There has been significant growth in the volume and complexity of percutaneous structural heart procedures in the past decade. Increasing procedural complexity and accompanying reliance on multimodality imaging have fueled the development of fusion imaging to facilitate procedural guidance. The first clinically available system capable of echocardiographic and fluoroscopic fusion for real-time guidance of structural heart procedures was approved by the US Food and Drug Administration in 2012. Echocardiographic-fluoroscopic fusion imaging combines the precise catheter and device visualization of fluoroscopy with the soft tissue anatomy and color flow Doppler information afforded by echocardiography in a single image. This allows the interventionalist to perform precise catheter manipulations under fluoroscopy guidance while visualizing critical tissue anatomy provided by echocardiography. However, there are few data available addressing this technology’s strengths and limitations in routine clinical practice. The authors provide a critical review of currently available echocardiographic-fluoroscopic fusion imaging for guidance of structural heart interventions to highlight its strengths, limitations, and potential clinical applications and to guide further research into value of this emerging technology.
Highlights
- •
Echocardiographic-fluoroscopic fusion has the potential to improve procedural guidance in the future.
- •
Echocardiographic-fluoroscopic fusion allows real-time imaging, an advantage over other modalities.
- •
Integration of color Doppler imaging is a unique advantage of echocardiographic-fluoroscopic fusion.
- •
Registration error in the direction of the fluoroscopic beam is an important limitation.
- •
Additional work is needed to assess this technology’s accuracy and clinical value.
Over the past decade, there has been exponential growth in novel percutaneous structural heart interventions developed to treat many valvular and structural heart conditions through a transcatheter approach. Steady growth in the volume of transcatheter aortic valve replacement, along with the introduction of transcatheter mitral valve repair (MitraClip; Abbott Vascular, Santa Clara, CA), left atrial appendage occlusion (Watchman; Boston Scientific, Natick, MA), percutaneous paravalvular leak closure, and a host of other approved and investigational device-based therapies have necessitated more sophisticated imaging guidance not afforded by fluoroscopy alone.
Traditionally, x-ray fluoroscopy with supplemental echocardiography has been used for image guidance. Fluoroscopy offers a wide field of view and excellent visualization of bony structures, catheters, and devices but affords limited visualization of cardiac structural anatomy and adjacent tissues. In contrast, echocardiography has a relatively small field of view, providing limited visualization of catheters and devices, but offers excellent visualization of soft tissue and provides physiologic information using color flow Doppler. Furthermore, echocardiography and fluoroscopy are typically displayed in different orientations, which hinders rapid image interpretation and further contributes to procedural complexity.
The use of cardiac computed tomographic–fluoroscopic fusion imaging and three-dimensional (3D) rotational angiography have recently been described. Both of these modalities offer detailed 3D anatomic information. However, unlike echocardiographic-fluoroscopic fusion imaging, they do not provide real-time imaging to account for translational motion of the heart due to respiration, changes in patient positioning, or changes related to the cardiac cycle. Several reports describing the development and early experience with real-time echocardiographic-fluoroscopic fusion imaging have been published, but literature outlining practical application of this technology is limited. In this report, we provide a critical review of echocardiographic-fluoroscopic fusion imaging for guidance of structural heart interventions and focus on the technical aspects of image registration, supported imaging modes, potential work flows for several commonly performed procedures, and limitations of the current technology. We believe this is critical to facilitate the safe and efficient incorporation into routine clinical practice and to guide further research into this new technology.
Technical Considerations and Sources of Image Registration Error
The first step in fusion imaging is the process of image registration, which involves reorientation of one image (e.g., the echocardiographic image) to match the orientation of a second image (e.g., the fluoroscopic image). The currently available platform (EchoNavigator; Philips Healthcare, Best, The Netherlands) is a software-based solution that provides automated registration of two-dimensional (2D) and 3D transesophageal echocardiographic (TEE) images with x-ray fluoroscopy. Because no additional hardware is necessary to fuse the echocardiographic and fluoroscopic images, incorporation into contemporary catheterization laboratories is relatively straightforward, although it does require proprietary echocardiographic and fluoroscopic systems.
