The introduction of electrocardiographic (ECG)-gated myocardial single-photon emission computed tomography (SPECT) in the 1990s expanded the application of myocardial perfusion imaging to routinely include the assessment of LV systolic function.1 The development and use of this technique occurred when technetium-based imaging agents were placed into clinical use as these tracers provided much higher counts, and therefore image quality for measuring function. This was a critical development in the evolution of myocardial perfusion imaging. Currently, the majority of myocardial perfusion studies performed in the United States use gated SPECT technology and Tc-99m tracers. A more recent development has been the evaluation of ventricular dyssynchrony by SPECT, providing further data beyond myocardial perfusion. This chapter will discuss both aspects of ventricular assessment.
Gated SPECT images can be acquired using single- or multiple-detector cameras. More recently, dual-headed cameras in the 90-degree configuration have been preferred, as images can be acquired in half the time required using a single-headed system without sacrificing image quality. The majority of gated SPECT imaging is performed with high-resolution parallel hole collimators for Tc-99m studies, while all-purpose collimators are used for thallium-201 (Tl-201) studies. A 180-degree imaging arc (45-degree right anterior oblique to 45-degree left posterior oblique projections) with a circular orbit is most commonly used, although noncircular (body contour) orbits can also be used. The most common detector rotation mode is the “continuous step and shoot” acquisition method, in which the detector records events when stationary at each projection, and then rotates (moves) to the next projection. A “continuous” acquisition mode is also available. The standard image matrix size for gated and nongated SPECT imaging is 64 × 64 pixels, with pixel sizes of 5 to 7 mm. This size offers adequate image resolution for interpretation and quantitation of both Tl-201 and Tc-99m tomograms. Contemporary computers possess adequate processing speed and internal hard disk space to process and store large amounts of scintigraphic data. Acquisition computers are usually separate from processing computers to allow for efficient laboratory operations. In addition, unsophisticated, relatively inexpensive, three-lead gating devices are provided by manufacturers to supply the trigger to the acquisition computer.2
In an ECG-gated acquisition, a three-lead ECG provides the R-wave trigger to the acquisition computer, with two successive R-wave peaks on the ECG defining a cardiac cycle. Counts from each phase of the cardiac cycle are binned to a corresponding temporal “frame” within the computer. Perfusion projection images are obtained from summation of the individual frames (Fig. 11-1).1 There is a trade-off between the temporal resolution of gated Tc-99m-sestamibi images and the count density of the individual frames. Gating of myocardial perfusion is usually performed at 8 or 16 frames per R–R interval per projection to maintain the count density using a single-headed camera, although 32 frames per cycle are also possible. With dual-headed cameras, 16 frames per cycle (rather than 8 frames) are preferred as the ejection fraction (EF) results are more in line with other imaging modalities. With a multiheaded SPECT system, more frames can be acquired with no increase in acquisition time, as these systems can obtain higher count density images.
Figure 11-1
Principle of ECG-gated SPECT acquisition. Separate temporal frames corresponding to different phases of the cardiac cycle are acquired for each angular projection. Perfusion images are obtained from summation of the individual frames. (Reproduced with permission from Cullom SJ, Case JA, Bateman TM: Electrocardiographically gated myocardial perfusion SPECT: Technical principles and quality control considerations. J Nucl Cardiol. 1998;5(4):418–425.)

