure, may be more representative of both static and dynamic workload and therefore may be a determinant of RV failure and clinical outcomes. RVSW can be estimated as the product of peak RV systolic pressure and stroke volume and therefore is related to both resistance and capacitance. Moreover, RVSW reflects the area bounded by the pressure-volume loop and so is dependent on contractility, afterload, and ventriculoarterial coupling. We have recently shown that estimated RVSW, calculated on the basis of hemodynamic data obtained at cardiac catheterization, is predictive of outcomes in children with PAH. Cardiac catheterization, though an essential tool in the evaluation of patients with PAH, requires deep sedation and carries a quantifiable risk to the patient.
Therefore, noninvasive assessment of RVSW using echocardiography may be useful, but it has not been evaluated in this population. We hypothesized that echocardiography can be used to derive RVSW. The aim of this study was to compare RVSW determined by invasively-derived data during cardiac catheterization and noninvasively derived data using echocardiography. The subjects in the retrospective cohort of this study were previously included in the analysis for our report exploring the relationship between RVSW derived from invasive hemodynamic data and clinical outcomes ; however, the feasibility and accuracy of the noninvasive derivation of RVSW were not assessed. Similarly, the relationship of noninvasively derived RVSW with other echocardiographic indices of ventricular performance is of interest and warrants further investigation.
Methods
Subjects
Retrospective Cohort
A cross-sectional retrospective chart review was conducted for the retrospective comparison of catheterization-derived and echocardiographically derived RVSW. Subjects in the Children’s Hospital Colorado PAH database were included in the study if they met diagnostic criteria for PAH (mean pulmonary artery pressure > 25 mm Hg, pulmonary capillary wedge pressure < 10 mm Hg, and PVR > 3 Wood units). Exclusion criteria include age > 22 years and a tricuspid regurgitant jet that was inadequate for the purposes of estimating RV pressure. Subjects in whom PAH was related to pulmonary venous or left-heart obstructive lesions were also excluded. Subjects were included in the present analysis on the basis of the availability of both cardiac catheterization data and echocardiographic data within a period of 30 days. Demographic and clinical data were obtained from medical records, including cardiac catheterization and echocardiographic reports. All hemodynamic data were collected from clinically indicated, standard-of-care studies.
Prospective Cohort
In a smaller group of patients, invasive hemodynamic and echocardiographic data were acquired simultaneously. These patients were undergoing clinically indicated cardiac catheterization for the evaluation of PAH and were prospectively enrolled into a study for the evaluation of ventricular function in children with PAH between May 1, 2012, and October 1, 2013. Studies were done under general anesthesia. Exclusion criteria were similar to the retrospective cohort, including an inadequate tricuspid regurgitant jet spectral Doppler envelope and pulmonary venous or left-heart obstructive lesions. Additionally, single-ventricle physiology, the presence of a pacemaker, cardiomyopathy, pulmonary artery stenosis, and systemic hypertension were grounds for exclusion. The calculation of RVSW was done retrospectively on the prospectively collected data set.
Cardiac Catheterization
Right-heart catheterization was performed during respiration of 21% oxygen and under either general anesthesia or conscious sedation, on the basis of clinician preference. A fluid-filled, end-hole catheter was used to obtain right atrial, RV, and pulmonary artery pressures. Cardiac output was measured by thermodilution in those without intracardiac shunts and by oximetry (Fick method) when felt to be appropriate by the interventional cardiologist. PVR was calculated by dividing the transpulmonary gradient (mean pulmonary artery pressure − pulmonary capillary wedge pressure) by pulmonary blood flow; PVR was then indexed to body surface area (Wood units). Cardiac catheterization reports were reviewed to obtain anthropometric data, right-heart pressures, and cardiac output.