Although a full technical review is beyond the scope of this article, image fusion using the current system relies on rapid, automated identification of the TEE probe tip during active fluoroscopy. The electronic and acoustic core of the TEE transducer are housed in a plastic shell at the tip of the probe and have a characteristic x-ray signature, referred to as the x-ray projection, which changes predictably with changes in probe position (translational dimension) and angulation (rotational dimension) ( Figure 1 ). The system continuously follows the probe position and angulation using a 2D-3D correlation algorithm to find the best match between the visualized x-ray projection on fluoroscopy and the predicted x-ray projection on the basis of a template high-resolution C-arm computed tomographic reconstruction.

Preclinical validation studies using this technology found median registration error of 2 to 3 mm in the plane of the fluoroscopic image, but previous data suggest that there is potential for more substantial error in the direction of the fluoroscopic beam. Echocardiographic-fluoroscopic fusion images rely only on accuracy in the plane of the fluoroscopic image and as such are not affected by this limitation. However, the system also supports placement of annotation points as fiducial markers, which represent points in 3D space and thus require accuracy in all three dimensions ( Figure 2 ). Registration error in the direction of the fluoroscopic beam can be minimized by using multiple fluoroscopic images obtained from different angles at the time of probe registration. The recommended technique for placement of annotation points is discussed later.

A second source of inaccuracy relates to errors in rotational registration. Any error in rotational registration will be magnified as the area of interest moves from the echocardiographic near field to the far field ( Figure 2 ). As an example, a 2° error in rotational registration will result in an error of 1.7 mm at 5 cm of ultrasound depth compared with 3.5 mm at a depth of 10 cm. An early validation study using a phantom heart model demonstrated a mean registration error of 0.8 mm at 5 cm, which increased to 1.4 cm at 10 cm of depth within the ultrasound volume. This source of error can also be minimized by using multiple fluoroscopic images for registration. Further validation of the accuracy of this system in clinical practice and evaluation of potential mechanisms of inaccuracy are needed, with the knowledge that there is increased risk for inaccuracy with increasing distance from the ultrasound transducer.
Application of Fusion Imaging in the Clinical Setting
Our early clinical experience with echocardiographic-fluoroscopic fusion imaging includes 34 cases and is summarized in Table 1 . We used a Philips iE33 echocardiographic system for the first 22 cases and an EPIQ machine for the remaining 12 cases (Philips Healthcare). This was coupled with a Philips Allura Xper FD20/10 fluoroscopic system (Philips Healthcare). The central processing unit running the fusion imaging software was housed in the catheterization laboratory control room and operated remotely from the procedure room with a wireless mouse. Procedural staff members generally included an interventional cardiologist, a structural heart disease interventional fellow, a structural echocardiographer, an advanced echocardiography fellow, and typical procedural support staff members. In our clinical practice, the fusion imaging system and 3D echocardiographic data sets were controlled primarily by the structural echocardiographer or the advanced echocardiography fellow. This is in contrast to some centers, at which the fusion imaging is controlled by the interventional cardiologist.
Procedure Type | Number of cases |
---|---|
Transcatheter mitral valve repair | 13 |
Paravalvular leak closure | 10 |
Mitral paravalvular leak | 6 |
Aortic paravalvular leak | 4 |
Left atrial appendage occlusion | 10 |
Mitral valve-in-valve | 1 |
Fusion Images
Echocardiographic-fluoroscopic fusion imaging is compatible with 2D echocardiographic imaging with or without color Doppler, simultaneous multiplane, and 3D echocardiographic imaging modes. The fused images are automatically displayed from the perspective of the frontal fluoroscopic C arm. The current system does not support fusion with the lateral C arm when using biplane fluoroscopy.
The system offers several options to display and edit echocardiographic images. Two-dimensional and 3D images can be displayed as they are visualized on the imaging machine (“Echo” view) or from the perspective of the fluoroscopic C arm (“C arm” view). Three-dimensional volumes can be displayed in “Free” view, which allows reorientation and cropping in any plane of interest. Three-dimensional volume data sets can also be displayed as the complete volume of data, which can be cropped in the plane of the fluoroscopic image to display soft tissue anatomy relevant to the procedure ( Figure 3 A, Video 1 ; available at www.onlinejase.com ), or as a partial-thickness slice that can be moved from near to far in the direction of the fluoroscopic beam ( Figure 3 B, Video 2 ; available at www.onlinejase.com ). Annotation points (markers), discussed in more detail below, are also displayed on echocardiographic images within the fusion imaging system.