Most manufacturers provide one of two modes of gated SPECT acquisition—”fixed” or “variable”—to define the R–R interval. In fixed acquisition mode, the R–R interval is estimated by the acquisition computer prior to the study, based on previously observed 10 to 20 heartbeats, and remains fixed throughout the study. In the variable acquisition mode, the heart rate is continuously monitored throughout the study and the acquisition computer alters the duration of temporal frames as needed to bin counts equally into the prespecified intervals (8 or 16) per each previously detected R–R interval. In both of these modes “fixed” and “variable,” the data cannot be reformatted after acquisition is complete. An alternative to this is the list mode, a technique increasingly used in contemporary radionuclide studies.3 This technique allows counts to be reformatted into temporal frames after acquisition is complete. The computer records the spatial coordinates of each detected count as well as the timing marker that identifies at what time the count was detected. After acquisition, gated SPECT frames are generated by selecting the mean R–R interval and the beat rejection criteria followed by appropriate binning of the data. The advantage of the list mode is its flexibility, and thus potentially avoids the gating artifacts seen in the fixed- and the variable-mode acquisitions. A disadvantage, however, is that it requires storage of massive amounts of data, and therefore was rarely used in clinical practice until recently, when high-capacity computers became ubiquitous.2
Variations in heart rate due to a variety of factors (sinus arrhythmia, other arrhythmias, patient anxiety or motion, poor ECG lead contact, etc.) can result in temporal “blurring,” that is, mixing of counts from adjacent frames. To limit acquired data to those heartbeats that are representative of the patient’s average heartbeat and to minimize temporal blurring, a beat rejection window is set by specifying the acceptable deviation of R–R interval from the expected value. A 20% (±10) window has historically been applied, although in patients with highly variable heart rates, up to a 100% (±50) acceptance window can be set. Some camera manufacturers provide an extra frame in which counts from all rejected beats are accumulated. The counts within the extra frame can be added to the nongated data after the acquisition is complete in order to generate a summed SPECT dataset for interpretation of the static perfusion images. On most commercial systems, a premature ventricular contraction (PVC) mode may be set that programs the computer to skip one or more cardiac beats before the R–R gating is reestablished. This is done to avoid mixing counts from the two successive cardiac cycles.2
As can be inferred from the above discussion, the detection of an adequate R-wave signal is essential to the successful collection of image data synchronized to heart rate. In patients with severe arrhythmias, the triggering mechanism is incapable of properly identifying the R wave and EF fluctuations, artifactual perfusion abnormalities, and wall thickening discordance may occur.3 Thus, in this situation, a nongated SPECT study may be preferred.
A variety of single- and 2-day protocols may be used in conjunction with gated SPECT. As long as counts are adequate, either Tl-201 or Tc-99m perfusion tracers may be used. Either or both the acquisitions composing the stress/rest or rest/stress protocol can be gated, although the most commonly utilized is the high-dose technetium stress study because of its superior count density. Although the common practice is to gate only the poststress image, a study by Johnson et al.4 reported that in 36% of patients with reversible perfusion defects, the poststress left ventricular ejection fraction (LVEF) was >5% lower than at rest. This implies that global and regional LV functions obtained from poststress–gated acquisitions are not representative of basal LV function in patients with stress-induced ischemia, and that perhaps both rest and stress images should be gated routinely, as long as count density is adequate. It is currently recommended that both rest and stress ECG gating be performed. In general, resting function is of lesser quality due to lower counts and information is not reported unless changes occur between the post stress and rest images.
The ECG-gated SPECT procedure results in the derivation of a time–volume curve, based on the volume of the left ventricle derived by endocardial definition at each of the 8 or 16 bins (frames) within one cardiac cycle. This is in contrast to the time–activity curve derived from radionuclide ventriculography (RVG), a technique that calculates EF from the difference in LV cavity counts between end-systole and end-diastole, rather than from LV volumes determined by endocardial definition. This technique is reviewed in Chapter 22.
To maximize the value of functional ECG-gated SPECT data, a systematic approach to interpretation is essential. Ventricular function should be interpreted only in the context of the perfusion data, as the latter has potential to influence the results and, the two sets of information are complementary. The sequence of interpretation is listed in Table 11-1, and should begin with the evaluation of rotation images followed by perfusion data and finally ventricular function. The final step is integration of clinical information.
|
The interpretation of gated SPECT begins with evaluation of the rotating unprocessed data for overall image quality and any information that might impact function. This includes potential soft tissue attenuation from breast or diaphragm, and interference from extra cardiac liver or gut activity. Inspection of raw data provides assessment of the technical quality of gated SPECT acquisition. In general, images with poor counts should be interpreted with caution as they could be associated with artifacts. Periodic flashing of the display results from gating errors, occurring as a result of wide variation in the cardiac cycle during the acquisition leading to variation in counts between images. Wide variability of R–R interval can also cause a radial blurring artifact in the gated tomographic images. This may limit the definition of end-systolic frame, affecting LVEF and end-systolic volume (ESV) measurements. The presence of gating errors can be further confirmed by graphs displaying accepted counts as a function of the projection number. If there are no gating errors, all projection curves should superimpose nearly perfectly.
