Background
Aortic pulse-wave velocity (PWV) is a measure of aortic stiffness that has a prognostic role in various diseases and in the general population. A number of methods are used to measure PWV, including Doppler ultrasound. Although echocardiography has been used for PWV measurement, to the authors’ knowledge, it has never been tested against an invasive reference method at the same time point. Therefore, the aim of this study was to compare prospectively an echocardiographic PWV measurement, called echo-PWV, with an invasive study.
Methods
Forty-five patients (mean age, 66 years; 60% men) underwent simultaneous intra-arterial pressure recording and echocardiographic Doppler flow evaluation during elective cardiac catheterization. Proximal pressure and Doppler waveforms were acquired in the aortic arch. Distal pressure waveforms were registered in the right and distal Doppler waveforms in the left external iliac artery. Transit time was measured as a delay of the foot of pressure or Doppler waveform in the distal relative to the proximal location. Distance was measured on the catheter for invasive PWV and over the surface for echo-PWV. Echo-PWV was calculated as distance divided by transit time.
Results
In the whole group, mean invasive PWV was 9.38 m/sec and mean echo-PWV was 9.51 m/sec ( P = .78). The Pearson’ correlation coefficient between methods was 0.93 ( P < .0001). A Bland-Altman plot revealed a mean difference between invasive PWV and echo-PWV of 0.13 ± 0.79 m/sec.
Conclusions
Echo-PWV, based on Doppler echocardiography, is a reliable method of aortic PWV measurement, with a close correlation with invasive assessment. Wider implementation of the echo-PWV method for the evaluation of aortic wall stiffness can further expand the clinical and scientific utility of echocardiography.
Aortic pulse-wave velocity (PWV) is a measure of aortic stiffness, and it has an established prognostic role in cardiovascular diseases and in the general population. Several studies have shown that increased PWV independently predicts cardiovascular outcomes, including cardiovascular or all-cause mortality in patients with renal insufficiency, arterial hypertension, and diabetes and in the general population. Increased PWV also predicts incident hypertension in initially normotensive individuals.
At the same time, echocardiography is the most widely available imaging technique in clinical practice. However, there is a need to better demonstrate that echocardiography can be more often used for prognostic assessment in patients with cardiovascular diseases. It was one of the leading goals of the American Society of Echocardiography roadmap for 2020.
Assessment of the echocardiographic method used for PWV measurements addresses an important clinical issue and it will potentially expand the use of echocardiography. Unfortunately, there have been only a few studies of aortic PWV measured using Doppler echocardiography. These studies included patients with Marfan syndrome, arterial hypertension, and heart failure, as well as children. Regional PWV in different aortic segments was assessed using Doppler echocardiography, with good intra- and interobserver variability. Echocardiographically measured PWV was shown to independently correlate with descending aortic atherosclerosis evaluated by transesophageal echocardiography.
However, we were not aware of studies directly and simultaneously comparing PWV measured with Doppler echocardiography with invasive method. Therefore, we planned a prospective study with direct and simultaneous comparison of echocardiographic and invasive methods of aortic PWV measurement in patients scheduled for elective coronary angiography.
We hypothesized that echocardiographically measured PWV would be highly correlated with invasive measurement. The approach used in this study we call echo-PWV, to indicate the connection of prognostically important PWV results with widely available standard echocardiographic techniques. If feasible, echo-PWV would potentially further expand the role of echocardiographic techniques in cardiovascular medicine, in both the clinical and research settings.
Methods
Patients
We initially evaluated 57 patients scheduled for elective coronary angiography with femoral access who were in stable sinus rhythm on electrocardiography during preparation for the invasive study and who had no significant aortic stenosis or significant peripheral artery disease in their medical histories. From this group, four patients were excluded because of poor visualization of the aortic arch, three patients because of arrhythmia that occurred during the study, and five patients because of abnormal Doppler waveforms in the left external iliac artery (EIA), interpreted as related to suspected aortoiliac occlusive disease. Finally, 45 patients were evaluated prospectively and analyzed as planned. Characteristics of the patients are presented in Table 1 . Indications for coronary angiography included stable severe angina (Canadian Cardiovascular Society grade III) in eight patients, stable phase of non–ST-segment elevation myocardial infarction in six patients, suspicion of restenosis of a coronary stent or coronary bypass stenosis in seven patients, elective percutaneous coronary intervention in seven patients, evaluation of the cause of left ventricular systolic dysfunction in five patients, evaluation of coronary artery disease before hepatic or renal transplantation in two patients, evaluation of the cause of ventricular arrhythmia in four patients, elective evaluation of the significance of coronary stenosis using intravascular ultrasound or fractional flow reserve measurement in five patients, and suspicion of coronary artery disease on the basis of the results of computed tomographic angiography in one patient.
