Fig. 3.1
(a) Dilatation of the basal inferior wall (yellow arrow a) as a result of myocardial infarction. (b) Jet of anteriorly-directed MR (yellow arrow b) resulting from restriction of the posterior leaflet secondary to inferior myocardial infarction
This chapter focuses on chronic FIMR, which is the result of remodeling of the ventricle over time after infarction.
The role of the echocardiocardiogram is threefold: first to confirm the diagnosis of FIMR, which encompasses a detailed assessment of the mechanism of regurgitation, the left ventricle and also exclusion or full description of other cardiac structural or functional abnormalities which may be contributing to the patient’s presenting symptoms. Secondly, evaluation of severity by both qualitative and quantitative methods [6, 7] and finally (and increasingly important) the identification of prognostic features, such as those which predict the possibility of a less satisfactory outcome after surgical repair [8], and the presence of pulmonary hypertension (Fig. 3.2).


Fig. 3.2
Pulmonary hypertension in a patient with severe FIMR – the PASP is estimated as 4 V2 + RAPmmHg where V is the Vmax of the tricuspid regurgitant signal measured from the CW Doppler trace and RAP is right atrial pressure estimated from the size and inspiratory reactivity of the IVC
It must be noted that due to the complex, dynamic nature of FIMR, there is no one parameter which surmounts all others in terms of usefulness either diagnostically or prognostically: conventional 2-dimensional measurements and Doppler indices of regurgitation severity must be combined with the newer techniques of 3-dimensional and speckle-tracking echocardiography to provide an in-depth morphological assessment of valve anatomy and ventricular geometry, focusing on the effects of ischemic remodeling. The approach must be fully-comprehensive in every patient and the final conclusion is a synthesis of all the relevant information which can be used to inform clinical decision-making in a heart-team context [9].
Diagnosis of FIMR and Quantification of Severity
Confirming a Diagnosis of Functional Ischemic MR by Echocardiography
Correctly identifying the mechanism of MR as functional and ischemic and correctly evaluating its severity can be surprisingly challenging. It is therefore fundamental to success that the echocardiologist has an in-depth understanding of the normal morphology and physiology of the mitral valve-LV complex [10]. The term “mitral valve-LV complex includes the leaflets, annulus, chordae, papillary muscles and supporting left ventricular myocardium, and the exact geometry required to produce perfect leaflet coaptation may be disrupted by abnormalities of any one of these components. Transthoracic echocardiography (TTE) may in many cases prove sufficient to confirm the diagnosis, but transesophageal echocardiography (TEE) is needed in cases where the ischemic mechanism is unclear and is also a useful adjunct for delineating the mechanism and quantifying morphological parameters in the pre- and perioperative patient thus allowing for advanced planning of individually-tailored intervention [11]. Guidelines state that all patients being considered for mitral intervention should undergo transesophageal echocardiography [19].
Many, but not all, patients will give a definite history of myocardial infarction and/or coronary artery disease. Before assessing the mitral valve itself, the echocardiographer must first make a thorough assessment of the left ventricle (LV), including wall thickness and diastolic and systolic chamber dimensions and volumes, either from 2-dimensional or 3-dimensional imaging (Fig. 3.3). The abnormality of the ventricle may be global dilatation or regional/segmental dysfunction (Fig. 3.4). Differentiation between the two is key to classification of the FIMR. Identification of a segmental wall motion abnormality suggesting previous infarction is often the key finding and the typically-affected segments are the basal and mid inferior and/or inferolateral segments [12]. These regional abnormalities can be very subtle, particularly if the infarct is subendocardial. In addition, in the presence of moderate or severe MR, the resulting hyperdynamic wall motion of other segments will tend to mask mild segmental abnormalities. The key is to have a high degree of suspicion in any patient with a history of CAD or significant risk factors and to look for abnormalities of thickening rather than motion. Newer techniques such as analysis of basal rotational dynamics and segmental layered strain analysis by speckle-tracking echocardiography can be a useful adjunct if there is doubt about the presence of a segmental wall motion abnormality [13]. Cardiac magnetic resonance imaging has also proved to be helpful in these situations, in that late-enhancement with gadolinium contrast agents can identify areas of segmental partial thickness infarction, which may be revealed even in patients who do not have a confirmed history of myocardial infarction (Fig. 3.5) [14]. As infarction of the basal inferior and inferolateral segments is a predictor of recurrent MR after mitral ring annuloplasty this author recommends cardiac MRI in any patient with valve morphology consistent with FIMR but no history or obvious regional wall motion abnormality.




