Background
Current guidelines advise using echocardiography for noninvasive estimation of the likelihood that a patient has pulmonary hypertension (PH). To estimate the echocardiographic probability of PH, the maximal tricuspid regurgitation velocity (TR Vmax) is recommended as the main parameter to use over more complex algorithms that provide an estimation of pulmonary artery pressure. This preference is based on concerns about inaccuracies and amplification of measurement errors that can occur from using derived variables. However, this has not been examined systematically.
Methods
A retrospective database analysis was performed of invasively determined measurements of right heart pressure in 90 patients, corresponding echocardiographic estimations of pulmonary artery pressure, and additional parameters obtained within 24 hours. Several algorithms were compared for their correlations and accuracy parameters.
Results
Although a Bland-Altman analysis demonstrated that all examined algorithms exhibited inaccuracies that could be clinically relevant in individuals, algorithms estimating mean pulmonary artery pressure (PAPm) on the basis of tricuspid regurgitation generally exhibited stronger correlations with invasively determined PAPm and more accurate identification of PH than did TR Vmax. Echocardiographic estimation of right atrial pressure >15 mm Hg exhibited the highest odds ratio for invasively confirmed PH, suggesting that this parameter is of additional diagnostic value. Indeed, algorithms that also considered right atrial pressure performed best, whereas empirical algorithms, TR Vmax, and methods relying on pulmonary acceleration time exhibited weaker performance.
Conclusions
Although all methods are associated with inaccuracies, echocardiographically determined PAPm was superior to the current guideline recommendation of using TR Vmax with regard to its correlation with invasively determined PAPm and the presence of PH. PAPm may be considered as an alternative to TR Vmax for evaluating the echocardiographic probability of PH.
Highlights
- •
This study challenges the current preference for TR Vmax for estimating the probability of PH in the guidelines.
- •
Estimation of PAPm by adding RAP to TR maximal right atrial–right ventricular gradient (by tracing the TR time-velocity integral) resulted in the best correlation with invasively measured PAPm.
- •
Compared with several other algorithms, this method was also associated with the highest OR with regard to the prediction of PH.
- •
Measurement of PAPsys and simple measurement of TR Vmax without addition of RAP were associated with lower predictive values.
Pulmonary hypertension (PH) is defined as a mean pulmonary artery pressure (PAPm) of ≥25 mm Hg at rest as assessed by right heart catheterization (RHC). Although a definite diagnosis of PH requires invasive assessment of PAPm, noninvasive estimation of PAPm from Doppler measurements obtained by transthoracic echocardiography may help either suggest a preliminary diagnosis of PH or make it unlikely. Thus, echocardiography is a Class 1C recommended first-line, noninvasive diagnostic investigation in cases of suspected PH. Furthermore, noninvasive echocardiographic estimation of PAPm may be used to follow and monitor treatment effects in patients with definite PH. The results obtained from echocardiographic examination in combination with the degree of clinical suspicion of PH should be used to define the probability of PH and to determine the need for RHC in an individual patient.
Commonly used methods for estimating PAPm are based on the Doppler-derived velocity-time integral of tricuspid regurgitation (TR). PAPm can be calculated by adding the right atrial–right ventricular mean gradient (TR Pmean) to estimated right atrial pressure (RAP). Alternatively, various empirical formulas for calculating PAPm from the TR-derived systolic pulmonary artery pressure (PAPsys) have been described. However, all TR-based methods for estimating PAPm require estimation of RAP. Alternatively, such as in cases of weak TR signal (no or only trace TR), PAPm may be calculated independently of TR signals using pulmonary acceleration time (PAT) of the pulmonary artery flow as measured by pulsed Doppler ultrasonography.
The 2015 European Society of Cardiology (ESC) and European Respiratory Society guidelines for the diagnosis and treatment of PH recommend continuous-wave Doppler measurement of maximal TR velocity (TR Vmax; not estimated PAPsys or PAPm) as the main variable to use in determining the echocardiographic probability of PH. The reason for this preference according to the guidelines is “the inaccuracies of RAP estimation and the amplification of measurement errors by using derived variables.” However, although these guidelines highlight the impact of echocardiography for estimating the probability of PH, whether the extent of such inaccuracies fully support a preference for TR Vmax was not examined previously. Hence, the best method for assigning the likelihood of PH or noninvasively estimating PAPm is not known. Therefore, we examined the accuracy of various approaches for estimating PAPm and additional parameters, including the TR Vmax method, in a retrospective analysis of unselected patients who underwent echocardiography and RHC.