Characteristic | Value |
---|---|
Number of patients | 45 |
Age (y) | 66 ± 12 |
<50 | 4 (9) |
50–70 | 25 (55) |
>70 | 16 (36) |
Men/women | 27/18 |
BMI (kg/m 2 ) | 27 ± 4 |
SBP (mm Hg) | 145 ± 19 |
DBP (mm Hg) | 72 ± 11 |
HR (beats/min) | 67 ± 10 |
LVEF (%) | 51 ± 11 |
CAD | 40 (89) |
One-vessel disease | 12 (30) |
Two-vessel disease | 8 (20) |
Three-vessel disease | 20 (50) |
Hypertension | 39 (87) |
Diabetes | 12 (27) |
Active or past smokers | 23 (51) |
Pharmacologic treatment | |
ACE inhibitors/ARBs | 40 (89) |
β-blockers | 40 (89) |
Statins | 41 (91) |
Aspirin | 39 (87) |
The study was approved by the local ethics committee, and all patients gave informed written consent to participate in the study.
Invasive PWV Measurement
At the beginning of the angiographic study (Sensis; Siemens Healthcare, Erlangen, Germany) (2,000-Hz sampling rate of electrocardiographic and pressure signal), after the introduction of a 6-Fr vascular sheath into the right common femoral artery, pressure tracing from the aortic arch was recorded using a multipurpose catheter (PendraCare, Leek, The Netherlands) at a speed of 200 mm/sec. Ten consecutive cardiac cycles were stored and printed, and the position of the catheter was marked at the entrance to the sheath with a sterile external marker. Next, the catheter was withdrawn to the external femoral artery and marked at the entrance to the sheath, and again 10 consecutive cycles were stored and printed. After that, the catheter was fully withdrawn, and the distance between the markers was measured. This represented the distance between proximal and distal points of measurements. Transit time was calculated as the difference between the time from the tip of the S wave to the beginning of the pressure wave in the femoral artery and time from the tip of the S wave to the beginning of pressure wave in the aortic arch ( Figure 1 ). The mean time from 10 consecutive cardiac cycles was used for analysis. The point of the beginning of pressure wave was marked manually using the method of intersecting tangents, one tangent through the point of lowest pressure and the second tangent to the steepest part of the pressure-wave upstroke ( Figure 2 ). PWV was calculated as the distance between markers on the catheter divided by transit time.


Echo-PWV Measurement
Simultaneously with invasive pressure recordings, Doppler echocardiography with the use of a portable system (Vivid I; GE Healthcare, Milwaukee, WI) was performed. The pulsed-wave Doppler sample volume was set at 5.07 mm and the low-velocity filter was reduced to get the beginning of the waveform adjacent to the baseline. Ten consecutive Doppler waveforms were recorded at a sweep speed of 100 mm/sec, first in the distal aortic arch and then in the left distal EIA. Images were stored and evaluated using dedicated software (EchoPAC; GE Healthcare). Transit time was calculated as the difference between time from the QRS complex to the beginning of the EIA Doppler waveform and time from the QRS complex to the beginning of the distal aortic arch Doppler waveform ( Figure 3 ). The mean time from 10 consecutive cardiac cycles was used for analysis. The distance between two points of the Doppler recordings was measured over the body surface with a flexible measuring tape. The distance from the suprasternal notch to the umbilicus was added to the distance from the umbilicus to the left groin. Then the distance between the suprasternal notch and the Doppler gate in the distal aortic arch was subtracted. Both Doppler echocardiography and distance measurements were performed by a single experienced echocardiographer. Calculations of invasive and echo-PWV measurements were performed offline by two independent observers. Interobserver variability assessment of invasive and echo-PWV was based on the variability of transit time, as distance was measured only once during cardiac catheterization.

Statistical Analysis
Statistical analysis was performed using Statistica version 12 (StatSoft, Tulsa, OK). Continuous data are presented as mean ± SD, and numeric data are presented as percentages. For comparison of mean values between two methods, Student’s t test for paired data was used. The Spearman correlation coefficient was used to measure associations between the methods. Agreement between the methods was evaluated using Bland-Altman analysis. Interobserver variability of invasive PWV and echo-PWV was measured using the intraclass correlation coefficient and the absolute difference between repeated measurements expressed as a percentage of their mean.
Results
Mean PWV measured invasively was 9.43 m/sec, and mean echo-PWV with Doppler echocardiography was 9.56 m/sec ( P = .78). The Spearman correlation coefficient ( Figure 4 ) between methods was 0.93 ( P < .0001). The Bland-Altman plot ( Figure 5 ) revealed a mean difference between the methods of 0.13 ± 0.79 m/sec. The limits of agreement (2 SDs) between methods were 1.7 and 1.43 m/s. The mean transit time measured invasively was 57.2 msec and measured with echo-PWV was 53.8 msec ( P = .24). The mean difference between two methods was 3.4 ± 6 msec, with limits of agreement (2 SDs) of 9.3 and 15.2 msec. The mean distance measured invasively was 51.3 cm and with echo-PWV was 48.7 cm ( P = .01). The mean difference between two methods was 2.5 ± 4.7 cm, with limits of agreement (2 SDs) of 11.8 and 6.8 cm. In the subgroup of 24 patients <65 years of age, the accuracy of echo-PWV versus invasive PWV improved even further, with a mean difference between the methods of 0.03 ± 0.66 m/sec, with limits of agreement (2 SDs) of 1.32 and 1.26 m/sec. Interobserver variability of invasive PWV and echo-PWV was 0.98 (95% CI, 0.96–0.99) and 0.96 (95% CI, 0.93–0.98), respectively. The absolute difference between repeated measurements, expressed as a percentage of their mean, was 3.61 ± 2.56% for invasive PWV and 5.20 ± 3.24% for echo-PWV.