Tomographic slices of the myocardium should be displayed according to the standardized model recommended by the American Society of Nuclear Cardiology,5 and interpreted prior to assessment of ventricular function. Using this model, the myocardium is divided into 17 segments based on three short-axis slices (the apical, mid-ventricular, and basal) and a mid-ventricular vertical long-axis slice. The presence of a defect and the degree of reversibility (differences between stress and rest) should also be noted. Quantitative confirmation of the visual observations of the perfusion data should generally be made. Fixed and reversible perfusion deficits should be carefully evaluated for regional function as described in the next section.
Assessment of wall motion should be performed for both the left and the right ventricle. Assessment should include both global left and right ventricular function, and regional function for the left ventricle. The latter is critically important in the presence of a perfusion abnormality. It is generally recommended that ventricular function be interpreted using at least three short-axis slices and one horizontal and vertical long-axis slice at a magnification sufficient to easily assess regional changes. The short-axis slices selected should be similar to those used for perfusion interpretation to enable meaningful comparison.
The interpreter should first estimate the global LVEF by visual interpretation, and then confirm with the quantitative software. Confirmation of quantitated LVEF should include examining the contours, as gut and liver activity might be mistakenly included. It is important for the reader to know the lower limit of normal for a given software quantitation package, since the absolute number varies by imaging modality as well as among software and hardware vendors. Global function can be categorized as normal (EF >50%), mild (EF 40–50%), moderate (EF 30–40%), or severe (EF <30%).
Parameters of regional ventricular function assessed by gated SPECT include segmental myocardial wall motion and myocardial thickening. Segmental wall motion analysis consists of observing both epicardial and endocardial surfaces. Wall motion in one region should be compared to adjacent regions (i.e., inferior compared with anterior). In general, wall motion is best observed using monochrome display scales (thermal, gray, etc.), while wall thickening is best observed using color scale (e.g., isocontour). Assessment of both wall motion and wall thickening contributes to overall evaluation of ventricular function. A visual semi-quantitative assessment of regional wall motion and thickening can be performed using the same 17-segment model used for perfusion assessment. For assessing wall motion, a six-point (0, normal; 1, mild hypokinesis; 2, moderate hypokinesis; 3, severe hypokinesis; 4, akinesis; and 5, dyskinesis) scoring system is used.5 This includes estimation and quantification of LVEF and quantification of LV volumes. Completely automated algorithms, both count based and geometry based, are available.
Right ventricular (RV) size and global functions should also be noted, and important clinical data points. Reports should reflect the best estimation of RV size and function.