Methods
Study Design, Study Population, and Definitions
We retrospectively reviewed echocardiographic examinations and the results of RHC performed at the Clinic for Cardiology and Pneumology, University Medical Center Göttingen; King’s College Hospital, London; and the Department of Internal Medicine II, University of Regensburg between 2011 and 2016. In all cases, the maximal interval between echocardiography and cardiac catheterization was 24 hours. The study was conducted as a database search limited to echocardiographic and RHC data as approved by the local ethics committees and in accordance to the amended Declaration of Helsinki. All data relevant to this analysis were subjected to careful review by an experienced imaging specialist who was blinded to the results of the invasive pulmonary pressure measurements. As part of this review, any echocardiographic reading that was suboptimal was remeasured (using Philips Xcelera/TomTec software, Philips, Amsterdam, The Netherlands), and any image (and associated readings) of insufficient quality were excluded from the analysis. The definition of PH and further classification of PH were based on the 2015 ESC guidelines for the diagnosis and treatment of PH: PH was defined as PAPm ≥ 25 mm Hg as assessed at rest by RHC. When PH was diagnosed, it was further classified as precapillary (pulmonary capillary wedge pressure ≤ 15 mm Hg), combined pre- and postcapillary (pulmonary capillary wedge pressure > 15 mm Hg and diastolic pressure gradient ≥ 7 mm Hg), or isolated postcapillary (pulmonary capillary wedge pressure > 15 mm Hg and diastolic pressure gradient < 7 mm Hg).
To identify the best method for noninvasively estimating PAPm, various TR-derived or PAT-derived approaches were compared, as detailed in Table 1 and Figure 1 . In brief, continuous-wave Doppler ultrasonography of TR in apical four-chamber and parasternal short-axis views was applied, and the TR time-velocity tracing was determined to obtain the time-velocity integral, TR Vmax, mean TR velocity, maximal right atrial–right ventricular gradient, and TR Pmean. Patients without or with very weak continuous-wave Doppler signals that did not allow clear determination of the time-velocity signal (such as patients without or with only trace TR) were excluded from the analysis that relied on TR signals. (The application of agitated saline contrast for enhancing weak TR signals was not a part of the standard operational procedures.)
Method | Publication | Number of patients included in this study ( n ) | Maximal time frame between RHC and echocardiography | Study design | Formula/abbreviation |
---|---|---|---|---|---|
Methods for estimating PAPm | |||||
TR-derived methods | |||||
RA-RV mean gradient (TR Pmean) + RAP ∗ (obtained by tracing the TR time-velocity integral added to the estimated RAP) | Aduen et al | 102 | Simultaneously | Prospective | PAPm = TR Pmean + RAP |
Er et al | 164 | Within 120 min | Prospective | ||
Empirical; using PAPsys | Chemla et al , † | 31 | — ‡ | Prospective | PAPm = 0.61 × PAPsys + 2 |
Steckelberg et al , † | 307 (RHC), 109 (echocardiography) | Within 1 mo | Retrospective | PAPm = 0.61 × PAPsys + 1.95 | |
Amsallem et al , † | 307 | Within 5 d | Retrospective | PAPm = 0.60 × PAPsys + 2.1 | |
Friedberg et al | 17 | Within 30 d | Retrospective | PAPm = 0.69 × PAPsys − 0.22 | |
Syyed et al | 65 | — # | Retrospective | PAPm = 0.65 × PAPsys + 0.55 | |
PAT-derived method | |||||
Empirical; using PAT | Dabestani et al | 39 | Within 6 d | Not specified, probably prospective | PAT ≥120 msec: PAPm = 79 − (0.45 × PAT) PAT <120 msec: PAPm = 90 − (0.62 × PAT) |
Other echocardiographic parameters that may correlate with invasive PAPm | |||||
RA-RV maximal velocity (TR Vmax) (obtained by tracing the TR time-velocity integral without addition of RAP) | ESC | No study specified within the guideline | — | — | TR Vmax |
RA-RV maximal gradient (TR Pmax) added to estimated RAP | Rudski et al | No study specified | — | — | PAPsys = TR Pmax + RAP |
PAT (without empirical calculation of PAPm) | Dabestani et al | 39 | Within 6 d | Prospective | PAT |
Granstam et al | 29 | Within 2 d | Retrospective | ||
Yared et al | 371 | — § | Retrospective and prospective ( n = 100) | ||
Kitabatake et al | 33 | Within 1 wk | Not specified, probably prospective |
∗ RAP was estimated according to Rudski et al and Lang et al 13 : diameter of IVC ≤ 21 mm and collapse with sniff >50%, RAP = 3 mm Hg; IVC diameter ≤ 21 mm and collapse with sniff <50%, RAP = 8 mm Hg; IVC diameter > 21 mm and collapse with sniff >50%, RAP = 8 mm Hg; IVC diameter > 21 mm and collapse with sniff <50%, RAP = 15 mm Hg.