Echocardiography
Echocardiography was performed using a Vivid 7 (GE Healthcare, Milwaukee, WI) or iE33 (Philips Medical Systems, Andover, MA) platform, with probe frequencies appropriate for body habitus. Studies were performed with subjects breathing room air. Echocardiographic reports were reviewed to obtain anthropometric data, and echocardiographic images were reviewed offline, using commercially available software (Cardiovascular Review Station version 2.14.03; AGFA Healthcare, Mortsel, Belgium). Spectral Doppler patterns of tricuspid regurgitation were analyzed to determine the peak velocity of the regurgitant jet, when present. The duration of the regurgitant jet was also noted; the RV ejection time was obtained from the spectral Doppler pattern of interrogation of the RV outflow tract from the parasternal window, for the purposes of calculating MPI. MPI was determined by obtaining the sum of the isovolumic periods and dividing by RV ejection time. TAPSE was measured from the apical window using an M-mode capture of the tricuspid annulus. RV wall thickness was measured in diastole in a parasternal short-axis view, taking care to avoid including any trabeculations in the assessment.
Calculation of RVSW
For estimation on the basis of invasive data, stroke volume (milliliters per beat) was determined by dividing pulmonary blood flow (milliliters per minute) by heart rate (beats per minute). RVSW was calculated by multiplying peak RV pressure by RV stroke volume. The estimated right atrial pressure was not added to RV pressure, because this did not significantly alter the calculation of stroke work.
Using noninvasive data, stroke volume was calculated from the pulmonary valve area multiplied by the velocity-time integral (VTI) of flow at the same location. More specifically, the diameter of the pulmonary valve annulus was measured on a parasternal short axis image during systole, and the cross-sectional area of the pulmonary valve was calculated (π · r 2 ). After pulsed-wave Doppler interrogation at the level of the pulmonary valve, the VTI was determined by measuring the area under the curve of the spectral Doppler pattern. RV systolic pressure was estimated by measuring the velocity of the tricuspid regurgitant jet and using the modified Bernoulli equation to arrive at peak RV pressure ( P = 4 V 2 ). An assumed right atrial pressure was also not added, for the reasons described previously. Finally, noninvasively derived RVSW was determined by multiplying peak RV systolic pressure by RV stroke volume.
Statistical Analysis
Continuous data are presented as median (interquartile range), and categorical data are presented as proportion (percentage). The Shapiro-Wilk test was used to determine normality of the distributions. Comparison of median values was performed using the Wilcoxon rank sum test. Correlation was performed by calculating Spearman and intraclass correlation coefficients. P values < .05 were determined to indicate statistical significance. Interobserver and intraobserver variability was assessed using intraclass correlation and coefficients of variation. Stata version 12 (StataCorp LP, College Station, TX), was used to perform the analysis.
Results
In the retrospective cohort, invasive and noninvasive estimates of RVSW were available for 49 of 135 patients, with a median age of 9.5 years (interquartile range, 6.0–15.7 years). Demographic and baseline data are presented in Table 1 . The median number of days between catheterization and echocardiography in the retrospective cohort was 1 (interquartile range, 1–23 days). Baseline data for the prospective cohort are presented in the second column of Table 1 .