While 8- and 16-bin gating are routinely performed for data acquisition, ASNC guidelines recommend the latter. Recent data demonstrate that a larger number of gating bins results in higher fidelity of the time–volume curve, a fact that has practical implications. First, a 16-bin study routinely results in a higher EF value (by approximately 4–5 EF units) compared to 8-bin gating,6 which is more in line with other modalities. In our institution the EF threshold for normality is 45% and 50%, for 8-bin and 16-bin gating, respectively. Second, it is generally accepted that at least 32-bin gating is required for optimal assessment of LV diastolic function, an approach that is generally impractical during SPECT acquisition due to the long acquisition time required.7
The assessment of LV systolic function by gated SPECT has undergone an extensive validation.8 However, the 50% threshold for normality of EF was derived from RVG.9,10 It is well established that the absolute EF numbers are not interchangeable between modalities, and that the threshold for normality should really be modality specific.11 Nevertheless, the 50% threshold is applied across modalities for convenience, and the intermodality variability in absolute EF has been ignored even in the design of landmark clinical trials which have recruited patients based on an absolute EF value. For example, in the Multicenter Automatic Defibrillator Implantation (MADIT) trials the inclusion criteria of EF <35% could be determined by “angiography, radionuclide scanning, or echocardiography.”12 Furthermore, this intermodality variability is exaggerated in patients with LV systolic dysfunction.13
The clinically important attributes of biological measurements are accuracy and precision (reproducibility and repeatability). In the case of EF measurements, the absence of a truth standard makes accuracy difficult to determine. However, measurements of precision are particularly important for serial assessment, and is a strength of radionuclide-based approaches which are largely automated and rely less on operator input. Reproducibility refers to the serial processing of an acquired dataset by the same or different individual, and reflects the effects of operator input on the technique. Repeatability refers to variability in serial acquisition of the data and its subsequent processing. High reproducibility is therefore an inherent requirement for high repeatability. In the context of LVEF, when serial studies are performed in close temporal proximity (e.g., within minutes of each other), technical variability is measured, whereas studies performed days apart reflect both technical and biological variability.14 It is important to use the same processing software processing protocol for EF determination as there is considerable variability among vendors.
In general, EF determination by gated SPECT requires less operator input than RVG, unless completely automatic border detection options are chosen for the processing of the latter. However, several factors affect EF determination by SPECT. Equipment and processing software have profound influences and should be kept constant for serial imaging.15 Patient position, tracer dose, interval between tracer injection and imaging, image reorientation angle, and the presence of large perfusion defects can affect EF determination, often surreptitiously.16–19 The tracer dose and time to imaging affect the target to background ratio and thus, delineation LV borders and segmentation. Available data suggest that the repeatability coefficients (obtained by Bland Altman plotting) for RVG and gated SPECT for serial LVEF assessment are comparable, and in the range of 6% to 8% (EF units).14,20 LVEF changes on serial imaging beyond this range generally reflect a true difference. It must be noted that the contractile reserve of a normal LV is much higher (50–85%) than that of a dysfunctional ventricle. Thus biological variability is higher in the normal LV.21
As the left ventricle becomes dysfunctional and dilated, count statistics in the LV cavity improve, in contrast to the thinned out LV myocardium. Myocardial count statistics are further compromised by large perfusion defects. Thus, RVG may have some inherent advantages over SPECT myocardial perfusion imaging in the assessment of the dysfunctional left ventricle.
There are numerous clinical applications of the gated functional data (Table 11-2). The additive value of functional data to nongated perfusion data has been consistently shown in prior studies.
|
A useful clinical application of gated SPECT imaging is in adjudicating fixed perfusion defects which could be either scar or soft tissue attenuation. The basic assumption using ECG-gated SPECT imaging for this purpose is that a fixed perfusion abnormality associated with CAD should represent either prior myocardial infarction (MI) or stunned myocardium, both of which are associated with wall motion abnormalities. In contrast, if wall motion in the same area as the fixed perfusion abnormality is normal, this should represent attenuation artifact. Thus, a fixed perfusion abnormality with normal wall motion should be considered normal. This interpretation would therefore reduce the number of “false-positive” studies and improve specificity. Several studies have been published,22,23 demonstrating improved specificity using this assumption (Fig. 11-2).