† Chemla et al , Steckelberg et al , and Amsallem et al used similar formulas to estimate PAPm from PAPsys. Of those, only the formula proposed by Chemla et al (which was the first one) was used for analysis in our study.
‡ Chemla et al and Syyed et al did not perform echocardiographic examinations. Thus, the relationship between PAPsys and PAPm was derived solely from invasive measurements.
§ Yared et al did not perform RHCs. Thus, the relationship between PAPsys and PAPm was derived solely from echocardiographic measurements.

In all other patients, PAPm was calculated either according to Aduen et al by adding estimated RAP to TR Pmean obtained from tracing of the TR time-velocity integral or by empirical formulas for the calculation of PAPm from PAPsys ( Table 1 ). PAPsys was calculated by adding estimated RAP to TR Pmax. RAP was estimated by measuring the diameter of the inferior vena cava (IVC) at end-expiration and the inspiratory collapsibility in the subcostal view as follows: when the IVC diameter was ≤21 mm and the collapsibility with a sniff was >50%, RAP was estimated to be 3 mm Hg; when the IVC diameter was >21 mm and the collapsibility was <50%, RAP was estimated to be 15 mm Hg. In all other cases (either IVC diameter >21 mm and collapse >50% or IVC diameter ≤21 mm and collapse <50%), RAP was estimated to be 8 mm Hg. To estimate PAPm independent of the measurements of TR (and RAP), PAT was measured as previously described. In brief, the pulmonary artery was visualized in the parasternal short-axis view. The sample volume of the pulsed-wave Doppler was then placed about 1 cm distal to the open leaflet tips of the pulmonary valve in parallel orientation to the axis of blood flow. PAT was measured from the onset of ejection to the peak flow velocity. All echocardiographic dimensions were obtained from two-dimensional images (B-mode).
Data Analysis and Statistical Methods
Continuous variables are presented as median values with the corresponding 25th and 75th percentiles. Comparisons of continuous variables were performed using the Mann-Whitney U test. Proportions are expressed as percentages and as absolute numbers. Categorical variables were compared using the Fisher exact test. To test for correlations between estimated PAPm and invasively measured PAPm, the Spearman coefficient was calculated. CIs were obtained by nonparametric bootstrapping, with 9,999 bootstrap replications. The Spearman correlation coefficients of various echocardiographic variables with invasive PAPm were tested for differences using the approach by Steiger. Bland-Altman plots were used to analyze the degree of agreement between invasively measured PAPm and echocardiographic methods to noninvasively estimate PAPm. Receiver operating characteristic (ROC) analysis served to calculate the area under the curve (AUC) for noninvasive PAPm estimations, TR Vmax, and PAPsys. CIs were derived on the basis of the asymptotic normality of AUC. Youden index quantification was used to determine the optimal cohort-specific cutoff values. The ROC curves were tested for differences using the approach of DeLong et al. Using logistic regression analyses, the odds ratios (ORs) of noninvasive PAPm estimations, TR Vmax, PAPsys, and right ventricular size and function were calculated with regard to the likelihood of PH with 95% CIs also presented. Non-nested logistic regression models were compared using Clarke’s test. According to guideline recommendations, reference values were <25 mm Hg for PAPm, <2.8 m/sec for TR Vmax, <100 msec for PAT, and <35 mm Hg for PAPsys. Data were analyzed using SPSS version 22.0 (SPSS, Chicago, IL) and SAS version 9.4 (SAS Institute, Cary, NC). Statistical tests were two tailed and used a significance level of P < .05.
Results
Overall, 90 patients with a maximum time frame of 24 hours between RHC and echocardiographic examination were identified. The median time frame was 18.8 hours (7.6–21.7 hours). Baseline characteristics, echocardiographic findings, and results of RHC are summarized in the online supplement ( Supplemental Table 1 ). In brief, the mean age was 64.8 years (55.0–78.0 years), and 52.4% of patients were women. PH as determined by RHC was present in 68 patients (75.6%). Of these, 17 patients (25.0%) had precapillary, 11 patients (21.6%) had combined pre- and postcapillary, and 40 patients (58.8%) had isolated postcapillary PH. The median PAPm was 35.0 mm Hg (24.7–46.2 mm Hg). There was a near Gaussian distribution of invasively measured PAPm values ( Figure 2 ).