Fig. 3.3
Measuring volumes and LVEF by Simpson’s Biplane Method of Discs in a patient with MR and very severely impaired LV function

Fig. 3.4
Global and Regional Dysfunction causing FIMR. (Panel a) Globally-dilated ischaemic ventricle at end-diastole. (Panel b) Globally dilated ventricle at end-systole. (Panel c) Jet of functional ischaemic MR in association with globally-dilated ventricle. (Panel d) Zoomed PLAX image showing restricted posterior leaflet due to localised basal inferolateral infarction. (Panel e) posteriorly-directed jet of MR due to asymmetric tethering of the posterior leaflet

Fig. 3.5
(a) Cardiac magnetic resonance imaging with gadolinium contrast showing late-enhancement in the subendocardium of the basal posterolateral segment (arrowed) in a patient who presented with pulmonary oedema secondary to severe MR but with no history of previous myocardial infarction. (b) Apical 2-chamber view in the same patient showing infarcted basal inferior wall. (c) Colour jet of MR due to assymetric tethering of the posterior leaflet in this patient
Leaflet Tethering
Leaflet tethering or restriction (the terms are used interchangeably) due to displacement of one or both papillary muscles (PM) is the key echocardiographic finding in FIMR. Tethering may be symmetrical, affecting both leaflets, when it is also referred to as “tenting” (Fig. 3.6) or asymmetrical, affecting only one leaflet (Fig. 3.7a) [15]. If only one leaflet is affected, it is more often the posterior leaflet, as the posteromedial papillary muscle tends to have a single-vessel blood supply and so inferior or inferolateral infarction is more likely to cause asymmetric tethering than anterior infarction – the P3 scallop can be restricted almost in isolation if an infarct is limited and well-circumscribed. The result is that the anterior leaflet coaptation zone or rough zone apposes the body of the posterior leaflet rather then its corresponding coaptation zone. This reduces the coaptation length and produces an eccentric jet of mitral regurgitation – directed towards the restricted leaflet ie most commonly posteriorly-directed (Fig. 3.7b).



Fig. 3.6
Symmetrical tethering. (a) Deep symmetrical tethering of both anterior and posterior mitral leaflets shown in the parasternal long axis (PLAX) view by 2D Echo. The yellow shaded area is referred to as the tenting area. (b) Symmetrical tethering in a 2 chamber view. The red line is the annular plane and the yellow line indicates the tenting height/depth. (c) The anterior leaflet angle (α) is the angle subtended by the base of the anterior leaflet and the annular plane. The posterior leaflet angle (β) is that subtended by the base of the posterior leaflet and the annular plane. A posterior leaflet angle of >45° is associated with a higher rate of failure of mitral repair in FIMR