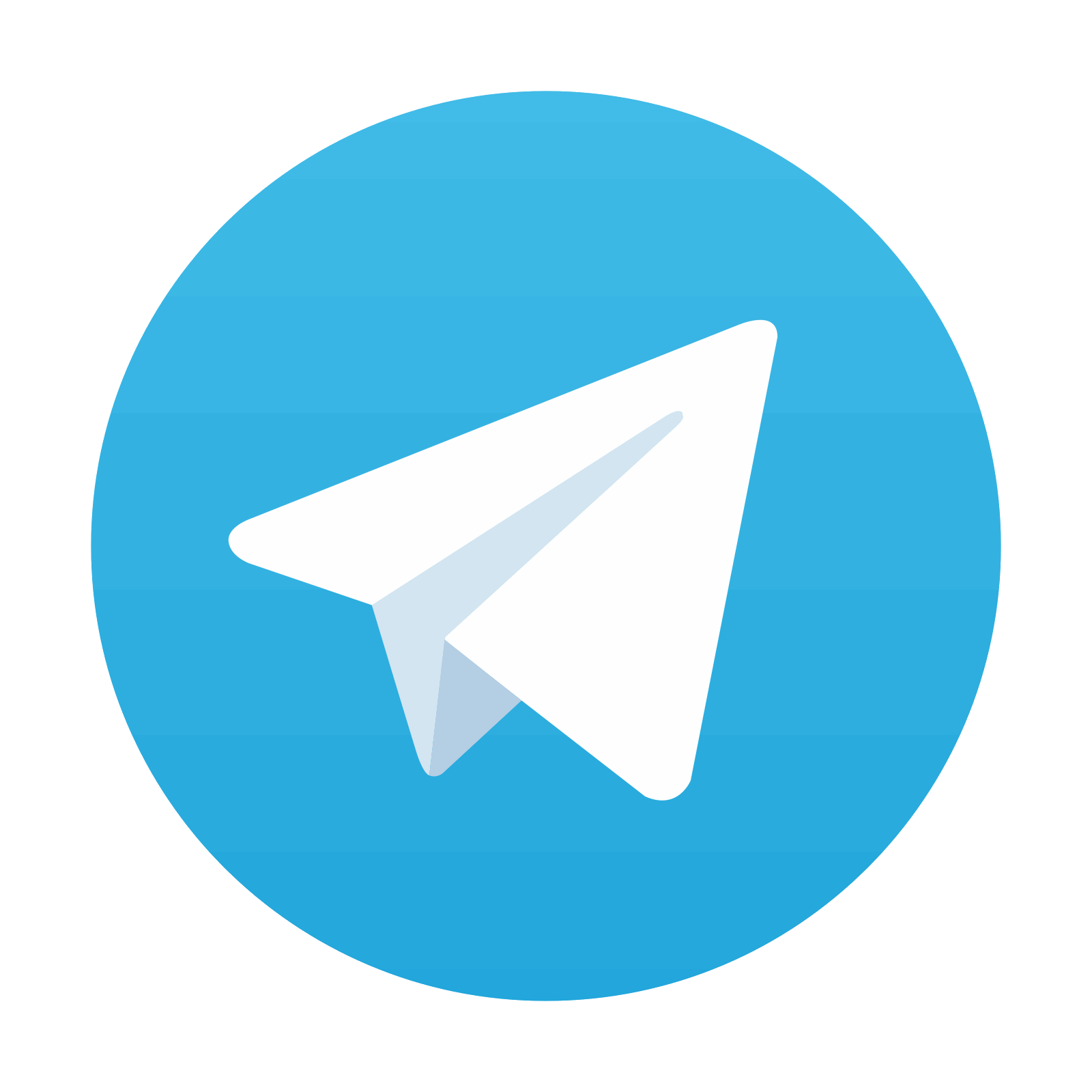
Stay updated, free articles. Join our Telegram channel

Full access? Get Clinical Tree
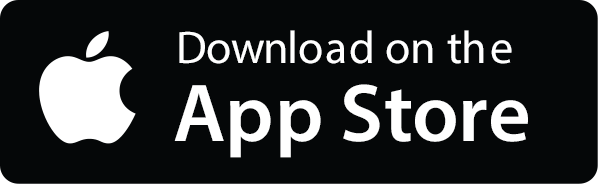
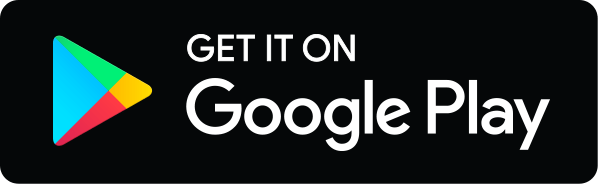