Variable | Retrospective | Prospective | ||
---|---|---|---|---|
Total | Median (IQR) or n (%) | Total | Median (IQR) or n (%) | |
Age (y) | 50 | 9.5 (6.0–15.7) | 14 | 13.5 (12–16) |
Diagnosis | ||||
Idiopathic PAH | 21 | 43% | 5 | 37% |
Repaired CHD | 13 | 26% | 0 | 0% |
Minor CHD | 11 | 23% | 2 | 14% |
Unrepaired CHD | 4 | 8% | 4 | 28% |
Other | 0 | 0% | 3 | 21% |
Male gender | 50 | 40% | 10 | 71% |
BSA (m 2 ) | 50 | 1.08 (0.8–1.6) | 14 | 1.3 (1.2–1.6) |
Anti–pulmonary hypertensive medications | ||||
Phosphodiesterase-5 inhibitor | 31 | 62% | 11 | 78% |
Prostacyclin | 20 | 40% | 7 | 50% |
Home oxygen | 20 | 40% | 3 | 21% |
Calcium channel blocker | 14 | 28% | 4 | 28% |
Endothelin receptor antagonist | 12 | 24% | 7 | 50% |
Days between catheterization and echocardiography | 50 | 1 (1–23) | 14 | 0 (0–0) |
Heart rate (beats/min) | 49 | 88 (74–100) | 14 | 73 (65–82) |
Mean RA pressure (mm Hg) | 47 | 6 (4–7) | 14 | 7 (5–8) |
Maximum PA pressure (mm Hg) | 50 | 50.5 (40–75) | 14 | 52 (40–89) |
Minimum PA pressure (mm Hg) | 50 | 21 (12–37) | 14 | 22 (15–45) |
Mean PA pressure (mmHg) | 50 | 33.5 (24–54) | 14 | 30.5 (26–60) |
Pulmonary capillary wedge pressure (mm Hg) | 50 | 8 (6–10) | 14 | 8.7 (6–10) |
Mean aortic pressure (mm Hg) | 49 | 61 (55–70) | 14 | 60.5 (56–67) |
Cardiac index (L/min/m 2 ) | 50 | 3.8 (3.1–4.7) | 14 | 3.5 (3.2–3.7) |
PVR (WU) | 50 | 6.5 (3.7–11.6) | 14 | 6.2 (3.6–14.1) |
Systemic vascular resistance (WU) | 49 | 13.7 (11.5–18.5) | 14 | 15.9 (13.4–17.4) |
Indexed pulmonary blood flow (L/min/m 2 ) | 50 | 3.8 (3.0–4.8) | 14 | 3.4 (3.2–3.7) |
Catheterization-derived indexed RVSW (mm Hg · mL/m 2 ) | 49 | 2,640 (1,767.7–3,312.5) | 13 | 2,446.8 (2,200–3,444.6) |
RV fractional area change (%) | 49 | 43 (31–51) | 14 | 34 (20–38) |
Tricuspid valve systolic tissue velocity (s′) | 31 | 12 (11–14) | 14 | 11 (10–12) |
Tricuspid valve early diastolic tissue velocity (e′) | 31 | 13 (10–17) | 14 | 12.5 (11–15) |
Tricuspid valve late diastolic tissue velocity (a′) | 31 | 9 (8–12) | 14 | 8 (5–11) |
Echocardiographically derived indexed RVSW (mm Hg · mL/m 2 ) | 50 | 2,612.1 (1,657.1–4,275.4) | 14 | 2,412.2 (1,580.2–3,568.2) |
For the retrospective cohort, RVSW derived from echocardiographic data is compared with RVSW derived from data obtained on cardiac catheterization in Figures 1A to 1C . Figure 1 A is a box plot showing that the distribution was similar by both modalities, and the median RVSW was not significantly different ( P = .87). In Figure 1 B, a statistically significant monotonic increasing association is shown, with a Spearman correlation coefficient of 0.74 ( P < .0001); the intraclass correlation coefficient was 0.76, which is in the range of good agreement. The Bland-Altman method was also applied ( Figure 1 C), and the two methods of deriving RVSW showed good agreement; notably, there was better agreement at lower mean RVSW.