Fig. 3.7
Asymmetrical Tethering. Panel a shows tethering affecting the posterior leaflet in a zoomed parasternal long axis (PLAX) view; Panel b shows the corresponding jet of severe posteriorly-directed mitral regurgitation
If the area of myocardial dysfunction is large and involves several segments, or if there is extensive ventricular remodeling, then LV dilatation may be global. The resulting apical displacement and increased separation of the papillary muscles causes both leaflets of the mitral valve to be tethered or tented into the LV. In these cases, the LV function is usually more severely impaired and the resultant mitral regurgitant jet is more central – though it is rarely absolutely central. There have been numerous studies investigating possible relationships between LV systolic dysfunction, dilatation and severity of FIMR. In an animal model it has been shown that systolic dysfunction in isolation does not cause MR, presumably because without the additional forces tethering the leaflets into the ventricle there is still sufficient force generated to produce sufficient leaflet coaptation. However, in the same model, when the LV was allowed to dilate, causing tethering of one or both leaflets, the result was MR. This confirms that the outward and apical displacement of the PMs is the key mechanism of FIMR. Other work in animal models suggests that ischemia or infarction of the PMs without displacement is not directly related to severity of FIMR. In a canine model there is poor correlation between reduced PM thickening and MR severity, and in another study FIMR was modeled by occlusion of the left circumflex artery in sheep. Ischemia and dysfunction of the PM in the ovine model correlated with reduced rather than increased MR, presumably due to reduction in tethering forces acting on the posterior leaflet. It is also recognized in humans that following inferior infarction, “waisting” or elongation of the posteromedial PM may prevent the occurrence or reduce the severity of FIMR. Gadolinium-enhanced cardiac MRI has also shown no firm correlation between PM infarction and FIMR.
The most important distinction to make is whether leaflet tethering is symmetrical or asymmetrical. In the context of limited inferior or inferolateral infarction, affecting one or two myocardial segments only, the outward displacement of the affected segments and distortion of the posterior annulus tethers only the posterior leaflet – often the P3 scallop is severely affected – and this produces a characteristic appearance which may be mistakenly interpreted by the less-experienced as “prolapse of the anterior mitral valve leaflet tip”. In reality, the coaptation point does not come above the annulus, but failure of the tethered posterior leaflet to move upwards towards the annular plane results in the tip of the AMVL meeting the body of the PMVL. The resulting coaptation is highly inefficient and produces a highly-eccentric jet of IMR directed towards the tethered leaflet i.e., posteriorly around the posterior wall of the left atrium (LA). Tenting height, tenting area and tenting angles should also be measured in the parasternal long axis (PLAX) view or apical four chamber (A4Ch) view (Fig. 3.6). Tenting height (or depth – the terms are used interchangeably) is usually measured in the A4Ch view as the maximum mid-systolic distance from the coaptation point to the annular plane. This gives an assessment of the degree of apical shift of the coaptation point and reduction in coaptation length. The term “tenting angles” refers to the relationship between the base of the leaflets and the annular plane. The anterior leaflet angle is termed “α” and the posterior leaflet angle “β”. Slices may be selected from 3D volumetric datasets to calculate tethering angles, but unfortunately these angles do vary depending on the exact slice selected and there is as yet no defined standard for how this should be done. In general terms, the greater the ratio of posterior leaflet angle to anterior leaflet angle, the greater the degree of asymmetric tethering, and increased asymmetry of tethering is associated with increased MR severity. A posterior leaflet angle of >45° is also important as a predictor of an unsatisfactory result after ring annuloplasty (see below). Tenting area is probably the most reproducible measurement as it is not dependent on delineation of one particular angle. It is defined as the area bounded by the anterior and posterior leaflets and the annular plane, usually in the PLAX view, and should be measured in mid-systole when the area is at a maximum. A tenting area of ≥ 4 cm2 has been shown to be a predictor of moderate-severe FIMR over a 2-year period, and also of worsening of FIMR. In patients with systolic dysfunction, tenting area is a major determinant of FIMR severity, independent of global LV function or sphericity. This is probably because tenting area correlates with linear measures of PM displacement and thus acts as a surrogate marker for the severity of posterior and apical PM displacement. 3D volumetric datasets can be used to measure tenting volumes. However whether tenting areas or volumes are measured, it should be noted that for any given value of tenting area or volume, a greater degree of asymmetric tethering will be associated with more severe FIMR.
In addition to tethering of the leaflets due to PM displacement, the body of the anterior leaflet may also be tethered by its secondary chords, causing it to fold into a slight “V” shape in systole – sometimes called the “seagull sign”, referring to the silhouette of a seagull’s wings in flight. This form of asymmetric tethering is also associated with more severe FIMR.
Annular Dilatation
Dilatation of the annulus is often found in association with FIMR, as it can be caused by global LV dilatation or regional remodeling and exacerbated by increased LA size due to either chronically increased filling pressures or atrial fibrillation. Determining the size of the annulus is useful to the surgeon or interventionist and should be measured in at least two planes – across the commissures and in the antero-posterior direction. These are best appreciated from the 60° and 120° lower esophageal views by TEE (Fig. 3.8). Annular area can also be calculated assuming an elliptical shape by the formula π(d/2) A x (d/2) B where A is the diameter of the long axis of the ellipse (ie across the commissures) and B is the short axis (anteroposterior diameter) or by using one of the widely available software analysis packages for quantification of the mitral valve. After infarction, reduced annular contraction correlates with increased MR severity.