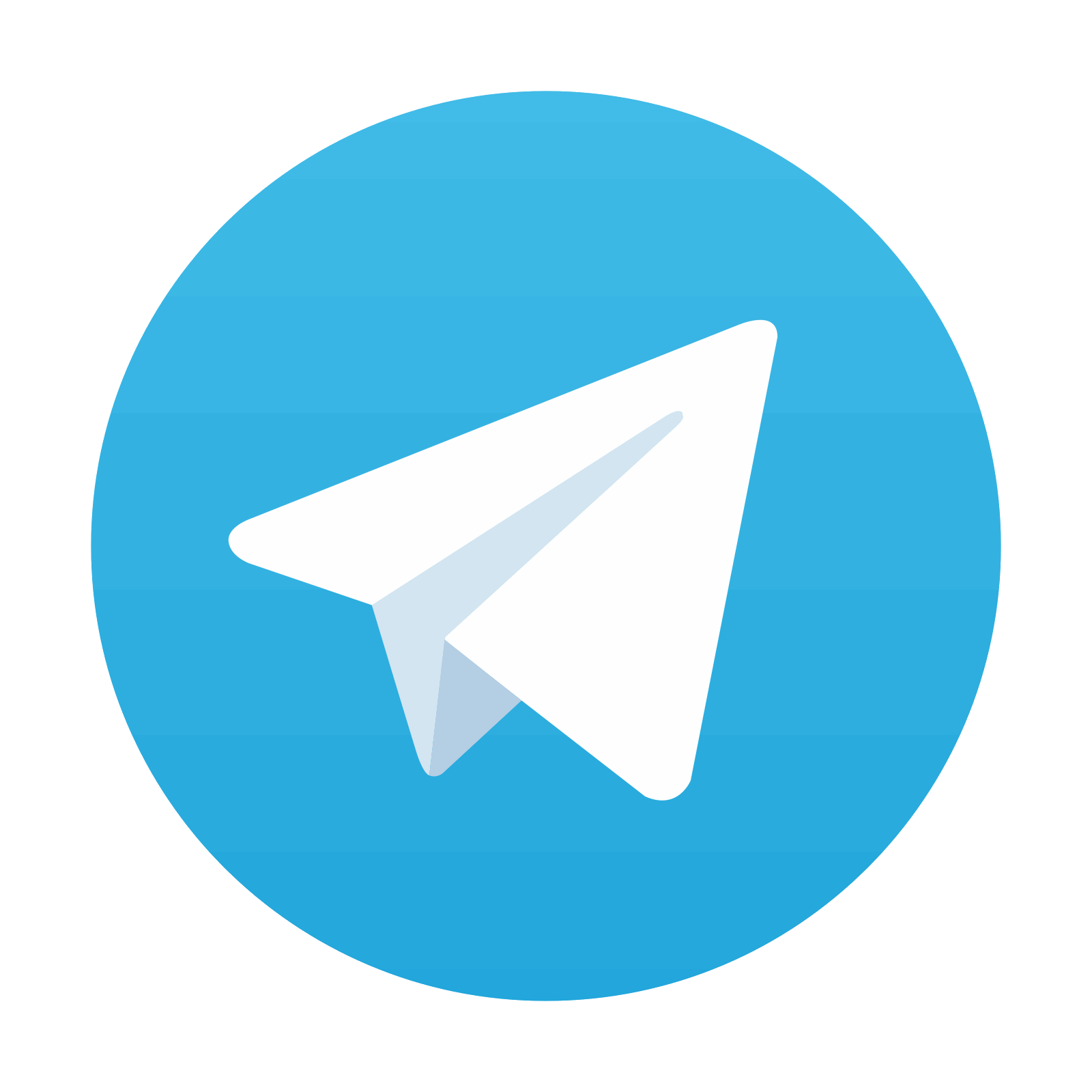
Stay updated, free articles. Join our Telegram channel

Full access? Get Clinical Tree
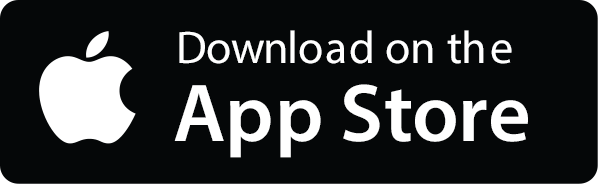
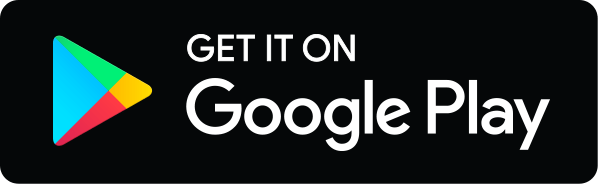