Placement of Annotation Points as Fiducial Markers
The use of annotation points as fiducial markers allows one to identify a point or region of interest in the echocardiographic space and transfer that point to the fluoroscopic space. All imaging modes are supported for the placement of fiducial markers (2D, simultaneous multiplane, and 3D); however, simultaneous multiplane imaging offers rapid and precise localization of regions of interest in 3D space and thus has been the primary modality used in our clinical practice. The transducer should be stationary before placement of annotation points to ensure optimal registration. Although the probe should ideally be “docked” to avoid inadvertent handheld translational motion, we acknowledge that it is frequently not feasible to do this and still maintain adequate esophageal contact.
To create a fiducial marker, one must first register the TEE transducer tip with the fluoroscopic image. This is typically performed with the transducer stationary in the esophagus and with the imaging face of the transducer directed anteriorly. We use three registration angles (45° left anterior oblique, 0°, and 45° right anterior oblique) to mitigate potential registration error in the direction of the fluoroscopic beam. The transducer tip must be fully in the fluoroscopic view at each angle, and the system will highlight the transducer tip in green when successful registration has occurred ( Figure 1 ). One second or less of active fluoroscopy is typically required at each registration angle. Following successful registration, one must locate the region of interest by echocardiography and place an annotation marker in the echocardiographic space. Once placed, a preliminary annotation marker will appear on the fluoroscopic image. If accurate, this marker is then accepted on the fluoroscopic image, which then “transfers” the marker to the fluoroscopic space. This marker will remain fixed in the fluoroscopic space and will remain accurate regardless of TEE probe position, TEE registration status, or horizontal movement of the procedure table. Vertical (up or down) movement of the table should be minimized because vertical tracking of the table may be less accurate and it is also important to remember that a change in patient position on the procedure table at this stage will render the fiducial markers inaccurate.
We have occasionally observed the echocardiographic annotation points to “drift” during the course of routine clinical practice despite the presence of an accurate fluoroscopic annotation point. Figure 4 demonstrates an example related to inaccurate registration that can occur when the TEE probe is unregistered for an extended period after the initial registration (e.g., following probe movement out of and back into the fluoroscopic view). This causes “resetting” of the TEE probe registration, resulting in the loss of the multiple initial registration points and potential registration error in the direction of the fluoroscopic beam. In this case the fluoroscopic marker remains accurate despite the observed drift in the echocardiographic marker. If an accurate echocardiographic marker is required at this stage, it is recommended that the marker be deleted, and a new marker and registration should be performed. A second potential source of “drift” relates to the static nature of annotation points, which do not respond to device-tissue interaction (e.g., deformation) or translational motion due to respiration or patient positioning; in this case, the annotation marker remains accurate in three dimensional space, but the anatomy changes position during the procedure. Last, small misalignment of 2D echocardiographic images with respect to the anatomy may not be appreciated during the procedure and may appear as “drift” on the echocardiographic image when the annotation marker is out of the echocardiographic imaging plane.

Procedure-Specific Considerations
Transseptal Puncture
Transseptal puncture is a critical step for many structural heart procedures, which often require a precise puncture site location. The addition of a fiducial marker on the live fluoroscopic image facilitates positioning of the transseptal needle in the region of interest, permitting fine adjustments using biplane or 3D echocardiography ( Figure 5 , Videos 3 and 4 ; available at www.onlinejase.com ). Annotation points also assist with procedural planning to guide coaxial alignment of devices with anatomy such as the left atrial appendage during left atrial appendage occlusion and the left superior pulmonary vein for guidewire passage after initial transseptal access has been obtained.