Fig. 3.8
Measuring the mitral annulus by TEE 60o (a) and 120° (b) views. Correct views for measuring dimensions of the mitral annulus during TEE. (a) The “bi-commissural” view, obtained at approximately 60° gives the dimension of the annulus across the commissures. (b) The 120° view gives the true AP annular dimension. If there is remodelling of the posterior wall and mitral annulus, then the AP dimension may almost equate to the dimension across the commissures, i.e., the mitral annulus becomes more circular
The Mitral Leaflets
Although it is usually asserted that FIMR is a condition of the ventricle and not the valve itself, and that the leaflets are “normal”, some work has suggested that over time, the leaflets do change in size and shape. In one study using 3D echocardiography to measure leaflet areas in humans, it was shown that total mitral leaflet area is greater in patients with FIMR than in patients without LV dilatation or previous infarction. However, the ratio of the total area to the “closing area” was decreased [16]. It has been postulated that the adaptation of the leaflets over time is due to reactivation of embryonic development pathways which allow the leaflets to enlarge and thicken, thus effectively remodeling the valve. Leaflet area can be appreciated well from 3-dimensional TOE datasets reconstructed using widely-available commercial analysis packages.
Assessment of Severity of Functional Ischemic Mitral Regurgitation
Echocardiographic assessment of the severity of functional ischemic mitral regurgitation is complicated by the dynamic nature of the condition, which is predominantly due to the changing function of the ventricle depending on pre and afterload. This load dependency means that there is no one parameter which alone indicates the severity of regurgitation. Clues to the severity can be gained both directly and indirectly and from all different modalities of echo. In this part of the chapter we will review each modality and what can be gained from it.
2-Dimensional Echocardiography
The dimensions and contractility of the LV can give the first clue to the severity. A reduced end-systolic dimension or volume is an indicator of hyperdynamic function due to a high degree of off-loading into the left atrium (LA) in severe MR. However, this can be confounded in the presence of severe ischemic impairment of LV function – infarcted myocardium is unable to contract so dimensions may not reflect the severity of MR. Annular size should always be measured and as this changes throughout the cardiac cycle, mid-systole is a reasonable point to do this. Dilatation of the annulus is a common finding in functional MR. The degree of tethering of the leaflet should be estimated and this is done by tracing the area bounded by the leaflets at end-systole and the annular plane. This “tenting area” is directly related to the chance of successful repair and so is vital information for the surgeon. The coaptation point and length should be measured. Only when the morphology of the valve has been fully delineated should other methods of echocardiography be used.
Color Doppler
Whilst the echocardiographic diagnosis of MR depends on the demonstration of a jet of mitral regurgitation on color flow Doppler imaging, this is not a good method of assessing severity. Nevertheless, there are some important clues which can be gained from an assessment of color flow.
Jet Direction and Area
The color jet alone is not a good indicator of severity. If imaged by 2-D echo it should be imaged in several planes to build up a 3-dimensional impression in the mind of the sonographer. An eccentric jet suggests asymmetric leaflet tethering and a more central jet is usually associated with global remodeling and annular dilatation. A jet which hugs the wall of the LA may appear to be very thin but as it is subject to the Coanda effect, whereby fluids are attracted to adjacent surfaces, the apparent slimness of the color jet is deceptive and these jets can be due to MR of significant volume [17] (Fig. 3.9). In broad terms, a jet with an area of <4.0 cm2 or which occupies <20 % of the area of the left atrium is consistent with mild MR.


Fig. 3.9
Wall-hugging jets of MR often contain higher regurgitant volumes despite the relative slimness of the jet, which is due to the Coanda effect
Vena Contracta
The vena contracta (VC) is the narrowest part of the jet, just as it emerges through the regurgitant orifice into the LA (Fig. 3.10). In theory it is a surrogate for the regurgitant orifice area. However, the regurgitant orifice is unlikely to be a regular shape in cross-section, so the VC width varies greatly depending on the plane chosen for its measurement. Parasternal views are recommended, but if this is not possible, it may be measured from the A4Ch or ALAX view (not the A2Ch view, as this typically tends to give a larger value). Values of ≤3 mm are consistent with mild MR and values ≥7 mm with severe MR but values in between fall into a grey area containing some mild, many moderate and some severe cases. Recently, the measurement of VC area (VCA) by 3D TEE has been shown to be consistent and reliable as a plane can be selected which gives a true cross-section through the jet at the point closest to the orifice [18]. A good description of how to do this practically can be found in the review by Thavendiranathan et al. [19] (Fig. 3.11).