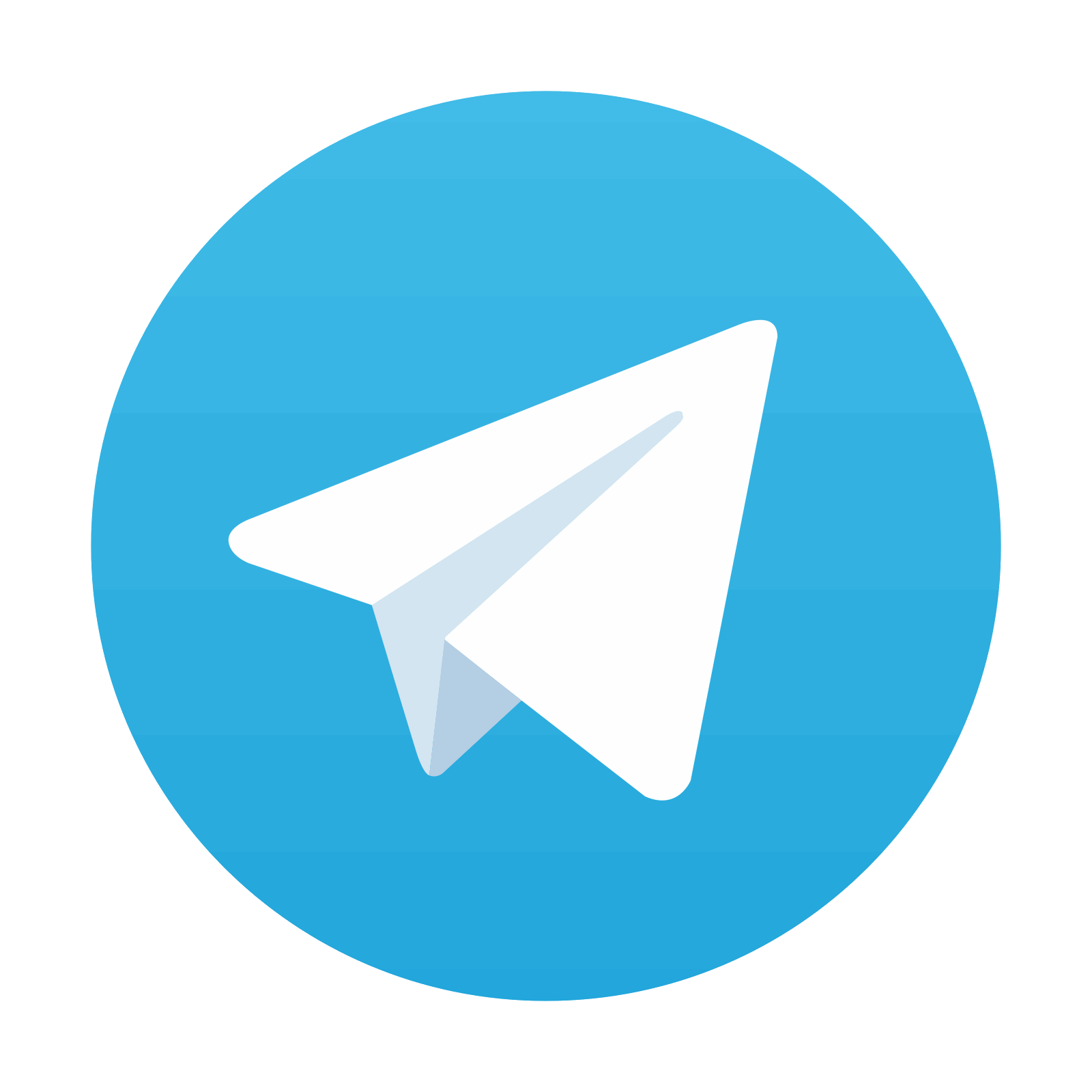
Stay updated, free articles. Join our Telegram channel

Full access? Get Clinical Tree
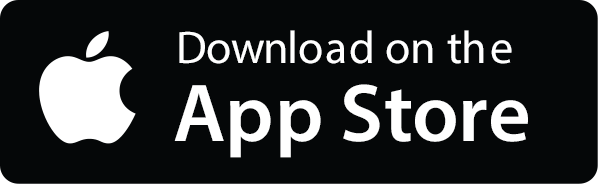
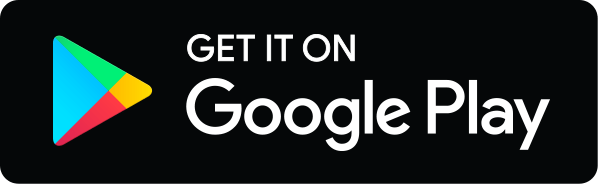