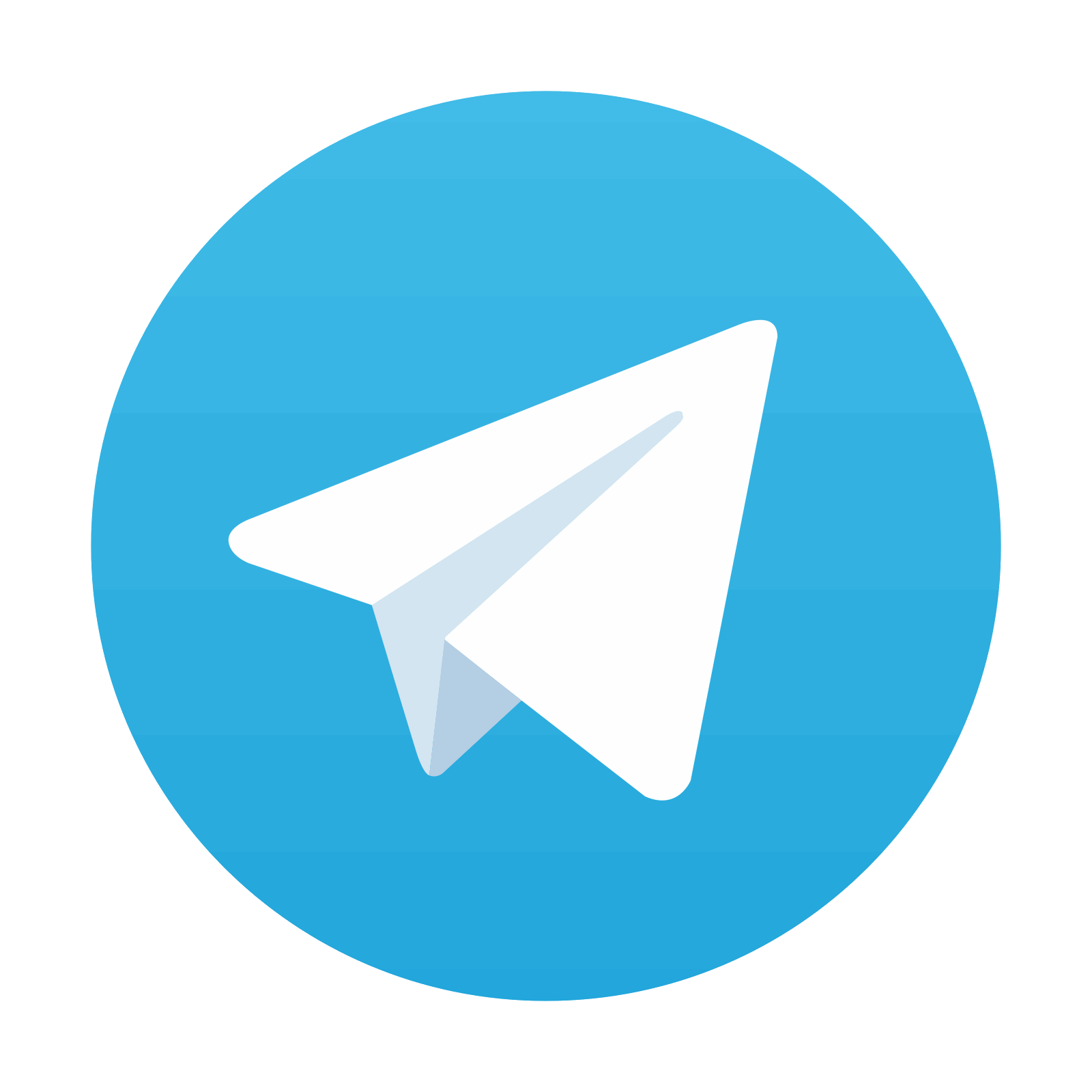
Stay updated, free articles. Join our Telegram channel

Full access? Get Clinical Tree
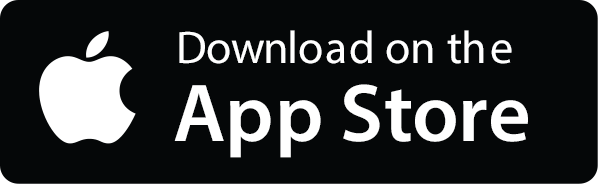
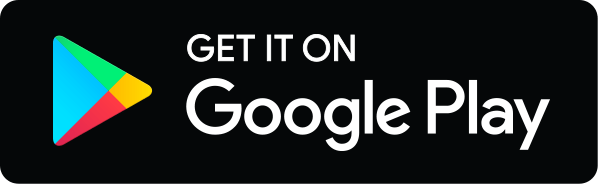