Fig. 3.10
2D vena contracta. The vena contracta (VC) is best measured in the PLAX view but in practical terms it tends to be measured in whichever view it is seen most clearly. This may lead to error as the VC width varies greatly depending on the plane chosen for measurement. 3D VC measurements have been shown to be more consistent

Fig. 3.11
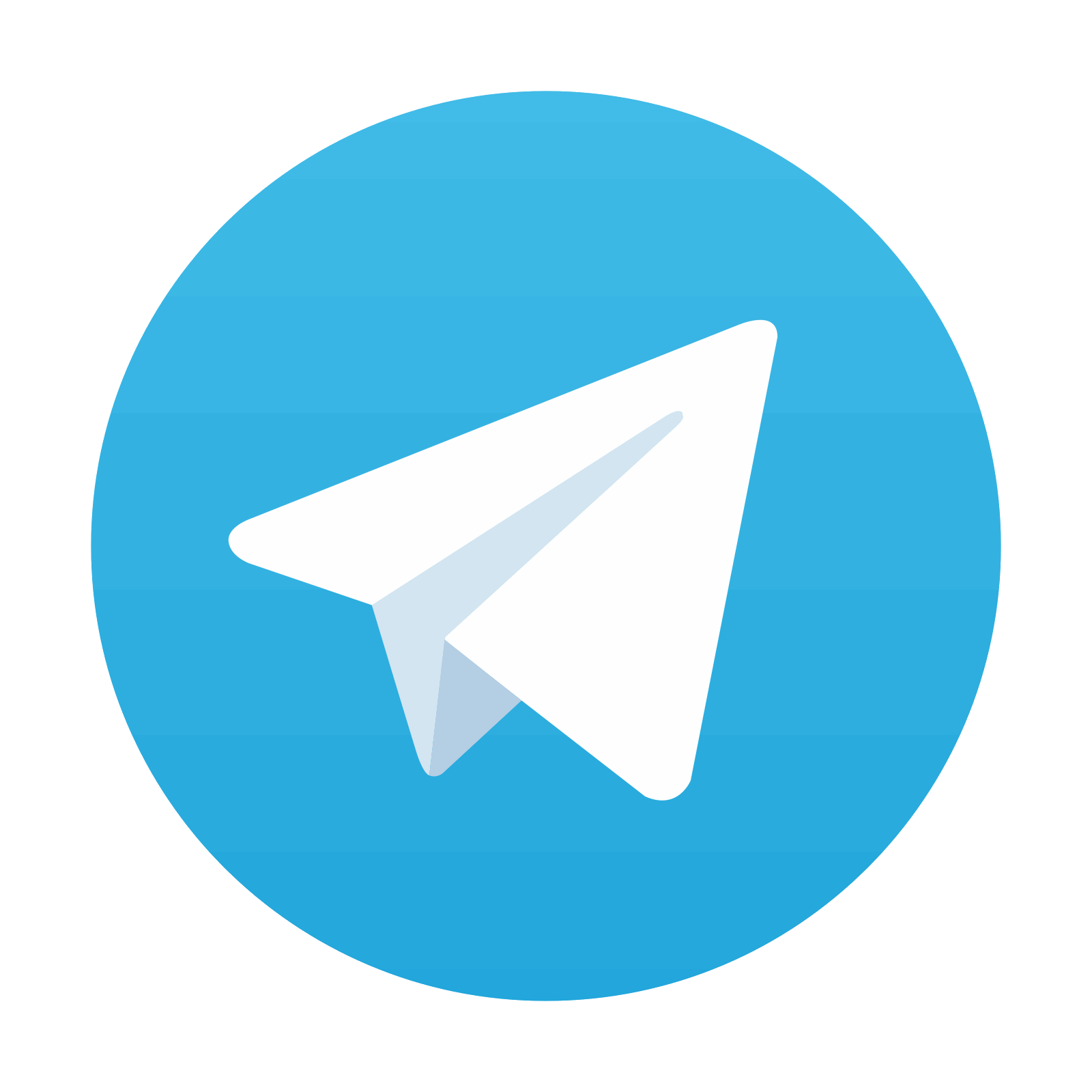
3D Vena contracta. VCA Measurement Using 3D Color Full-Volume Acquisition by TEE. (a, b) Multiplanar reformatting to obtain the best view of the regurgitant jet and the vena contracta. (c) En