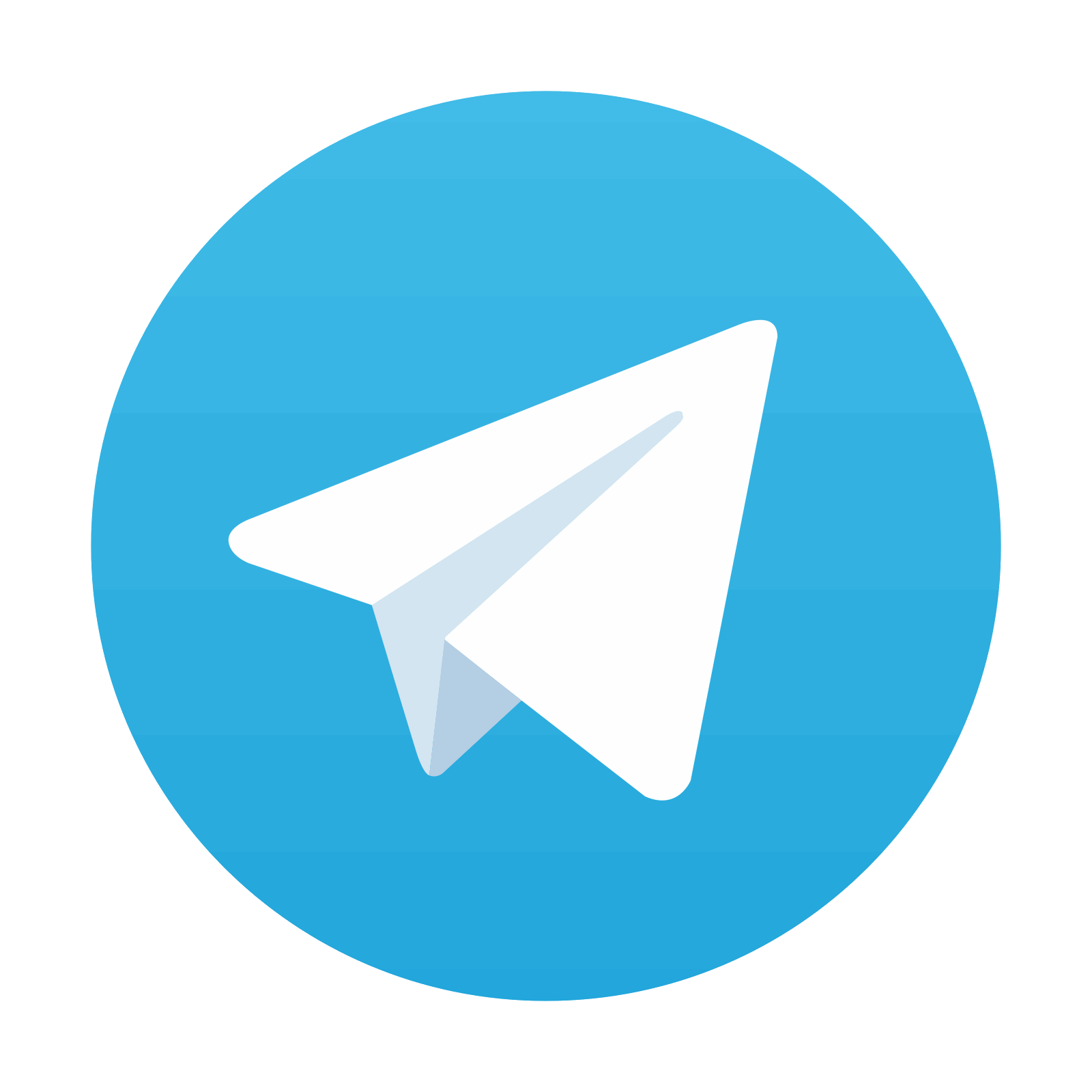
Stay updated, free articles. Join our Telegram channel

Full access? Get Clinical Tree
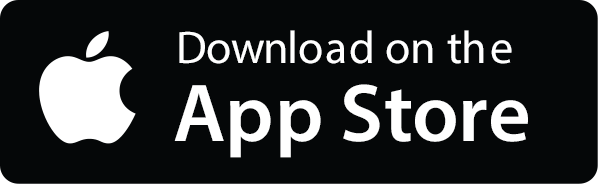
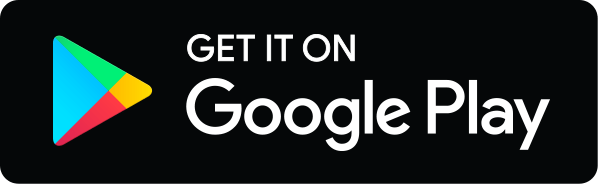