face view of the vena contracta area (VCA) with planimetry. (d) Reformatted 3-dimensional (3D) volume illustrating the regurgitant jet. (a2) and (b2) illustrate the effect of a change in systolic phase and the location of the en face (blue) plane, respectively, on the VCA measurement. (From Thavendiranathan et al. [19]. With permission from Elsevier)
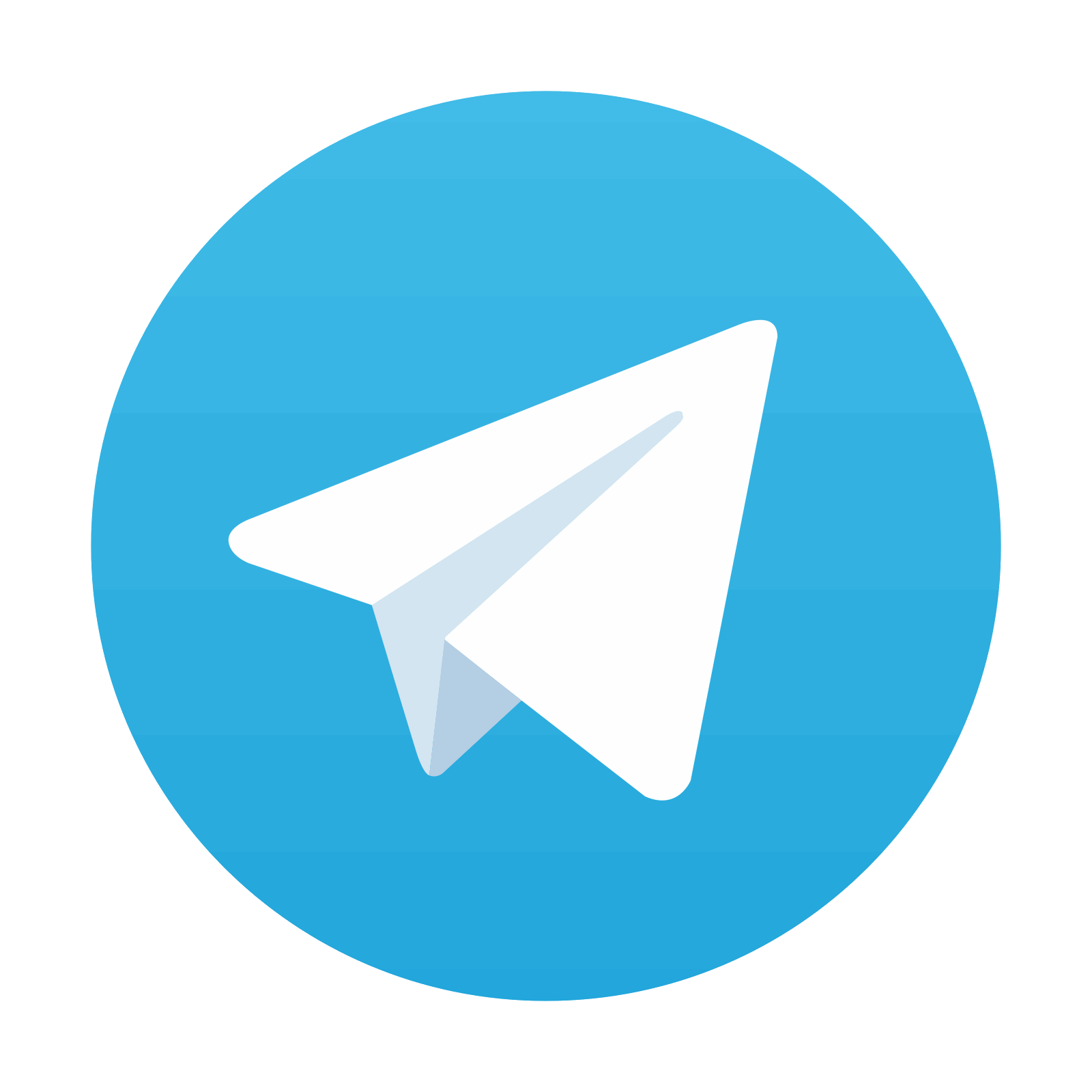
Stay updated, free articles. Join our Telegram channel

Full access? Get Clinical Tree
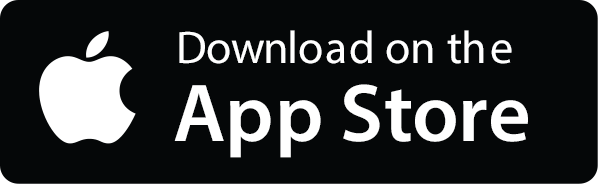
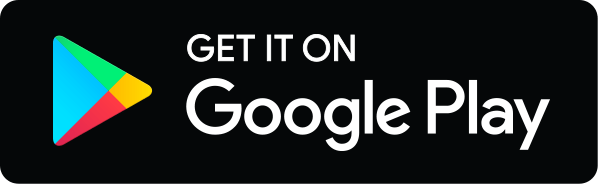
